Amphiphobic encapsulation for biodegradable electronics
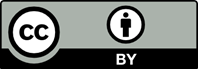
Biodegradable electronics, capable of degradation and resorption in biological environments, require an encapsulation layer for precise lifetime control to perform versatile sensing and actuation in various clinical scenarios. Recent advances in biodegradable polymer chemistry have enabled the development of photocurable encapsulation of biodegradable electronics. However, challenges, such as nonuniform irradiation and incomplete crosslinking due to the limited penetration depth of the light source, restrict their long-term implantable operation. In this study, a 50-μm layer-by-layer three-dimensional (3D) printing approach was adopted for a photocurable encapsulation layer to enhance the lifetime of biodegradable electronics through predictable and homogeneous crosslinking of the encapsulation material. The waterproof and mechanical properties of the 3D-printed polybutanedithiol 1,3,5-triallyl-1,3,5-triazine-2,4,6(1H,3H,5H)-trione pentenoic anhydride (PBTPA) polymer are analyzed and compared with at-once UV-cured PBTPAs. Our study also investigates the enhanced waterproofing properties of the binary hydrophobic polyanhydride, known for its amphiphobic structure. This structure combines both water-trapping and repulsion mechanisms, supported by a high-density network of hydrogen bonding, that create a barrier against water penetration. The 50-μm layer-by-layer 3D printing approach enables controlled irradiation, thereby improving the lifetime of biodegradable electronics and enhancing their mechanical properties. These advancements broaden the scope of biodegradable electronic applications in various fields.
- Shim JS, Rogers JA, Kang SK. Physically transient electronic materials and devices. Mater Sci Eng R Rep. 2021; 145:100624. doi: 10.1016/j.mser.2021.100624
- Bae H, Lee BH, Lee D, et al. Physically transient memory on a rapidly dissoluble paper for security application. Sci Rep. 2016;6(1):38324. doi: 10.1038/srep38324
- Tran H, Feig VR, Liu K, et al. Stretchable and fully degradable semiconductors for transient electronics. ACS Cent Sci. 2019;5(11):1884-1891. doi: 10.1021/acscentsci.9b00850
- Hu W, Jiang J, Xie D, et al. Transient security transistors self-supported on biodegradable natural-polymer membranes for brain-inspired neuromorphic applications. Nanoscale. 2018;10:14893-14901. doi: 10.1039/c8nr04136a
- Lee G, Choi YS, Yoon HJ, Rogers JA. Advances in physicochemically stimuli-responsive materials for on-demand transient electronic systems. Matter. 2020;3(4):1031-1052. doi: 10.1016/j.matt.2020.08.021
- Kang SK, Yin L, Bettinger C. The emergence of transient electronic devices. MRS Bull. 2020;45(2):87-95. doi: 10.1557/mrs.2020.19
- Jamshidi R, Taghavimehr M, Chen Y, Hashemi N, Montazami R. Transient electronics as sustainable systems: from fundamentals to applications. Adv Sustain Syst. 2022;6(2):2100057. doi: 10.1002/adsu.202100057
- Kim KS, Yoo J, Shim JS, et al. Biodegradable molybdenum/ polybutylene adipate terephthalate conductive paste for flexible and stretchable transient electronics. Adv Mater Technol. 2022;7(2):2001297. doi: 10.1002/admt.202001297
- Kim KS, Maeng WY, Kim S, et al. Isotropic conductive paste for bioresorbable electronics. Mater Today Bio. 2023;18:100541. doi: 10.1016/j.mtbio.2023.100541
- Choi Y, Koo J, Rogers JA. Inorganic materials for transient electronics in biomedical applications. MRS Bull. 2020;45(2):103-112. doi: 10.1557/mrs.2020.25
- Li R, Wang L, Kong D, Yin L. Recent progress on biodegradable materials and transient electronics. Bioact Mater. 2018;3(3):322-333. doi: 10.1016/j.bioactmat.2017.12.001
- Fanelli A, Ghezzi D. Transient electronics: new opportunities for implantable neurotechnology. Curr Opin Biotechnol. 2021;72:22-28. doi: 10.1016/j.copbio.2021.08.011
- Lee J, Cho HR, Cha GD, et al. Flexible, sticky, and biodegradable wireless device for drug delivery to brain tumors. Nat Commun. 2019;10(1):5205. doi: 10.1038/s41467-019-13198-y
- Benhabbour SR, Kovarova M, Jones C, et al. Ultra-long-acting tunable biodegradable and removable controlled release implants for drug delivery. Nat Commun. 2019;10(1):4324. doi: 10.1038/s41467-019-12141-5
- Koo J, Kim SB, Choi YS, et al. Wirelessly controlled, bioresorbable drug delivery device with active valves that exploit electrochemically triggered crevice corrosion. Sci Adv. 2020;6(35):eabb1093. doi: 10.1126/sciadv.abb1093
- Pal RK, Farghaly AA, Wang C, Collinson MM, Kundu SC, Yadavalli VK. Conducting polymer-silk biocomposites for flexible and biodegradable electrochemical sensors. Biosens Bioelectron. 2016;81:294-302. doi: 10.1016/j.bios.2016.03.010
- Li R, Qi H, Ma Y, et al. A flexible and physically transient electrochemical sensor for real-time wireless nitric oxide monitoring. Nat Commun. 2020;11(1):3207. doi: 10.1038/s41467-020-17008-8
- Kim HS, Yang SM, Jang TM, Oh N, Kim HS, Hwang SW. Bioresorbable silicon nanomembranes and iron catalyst nanoparticles for flexible, transient electrochemical dopamine monitors. Adv Healthc Mater. 2018;7(24):e1801071. doi: 10.1002/adhm.201801071
- Lu D, Yan Y, Deng Y, et al. Bioresorbable wireless sensors as temporary implants for in vivo measurements of pressure. Adv Funct Mater. 2020;30(40):2003754. doi: 10.1002/adfm.202003754
- Shin J, Yan Y, Bai W, et al. Bioresorbable pressure sensors protected with thermally grown silicon dioxide for the monitoring of chronic diseases and healing processes. Nat Biomed Eng. 2019;3(1):37-46. doi: 10.1038/s41551-018-0300-4
- Shin J, Liu Z, Bai W, et al. Bioresorbable optical sensor systems for monitoring of intracranial pressure and temperature. Sci Adv. 2019;5(7):eaaw1899. doi: 10.1126/sciadv.aaw1899
- Lee G, Ray E, Yoon HJ, et al. A bioresorbable peripheral nerve stimulator for electronic pain block. Sci Adv. 2022;8(40):eabp9169. doi: 10.1126/sciadv.abp9169
- Koo J, MacEwan MR, Kang SK, et al. Wireless bioresorbable electronic system enables sustained nonpharmacological neuroregenerative therapy. Nat Med. 2018;24(12): 1830-1836. doi: 10.1038/s41591-018-0196-2
- Choi YS, Hsueh YY, Koo J, et al. Stretchable, dynamic covalent polymers for soft, long-lived bioresorbable electronic stimulators designed to facilitate neuromuscular regeneration. Nat Commun. 2020;11(1):5990. doi: 10.1038/s41467-020-19660-6
- Maeng WY, Tseng WL, Li S, Koo J, Hsueh YY. Electroceuticals for peripheral nerve regeneration. Biofabrication. 2022;14(4):042002. doi: 10.1088/1758-5090/ac8baa
- Kim G, Hong M, Lee Y, Koo J. Biodegradable materials and devices for neuroelectronics. MRS Bull. 2023;48(5): 518-530. doi: 10.1557/s43577-023-00529-0
- Choi YS, Yin RT, Pfenniger A, et al. Fully implantable and bioresorbable cardiac pacemakers without leads or batteries. Nat Biotechnol. 2021;39(10):1228-1238. doi: 10.1038/s41587-021-00948-x
- Park CW, Kang SK, Hernandez HL, et al. Thermally triggered degradation of transient electronic devices. Adv Mater. 2015;27(25):3783-3788. doi: 10.1002/adma.201501180
- Gao Y, Zhang Y, Wang X, et al. Moisture-triggered physically transient electronics. Sci Adv. 2017;3(9):e1701222. doi: 10.1126/sciadv.1701222
- Choi YS, Koo J, Lee YJ, et al. Biodegradable polyanhydrides as encapsulation layers for transient electronics. Adv Funct Mater. 2020;30(31):2000941. doi: 10.1002/adfm.202000941
- Kim SY, Han G, Hwang DB, et al. Design and usability evaluations of a 3D-printed implantable drug delivery device for acute liver failure in preclinical settings. Adv Healthc Mater. 2021;10(14):e2100497. doi: 10.1002/adhm.202100497
- Won SM, Koo J, Crawford KE, et al. Natural wax for transient electronics. Adv Funct Mater. 2018;28(32):1801819. doi: 10.1002/adfm.201801819
- Kang SK, Hwang SW, Cheng H, et al. Dissolution behaviors and applications of silicon oxides and nitrides in transient electronics. Adv Funct Mater. 2014;24(28):4427-4434. doi: 10.1002/adfm.201304293
- Han G, Lee H, Kang JM, et al. 3D-printed NIR-responsive bullets as multifunctional nanodrug platforms for image-guided local chemo-photothermal therapy. Chem Eng J. 2023;477:147083. doi: 10.1016/j.cej.2023.147083
- Jang TS, Park SJ, Lee JE, et al. Topography‐supported nanoarchitectonics of hybrid scaffold for systematically modulated bone regeneration and remodeling. Adv Funct Mater. 2022;32(51):2206863. doi: 10.1002/adfm.202206863
- Quan H, Zhang T, Xu H, Luo S, Nie J, Zhu X. Photo-curing 3D printing technique and its challenges. Bioact Mater. 2020;5(1):110-115. doi: 10.1016/j.bioactmat.2019.12.003
- Wu J, Fei F, Wei C, et al. Efficient multi-barrier thin film encapsulation of OLED using alternating Al2O3 and polymer layers. RSC Adv. 2018;8(11):5721-5727. doi: 10.1039/c8ra00023a
- Zhao W, Zhou J, Hu H, Xu C, Xu Q. The role of crosslinking density in surface stress and surface energy of soft solids. Soft Matter. 2022;18(3):507-513. doi: 10.1039/d1sm01600h
- Cheng H, Vepachedu V. Recent development of transient electronics. Theor Appl Mech Lett. 2016;6(1):21-31. doi: 10.1016/j.taml.2015.11.012
- Yin L, Cheng H, Mao S, et al. Dissolvable metals for transient electronics. Adv Funct Mater. 2014;24(5):645-658. doi: 10.1002/adfm.201301847
- Fallegger F, Schiavone G, Lacour SP. Conformable hybrid systems for implantable bioelectronic interfaces. Adv Mater. 2020;32(15):e1903904. doi: 10.1002/adma.201903904
- Enzmann DR, Pelc NJ. Brain motion: measurement with phase-contrast MR imaging. Radiology. 1992;185(3):653-660. doi: 10.1148/radiology.185.3.1438741
- Carlsson M, Cain P, Holmqvist C, Stahlberg F, Lundback S, Arheden H. Total heart volume variation throughout the cardiac cycle in humans. Am J Physiol Heart Circ Physiol. 2004;287(1):H243-50. doi: 10.1152/ajpheart.01125.2003
- Banerjee S, Pal A, Fox M. Volume and position change of the stomach during gastric accommodation and emptying: a detailed three-dimensional morphological analysis based on MRI. Neurogastroenterol Motil. 2020;32(8):e13865. doi: 10.1111/nmo.13865
- Harrison DE, Cailliet R, Harrison DD, Troyanovich SJ, Harrison SO. A review of biomechanics of the central nervous system--part I: spinal canal deformations resulting from changes in posture. J Manipulative Physiol Ther. 1999;22(4):227-234. doi: 10.1016/s0161-4754(99)70049-7
- Handorf AM, Zhou Y, Halanski MA, Li WJ. Tissue stiffness dictates development, homeostasis, and disease progression. Organogenesis. 2015;11(1):1-15. doi: 10.1080/15476278.2015.1019687
- Liu J, Zheng H, Poh PSP, Machens HG, Schilling AF. Hydrogels for engineering of perfusable vascular networks. Int J Mol Sci. 2015;16(7):15997-16016. doi: 10.3390/ijms160715997
- Lu KW, Chen HL, Chen HP, Kuo CC. Design of organic/ inorganic multilayer water vapor barrier thin films deposited via plasma polymerization for encapsulation. Thin Solid Films. 2023;767:139672. doi: 10.1016/j.tsf.2023.139672
- Grover R, Srivastava R, Kamalasanan MN, Mehta DS. Multilayer thin film encapsulation for organic light emitting diodes. RSC Adv. 2014;4(21):10808-10814. doi: 10.1039/c3ra46077k
- Xu F, Song Y, Wei M, Wang Y. Water flow through interlayer channels of two-dimensional materials with various hydrophilicities. J Phys Chem C. 2018;122(27): 15772-15779. doi: 10.1021/acs.jpcc.8b04719