3D-printed variable stiffness tissue scaffolds for potential meniscus repair
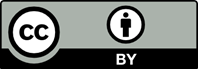
The treatment of meniscus injuries has recently shifted toward the field of tissue engineering (TE). In this work, bovine menisci were characterized, and the regionally-dependent mechanical properties were analyzed. Three-dimensional (3D) printing technology was employed to produce a scaffold that mimicked the mechanical properties of the meniscus. A polycaprolactone (PCL) meniscus scaffold was 3D printed, allowing for the deposition of fibers mimicking the internal architecture of the native meniscus, while achieving regional and variable mechanical stiffness, varying from 2.74 to 0.88 MPa. The PCL scaffold was infiltrated with extracellular matrix (ECM)-like hydrogels composed of gelatin methacrylate (GelMA) and glycosaminoglycans (GAGs), such as hyaluronic acid (HA) and chondroitin sulfate (CS), and subsequently freeze-dried. Human mesenchymal stem cells were seeded onto the scaffolds, and the infiltrated cells were observed to produce ECM components of the native meniscus. Collagen and GAGs production was successfully established. The synthesis of a new matrix reportedly enhances the mechanical properties of the hydrogel over time. Additionally, the circumferential PCL fibers within the scaffold guided the newly synthesized matrix, facilitating replication of the native tissue structure. These results indicate that the ECM-infiltrated 3D-printed PCL scaffold is a promising solution for meniscus repair.

- Zhang K, Li L, Yang L, et al. The biomechanical changes of load distribution with longitudinal tears of meniscal horns on knee joint: a finite element analysis. J Orthop Surg Res. 2019;14(1): 237. doi: 10.1186/s13018-019-1255-1
- Scotti C, Hirschmann MT, Antinolfi P, Martin I, Peretti GM. Meniscus repair and regeneration: review on current methods and research potential. Eur Cell Mater. 2013;26:150-170. doi: 10.22203/ecm.v026a11
- Murphy CA, Costa JB, Silva-Correia J, Oliveira JM, Reis RL, Collins MN. Biopolymers and polymers in the search of alternative treatments for meniscal regeneration: state of the art and future trends. Appl Mater Today. 2018;12:51-71. doi: 10.1016/j.apmt.2018.04.002
- Szojka A, Lalh K, Andrews SHJ, Jomha NM, Osswald M, Adesida AB. Biomimetic 3D printed scaffolds for meniscus tissue engineering. Bioprinting. 2017;8:1-7. doi: 10.1016/j.bprint.2017.08.001
- Leslie BW, Gardner DL, McGeough JA, Moran RS, Anisotropic response of the human knee joint meniscus to unconfined compression. J Eng Med. 2000;214(6):631-635. doi: 10.1243/0954411001535651
- Zhang ZZ, Wang SJ, Zhang JY, et al. 3D-printed poly(ε- caprolactone) scaffold augmented with mesenchymal stem cells for total meniscal substitution: a 12- and 24- week animal study in a rabbit model. Am J Sports Med. 2017;45(7):1497-1511. doi: 10.1177/0363546517691513
- Cengiz IF, Pitikakis M, Cesario L, et al. Building the basis for patient-specific meniscal scaffolds: from human knee MRI to fabrication of 3D printed scaffolds. Bioprinting. 2016;1(June):1-10. doi: 10.1016/j.bprint.2016.05.001
- Fisher MB, Henning EA, Söegaard N, Esterhai JL, Mauck RL. Organized nanofibrous scaffolds that mimic the macroscopic and microscopic architecture of the knee meniscus. Acta Biomater. 2013;9(1):4496-4504. doi: 10.1016/j.actbio.2012.10.018
- Tienen TG, Heijkants RG, de Groot JH, et al. Replacement of the knee meniscus by a porous polymer implant: a study in dogs. Am J Sports Med. 2006;34(1):64-71. doi: 10.1177/0363546505280905
- Vrancken ACT, Eggermont F, Hannink G, Van Tienen TG. Functional biomechanical performance of a novel anatomically shaped polycarbonate urethane total meniscus replacement. Knee Surg Sports Traumatol Arthrosc. 2016;24(5):1485-1494. doi: 10.1007/s00167-015-3632-6
- Cook JL, Fox DB. A novel bioabsorbable conduit augments healing of avascular meniscal tears in a dog model. Am J Sports Med. 2007;35(11):1877-1887. doi: 10.1177/0363546507304330
- Baker BM, Nathan AS, Gee AO, Mauck RL. The influence of an aligned nanofibrous topography on human mesenchymal stem cell fibrochondrogenesis. Biomaterials. 2010;31(24):6190-6200. doi: 10.1016/j.biomaterials.2010.04.036
- Ahn J-H, Kim J, Han G, et al. 3D-printed biodegradable composite scaffolds with significantly enhanced mechanical properties via the combination of binder jetting and capillary rise infiltration process. Addit Manuf. 2021;41:101988. doi: 10.1016/j.addma.2021.101988
- Liu D, Nie W, Li D, et al. 3D printed PCL/SrHA scaffold for enhanced bone regeneration. Chem Eng J. 2019;362: 269-279. doi: 10.1016/j.cej.2019.01.015
- Collins MN, Zamboni F, Serafin A, Ren G, Thanusha AV, Culebras M. The role of hyaluronic acid in tissue engineering. In: Oliveira JM, Radhouani H, Reis RL, eds. Polysaccharides of Microbial Origin: Biomedical Applications. Cham: Springer International Publishing; 2022: 1063-1116. doi: 10.1007/978-3-030-42215-8_56
- Serafin A, Culebras M, Collins MN. Synthesis and evaluation of alginate, gelatin, and hyaluronic acid hybrid hydrogels for tissue engineering applications. Int J Biol Macromol. 2023;233:123438. doi: 10.1016/j.ijbiomac.2023.123438
- Sarem M, Moztarzadeh F, Mozafari M. Optimization strategies on the structural modeling of gelatin/chitosan scaffolds to mimic human meniscus tissue. Mater Sci Eng C Mater Biol Appl. 2013;33(8):4777-4785. doi: 10.1016/j.msec.2013.07.036
- Groll J, Burdick JA, Cho DW, et al. A definition of bioinks and their distinction from biomaterial inks. Biofabrication. 2018;11(1):013001. doi: 10.1088/1758-5090/aaec52
- Rey-rico A, Klich A, Cucchiarini M, Madry H. Biomedical-grade, high mannuronic acid content (BioMVM) alginate enhances the proteoglycan production of primary human meniscal fibrochondrocytes in a 3-D microenvironment. Sci Rep. 2016;6:28170. doi: 10.1038/srep28170
- Puetzer JL, Bonassar LJ. High density type i collagen gels for tissue engineering of whole menisci. Acta Biomater. 2013;9(8):7787-7795. doi: 10.1016/j.actbio.2013.05.002
- Sarem M, Moztarzadeh F, Mozafari M. How can genipin assist gelatin/carbohydrate chitosan scaffolds to act as replacements of load-bearing soft tissues? Carbohydr Polym. 2013;93(2):635-643. doi: 10.1016/j.carbpol.2012.11.099
- Grogan SP, Chung PH, Soman P, et al. Digital micromirror device projection printing system for meniscus tissue engineering. Acta Biomater. 2013;9(7):7218-7226. doi: 10.1016/j.actbio.2013.03.020
- Nichol JW, Koshy ST, Bae H, Hwang CM, Yamanlar S, Khademhosseini A. Cell-laden microengineered gelatin methacrylate hydrogels. Biomaterials. 2010;31(21):5536-5544. doi: 10.1016/j.biomaterials.2010.03.064
- Benton JA, DeForest CA, Vivekanandan V, Anseth KS. Photocrosslinking of gelatin macromers to synthesize porous hydrogels that promote valvular interstitial cell function. Tissue Eng Part A. 2009;15(11): 3221-3230. doi: 10.1089/ten.TEA.2008.0545
- Bahcecioglu G, Hasirci N, Bilgen B, Hasirci V. Hydrogels of agarose, and methacrylated gelatin and hyaluronic acid are more supportive for in vitro meniscus regeneration than three dimensional printed polycaprolactone scaffolds. Int J Biol Macromol. 2019;122:1152-1162. doi: 10.1016/j.ijbiomac.2018.09.06
- Habuchi H, Yamagata T, Iwata H, Suzuki S. The occurrence of a wide variety of dermatan sulfate chondroitin sulfate copolymers in fibrous cartilage. J Biol Chem. 1973;248(17):6019-6028. doi: 10.1016/S0021-9258(19)43502-3
- Levett PA, Melchels FPW, Schrobback K, Hutmacher DW, Malda J, Klein TJ. A biomimetic extracellular matrix for cartilage tissue engineering centered on photocurable gelatin, hyaluronic acid and chondroitin sulfate. Acta Biomater. 2014;10(1):214-223. doi: 10.1016/j.actbio.2013.10.005
- Murphy CA, Cunniffe GM, Garg AK, Collins MN. Regional dependency of bovine meniscus biomechanics on the internal structure and glycosaminoglycan content. J Mech Behav Biomed Mater. 2019;94:186-192. doi: 10.1016/j.jmbbm.2019.02.020
- Murphy CM, Haugh MG, O’Brien FJ. The effect of mean pore size on cell attachment, proliferation and migration in collagen-glycosaminoglycan scaffolds for bone tissue engineering. Biomaterials. 2010;31(3):461-466. doi: 10.1016/j.biomaterials.2009.09.063
- Beck EC, Barragan M, Libeer TB, et al. Chondroinduction from naturally derived cartilage matrix: a comparison between devitalized and decellularized cartilage encapsulated in hydrogel pastes. Tissue Eng Part A. 2016;22(7-8): 665-679. doi: 10.1089/ten.TEA.2015.0546
- Costa B, Oliveira JM, Lu R. Biomaterials in meniscus tissue engineering. In: Oliveira JM, Reis RL, eds. Regenerative Strategies for the Treatment of Knee Joint Disabilities. Cham: Springer International Publishing; 2017: 249-270. doi: 10.1007/978-3-319-44785-8_13
- Kweon H, Yoo MK, Park IK, et al. A novel degradable polycaprolactone networks for tissue engineering. Biomaterials. 2003;24(5):801-808. doi: 10.1016/s0142-9612(02)00370-8
- Baker BM, Mauck RL. The effect of nanofiber alignment on the maturation of engineered meniscus constructs. Biomaterials. 2007;28(11):1967-1977. doi: 10.1016/j.biomaterials.2007.01.004
- Visser J, Melchels FPW, Jeon JE, et al. Reinforcement of hydrogels using three-dimensionally printed microfibres. Nat Commun. 2015;6:6933-6933. doi: 10.1038/ncomms7933
- Murphy CM, Haugh MG, O’Brien FJ. The effect of mean pore size on cell attachment, proliferation and migration in collagen-glycosaminoglycan scaffolds for bone tissue engineering. Biomaterials. 2010;31(3):461-466. doi: 10.1016/j.biomaterials.2009.09.063
- Pan Z, Duan P, Liu X, et al. Effect of porosities of bilayered porous scaffolds on spontaneous osteochondral repair in cartilage tissue engineering. Regen Biomater. 2015;2(1):9-20. doi: 10.1093/rb/rbv001
- Karageorgiou V, Kaplan D. Porosity of 3D biomaterial scaffolds and osteogenesis. Biomaterials. 2005;26(27): 5474-5491. doi: 10.1016/j.biomaterials.2005.02.002
- Murphy CA, Garg AK, Silva-Correia J, Reis RL, Oliveira JM, Collins MN. The meniscus in normal and osteoarthritic tissues: facing the structure property challenges and current treatment trends. Ann Rev Biomed Eng. 2019;21:495-521. doi: 10.1146/annurev-bioeng-060418-052547
- Zein I, Hutmacher DW, Tan KC, Teoh SH. Fused deposition modeling of novel scaffold architectures for tissue engineering applications. Biomaterials. 2002;23(4): 1169-1185. doi: 10.1016/s0142-9612(01)00232-0
- Shor L, Güçeri S, Chang R, et al. Precision extruding deposition (PED) fabrication of polycaprolactone (PCL) scaffolds for bone tissue engineering. Biofabrication. 2009;1(1):015003. doi: 10.1088/1758-5082/1/1/015003
- Kim JY, Yoon JJ, Park EK, Kim DS, Kim SY, Cho DW. Cell adhesion and proliferation evaluation of SFF-based biodegradable scaffolds fabricated using a multi-head deposition system. Biofabrication. 2009;1(1):015002. doi: 10.1088/1758-5082/1/1/015002
- Cahill S, Lohfeld S, McHugh PE. Finite element predictions compared to experimental results for the effective modulus of bone tissue engineering scaffolds fabricated by selective laser sintering. J Mater Sci Mater Med. 2009;20(6): 1255-1262. doi: 10.1007/s10856-009-3693-5
- McDermott ID, Sharifi F, Bull AMJ, Gupte CM, Thomas RW, Amis AA. An anatomical study of meniscal allograft sizing. Knee Surg Sports Traumatol Arthrosc. 2004;12(2):130-135. doi: 10.1007/s00167-003-0366-7
- O’Brien FJ, Harley BA, Waller MA, Yannas VI, Gibson LJ, Prendergast PJ. The effect of pore size on permeability and cell attachment in collagen scaffolds for tissue engineering. Technol Health Care. 2007;15(1):3-17. doi: 10.3233/THC-2007-15102
- Zhang Q, Lu H, Kawazoe N, Chen G. Pore size effect of collagen scaffolds on cartilage regeneration. Acta Biomater. 2014;10(5):2005-2013. doi: 10.1016/j.actbio.2013.12.042
- O’Brien FJ, Harley BA, Yannas VI, Gibson LJ. The effect of pore size on cell adhesion in collagen-GAG scaffolds. Biomaterials. 2005;26(4):433-441. doi: 10.1016/j.biomaterials.2004.02.052
- Silva MMCG, Cyster LA, Barry JJA, et al. The effect of anisotropic architecture on cell and tissue infiltration into tissue engineering scaffolds. Biomaterials. 2006;27(35):5909-5917. doi: 10.1016/j.biomaterials.2006.08.010
- Lien SM, Ko LY, Huang TJ, Effect of pore size on ECM secretion and cell growth in gelatin scaffold for articular cartilage tissue engineering. Acta Biomater. 2009;5(2):670-679. doi: 10.1016/j.actbio.2008.09.020
- Haugh MG, Murphy CM, O’Brien FJ. Novel freeze-drying methods to produce a range of collagen–glycosaminoglycan scaffolds with tailored mean pore sizes. Tissue Eng Part C Methods. 2010;16(5):887-894. doi: 10.1089/ten.TEC.2009.0422
- Stenhamre H, Nannmark U, Lindahl A, Gatenholm P, Brittberg M. Influence of pore size on the redifferentiation potential of human articular chondrocytes in poly (urethane urea) scaffolds. J Tissue Eng Regen Med. 2011;5(7):578-588. doi: 10.1002/term.350
- Nava MM, Draghi L, Giordano C, Pietrabissa R. The effect of scaffold pore size in cartilage tissue engineering. J Appl Biomater Funct Mater. 2016;14(3):e223-e229. doi: 10.5301/jabfm.5000302
- Sheehy EJ, Buckley CT, Kelly DJ. Oxygen tension regulates the osteogenic, chondrogenic and endochondral phenotype of bone marrow derived mesenchymal stem cells. Biochem Biophys Res Commun. 2012;417(1):305-310. doi: 10.1016/j.bbrc.2011.11.105
- Baker BM, Nathan AS,Huffman GR, Mauck RL. Tissue engineering with meniscus cells derived from surgical debris. Osteoarthritis Cartilage. 2009;17(3):336-345. doi: 10.1016/j.joca.2008.08.001
- Yoshikawa K, Kitamura N, Kurokawa T, Gong JP, Nohara Y, Yasuda K. Hyaluronic acid affects the in vitro induction effects of synthetic PAMPS and PDMAAm hydrogels on chondrogenic differentiation of ATDC5 cells, depending on the level of concentration. BMC Musculoskelet Disord. 2013;14:56. doi: 10.1186/1471-2474-14-56
- Frean SP, Abraham LA, Lees P, In vitro stimulation of equine articular cartilage proteoglycan synthesis by hyaluronan and carprofen. Res Vet Sci. 1999;67(2):183-190. doi: 10.1053/rvsc.1999.0328
- Bian L, Hou C, Tous E, Rai R, Mauck RL, Burdick JA. The influence of hyaluronic acid hydrogel crosslinking density and macromolecular diffusivity on human MSC chondrogenesis and hypertrophy. Biomaterials. 2013;34(2):413-421. doi: 10.1016/j.biomaterials.2012.09.052
- Re’em T, Kaminer-Israeli Y, Ruvinov E, Cohen S. Chondrogenesis of hMSC in affinity-bound TGF-beta scaffolds. Biomaterials. 2012;33:751. doi: 10.1016/j.biomaterials.2011.10.007
- Roberts JJ, Nicodemus GD, Giunta S, Bryant SJ. Incorporation of biomimetic matrix molecules in PEG hydrogels enhances matrix deposition and reduces load-induced loss of chondrocyte-secreted matrix. J Biomed Mater Res A. 2011;97 A(3):281-291. doi: 10.1002/jbm.a.33057
- Levett PA, Hutmacher DW, Malda J, Klein TJ. Hyaluronic acid enhances the mechanical properties of tissue-engineered cartilage constructs. PLoS ONE. 2014;9(12):e113216. doi: 10.1371/journal.pone.0113216
- Eyre DR, Koob TJ, Chun LE. Biochemistry of the meniscus: unique profile of collagen types and site-dependent variations in composition. Orthop Transact. 1983;8:56.
- Wu JJ, Eyre DR, Slayter HS. Type VI collagen of the intervertebral disc. Biochemical and electron-microscopic characterization of the native protein. Biochem J. 1987;248(2):373-381. doi: 10.1042/bj248037
- Makris EA, Hadidi P, Athanasiou KA. The knee meniscus: structure-function, pathophysiology, current repair techniques, and prospects for regeneration. Biomaterials. 2011;32(30):7411-7431. doi: 10.1016/j.biomaterials.2011.06.037
- Erickson IE, Kestle SR, Zellars KH, et al. High mesenchymal stem cell seeding densities in hyaluronic acid hydrogels produce engineered cartilage with native tissue properties. Acta Biomater. 2012;8(8):3027-3034. doi: 10.1016/j.actbio.2012.04.033
- Klein TJ, Rizzi SC, Schrobback K, et al. Long-term effects of hydrogel properties on human chondrocyte behavior. Soft Matter. 2010;6(20):5175-5183. doi: 10.1039/C0SM00229A