Advancements in 3D bioprinting for nanoparticle evaluation: Techniques, models, and biological applications
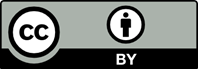
Three-dimensional (3D) bioprinting technology has opened new possibilities for nanoparticle evaluation. This review discusses the latest research trends using various disease models created through 3D bioprinting for the biological evaluation of nanoparticles. The focus is on tumor models, vascular models, and skin models. In tumor models, evaluations include antitumor effects, gene expression analysis, and cytotoxicity comparisons between 2D and 3D models. Vascular models are used to assess restenosis prevention, ischemic repair, and vascular regeneration. Skin models are employed to investigate nanoparticle toxicity, drug release, and transdermal penetration. These studies highlight the versatility of 3D bioprinting in replicating complex biological environments, enabling more accurate nanoparticle testing. The use of various bioinks and cell types enhances the relevance of in vitro findings. The integration of nanoparticles with 3D-bioprinted models shows significant potential in advancing therapeutic strategies, including cancer treatment, vascular repair, and drug delivery systems. Overall, by providing selected examples to illustrate the concepts, this comprehensive review underscores the importance of 3D bioprinting as an innovative platform for nanoparticle research, bridging the gap between traditional 2D cell cultures and in vivo studies, and contributing to the development of nanomedicines and personalized medical treatments.

- Jamieson C, Keenan P, Kirkwood D, et al. A review of recent advances in 3D bioprinting with an eye on future regenerative therapies in veterinary medicine. Front Vet Sci. 2020;7:584193. doi: 10.3389/fvets.2020.584193
- Ricci G, Gibelli F, Sirignano A. Three-dimensional bioprinting of human organs and tissues: bioethical and medico-legal implications examined through a scoping review. Bioengineering (Basel, Switzerland). 2023;10(9):1052. doi: 10.3390/bioengineering10091052
- Yaneva A, Shopova D, Bakova D, et al. The progress in bioprinting and its potential impact on health-related quality of life. Bioengineering (Basel, Switzerland). 2023;10(8):910. doi: 10.3390/bioengineering10080910
- Cheng Y, Hu H, Dong X, Hao X, Li Y. Exploring transformer model in longitudinal pharmacokinetic/pharmacodynamic analyses and comparing with alternative natural language processing models. J Pharm Sci. 2024;113(5):1368-1375. doi: 10.1016/j.xphs.2024.02.008
- Migulina N, de Hilster RHJ, Bartel S, et al. 3-D culture of human lung fibroblasts decreases proliferative and increases extracellular matrix remodeling genes. Am J Physiol Cell Physiol. 2024;326(1):C177-C193. doi: 10.1152/ajpcell.00374.2023
- Nizamoglu M, Alleblas F, Koster T, et al. Three dimensional fibrotic extracellular matrix directs microenvironment fiber remodeling by fibroblasts. Acta Biomater. 2024;177:118-131. doi: 10.1016/j.actbio.2024.02.008
- Bernal PN, Bouwmeester M, Madrid-Wolff J, et al. Volumetric bioprinting of organoids and optically tuned hydrogels to build liver-like metabolic biofactories. Adv Mater. 2022;34(15):2110054. doi: 10.1002/adma.202110054
- Muthusamy S, Kannan S, Lee M, et al. 3D bioprinting and microscale organization of vascularized tissue constructs using collagen-based bioink. Biotechnol Bioeng. 2021;118(8):3150-3163. doi: 10.1002/bit.27838
- Jia W, Gungor-Ozkerim PS, Zhang YS, et al. Direct 3D bioprinting of perfusable vascular constructs using a blend bioink. Biomaterials. 2016;106:58-68. doi: 10.1016/j.biomaterials.2016.07.038
- Zhu J, Wang Y, Zhong L, Pan F, Wang J. Advances in tissue engineering of vasculature through three-dimensional bioprinting. Dev Dyn. 2021;250(12):1717-1738. doi: 10.1002/dvdy.385
- Farouk SM, Khafaga AF, Abdellatif AM. Bladder cancer: therapeutic challenges and role of 3D cell culture systems in the screening of novel cancer therapeutics. Cancer Cell Int. 2023;23(1):251. doi: 10.1186/s12935-023-03069-4
- Jensen C, Teng Y. Is it time to start transitioning from 2D to 3D cell culture? Front Mol Biosci. 2020;7:33. doi: 10.3389/fmolb.2020.00033
- Abuwatfa WH, Pitt WG, Husseini GA. Scaffold-based 3D cell culture models in cancer research. J Biomed Sci. 2024;31(1):7. doi: 10.1186/s12929-024-00994-y
- Centeno EGZ, Cimarosti H, Bithell A. 2D versus 3D human induced pluripotent stem cell-derived cultures for neurodegenerative disease modelling. Mol Neurodegener. 2018;13(1):27. doi: 10.1186/s13024-018-0258-4
- Khafaga AF, Mousa SA, Aleya L, Abdel-Daim MM. Three-dimensional (3D) cell culture: a valuable step in advancing treatments for human hepatocellular carcinoma. Cancer Cell Int. 2022;22(1):243. doi: 10.1186/s12935-022-02662-3
- Badr-Eldin SM, Aldawsari HM, Kotta S, Deb PK, Venugopala KN. Three-dimensional in vitro cell culture models for efficient drug discovery: progress so far and future prospects. Pharmaceuticals (Basel). 2022;15(8):926. doi: 10.3390/ph15080926
- Belfiore L, Aghaei B, Law AMK, et al. Generation and analysis of 3D cell culture models for drug discovery. Eur J Pharm Sci. 2021;163:105876. doi: 10.1016/j.ejps.2021.105876
- Parodi I, Di Lisa D, Pastorino L, Scaglione S, Fato MM. 3D bioprinting as a powerful technique for recreating the tumor microenvironment. Gels. 2023;9(6):482. doi: 10.3390/gels9060482
- Germain N, Dhayer M, Dekiouk S, Marchetti P. Current advances in 3D bioprinting for cancer modeling and personalized medicine. Int J Mol Sci. 2022;23(7):3432. doi: 10.3390/ijms23073432
- Bojin F, Robu A, Bejenariu MI, et al. 3D bioprinting of model tissues that mimic the tumor microenvironment. Micromachines (Basel). 2021;12(5):535. doi: 10.3390/mi12050535
- Di Marzio N, Eglin D, Serra T, Moroni L. Bio-fabrication: convergence of 3D bioprinting and nano-biomaterials in tissue engineering and regenerative medicine. Front Bioeng Biotechnol. 2020;8:326. doi: 10.3389/fbioe.2020.00326
- Richards D, Jia J, Yost M, Markwald R, Mei Y. 3D bioprinting for vascularized tissue fabrication. Ann Biomed Eng. 2017;45(1):132-147. doi: 10.1007/s10439-016-1653-z
- Chen EP, Toksoy Z, Davis BA, Geibel JP. 3D bioprinting of vascularized tissues for in vitro and in vivo applications. Front Bioeng Biotechnol. 2021;9:664188. doi: 10.3389/fbioe.2021.664188
- Yang Q, Gao B, Xu F. Recent advances in 4D bioprinting. Biotechnol J. 2020;15(1):e1900086. doi: 10.1002/biot.201900086
- Phogat S, Thiam F, Al Yazeedi S, Abokor FA, Osei ET. 3D in vitro hydrogel models to study the human lung extracellular matrix and fibroblast function. Respir Res. 2023;24(1):242. doi: 10.1186/s12931-023-02548-6
- Vakhshiteh F, Bagheri Z, Soleimani M, et al. Heterotypic tumor spheroids: a platform for nanomedicine evaluation. J Nanobiotechnology. 2023;21(1):249. doi: 10.1186/s12951-023-02021-y
- Chia SL, Tay CY, Setyawati MI, Leong DT. Biomimicry 3D gastrointestinal spheroid platform for the assessment of toxicity and inflammatory effects of zinc oxide nanoparticles. Small. 2015;11(6):702-712. doi: 10.1002/smll.201401915
- Kim D, Jo S, Lee D, et al. NK cells encapsulated in micro/ macropore-forming hydrogels via 3D bioprinting for tumor immunotherapy. Biomater Res. 2023;27(1):60. doi: 10.1186/s40824-023-00403-9
- Sun S, Wang YH, Gao X, et al. Current perspectives and trends in nanoparticle drug delivery systems in breast cancer: bibliometric analysis and review. Front Bioeng Biotechnol. 2023;11:1253048. doi: 10.3389/fbioe.2023.1253048
- Chakraborty A, Roy A, Ravi SP, Paul A. Exploiting the role of nanoparticles for use in hydrogel-based bioprinting applications: concept, design, and recent advances. Biomater Sci. 2021;9(19):6337-6354. doi: 10.1039/d1bm00605c
- Datta P, Dey M, Ataie Z, Unutmaz D, Ozbolat IT. 3D bioprinting for reconstituting the cancer microenvironment. NPJ Precis Oncol. 2020;4:18. doi: 10.1038/s41698-020-0121-2
- Cho ES, Kang HE, Kim NH, Yook JI. Therapeutic implications of cancer epithelial-mesenchymal transition (EMT). Arch Pharm Res. 2019;42(1):14-24. doi: 10.1007/s12272-018-01108-7
- Chen Y, Xu L, Li W, et al. 3D bioprinted tumor model with extracellular matrix enhanced bioinks for nanoparticle evaluation. Biofabrication. 2022;14(2):025002. doi: 10.1088/1758-5090/ac48e4
- Tomeh MA, Hadianamrei R, Zhao X. A review of curcumin and its derivatives as anticancer agents. Int J Mol Sci. 2019;20(5):1033. doi: 10.3390/ijms20051033
- Gu X, Gao Y, Wang P, et al. Nano-delivery systems focused on tumor microenvironment regulation and biomimetic strategies for treatment of breast cancer metastasis. J Control Release. 2021;333:374-390. doi: 10.1016/j.jconrel.2021.03.039
- Su Y, Hu X, Kang Y, et al. Curcumin nanoparticles combined with 3D printed bionic tumor models for breast cancer treatment. Biofabrication. 2022;15(1):014105. doi: 10.1088/1758-5090/aca5b8
- Chirivì M, Bearzi C, Rosa P, et al. Biomimetic keratin-coated gold nanoparticles for photo-thermal therapy in a 3D bioprinted glioblastoma tumor model. Int J Mol Sci. 2022;23(17):9528. doi: 10.3390/ijms23179528
- Nam KH, Jeong CB, Kim H, et al. Quantitative photothermal characterization with bioprinted 3D complex tissue constructs for early-stage breast cancer therapy using gold nanorods. Adv Healthc Mater. 2021;10(18):e2100636. doi: 10.1002/adhm.202100636
- Dong X, Li R, Xiu P, et al. Meloxicam executes its antitumor effects against hepatocellular carcinoma in COX-2- dependent and -independent pathways. PloS One. 2014;9(3):e92864. doi: 10.1371/journal.pone.0092864
- Rarokar N, Ravikumar C, Gurav S, Khedekar P. Meloxicam encapsulated nanostructured colloidal self-assembly for evaluating antitumor and anti-inflammatory efficacy in 3D printed scaffolds. J Biomed Mater Res A. 2021;109(8):1441- 1456. doi: 10.1002/jbm.a.37135
- Kaur IP, Bhandari R, Bhandari S, Kakkar V. Potential of solid lipid nanoparticles in brain targeting. J Control Release. 2008;127(2):97-109. doi: 10.1016/j.jconrel.2007.12.018
- Rohit B, Pal Kaur I. A method to prepare solid lipid nanoparticles with improved entrapment efficiency of hydrophilic drugs. Current Nanosci. 2013;9(2):211-220. doi: 10.2174/1573413711309020008
- Liu D, Cito S, Zhang Y, Wang CF, Sikanen TM, Santos HA. A versatile and robust microfluidic platform toward high throughput synthesis of homogeneous nanoparticles with tunable properties. Adv Mater. 2015;27(14):2298-2304. doi: 10.1002/adma.201405408
- Arduino I, Liu Z, Rahikkala A, et al. Preparation of cetyl palmitate-based PEGylated solid lipid nanoparticles by microfluidic technique. Acta Biomater. 2021;121:566-578. doi: 10.1016/j.actbio.2020.12.024
- Figueiredo P, Lintinen K, Kiriazis A, et al. In vitro evaluation of biodegradable lignin-based nanoparticles for drug delivery and enhanced antiproliferation effect in cancer cells. Biomaterials. 2017;121:97-108. doi: 10.1016/j.biomaterials.2016.12.034
- Figueiredo P, Sipponen MH, Lintinen K, et al. Preparation and characterization of dentin phosphophoryn-derived peptide-functionalized lignin nanoparticles for enhanced cellular uptake. Small. 2019;15(24):e1901427. doi: 10.1002/smll.201901427
- Ahn M, Cho WW, Park W, et al. 3D biofabrication of diseased human skin models in vitro. Biomater Res. 2023;27(1):80. doi: 10.1186/s40824-023-00415-5
- Cho SW, Malick H, Kim SJ, Grattoni A. Advances in skin-on-a-chip technologies for dermatological disease modeling. J Invest Dermatol. 2024;144(8):1707-1715. doi: 10.1016/j.jid.2024.01.031
- Scheurer J, Sauer B, Focken J, et al. Histological and functional characterization of 3D human skin models mimicking the inflammatory skin diseases psoriasis and atopic dermatitis. Dis Model Mech. 2024;17(1):dmm050541. doi: 10.1242/dmm.050541
- Nuwayhid R, Schulz T, Siemers F, et al. A platform for testing the biocompatibility of implants: silicone induces a proinflammatory response in a 3D skin equivalent. Biomedicines. 2024;12(1):224. doi: 10.3390/biomedicines12010224
- Randall MJ, Jüngel A, Rimann M, Wuertz-Kozak K. Advances in the biofabrication of 3D skin in vitro: healthy and pathological models. Front Bioeng Biotechnol. 2018;6:154. doi: 10.3389/fbioe.2018.00154
- Zoio P, Oliva A. Skin-on-a-chip technology: microengineering physiologically relevant in vitro skin models. Pharmaceutics. 2022;14(3):682. doi: 10.3390/pharmaceutics14030682
- Mukhopadhyay C, Paul MK. Organoid-based 3D in vitro microphysiological systems as alternatives to animal experimentation for preclinical and clinical research. Arch Toxicol. 2023;97(5):1429-1431. doi: 10.1007/s00204-023-03466-8
- Marano F. Les méthodes alternatives à l’expérimentation animale, présent et futur [Alternative methods to animal testing, present and future]. Biol Aujourdhui. 2023;217(3- 4):199-205. doi: 10.1051/jbio/2023035
- Gao C, Lu C, Jian Z, et al. 3D bioprinting for fabricating artificial skin tissue. Colloids Surf B Biointerfaces. 2021;208:112041. doi: 10.1016/j.colsurfb.2021.112041
- Hou X, Liu S, Wang M, et al. Layer-by-layer 3D constructs of fibroblasts in hydrogel for examining transdermal penetration capability of nanoparticles. SLAS Technol. 2017;22(4):447-453. doi: 10.1177/2211068216655753
- do Amaral SR, Amantino CF, Atanasov A, et al. Evaluation of photodynamic therapy as a new therapeutic approach using quinizarin-loaded nanocapsules: in vitro cytotoxicity, permeation in 3D bioprinted skin equivalent and in vitro cytokines modulation. Preprints; 2024. doi: 10.20944/preprints202405.1323.v1
- Bhamare N, Tardalkar K, Khadilkar A, Parulekar P, Joshi MG. Tissue engineering of human ear pinna. Cell Tissue Bank. 2022;23(3):441-457. doi: 10.1007/s10561-022-09991-7
- Bos EJ, Doerga P, Breugem CC, van Zuijlen PP. The burned ear; possibilities and challenges in framework reconstruction and coverage. Burns. 2016;42(7):1387-1395. doi: 10.1016/j.burns.2016.02.006
- Abaci A, Camci-Unal G, Guvendiren M, Guest E. Three-dimensional bioprinting for medical applications. MRS Bulletin. 2023;48(6):624-631. doi: 10.1557/s43577-023-00546-z
- Bernal PN, Delrot P, Loterie D, et al. Volumetric bioprinting of complex living-tissue constructs within seconds. Adv Mater. 2019;31(42):1904209. doi: 10.1002/adma.201904209
- Yalgın A, Köse FA, Gökçe EH. The effect of cyclosporine A and co-enzyme Q10 loaded solid lipid nanoparticles on 3D printed human auricular model: evaluation of cell growth. J Drug Deliv Sci Technol. 2023;79:104087. doi: 10.1016/j.jddst.2022.104087
- Fang Y, Ouyang L, Zhang T, Wang C, Lu B, Sun W. Optimizing bifurcated channels within an anisotropic scaffold for engineering vascularized oriented tissues. Adv Healthc Mater. 2020;9(24):e2000782. doi: 10.1002/adhm.202000782
- Gao Q, Liu Z, Lin Z, et al. 3D bioprinting of vessel-like structures with multilevel fluidic channels. ACS Biomater Sci Eng. 2017;3(3):399-408. doi: 10.1021/acsbiomaterials.6b00643
- Zhou X, Gao Q, Yu D, et al. 3D-bioprinted vascular scaffold with tunable mechanical properties for simulating and promoting neo-vascularization. Smart Mater Med. 2022;3:199-208. doi: 10.1016/j.smaim.2022.01.003
- Gold KA, Saha B, Rajeeva Pandian NK, et al. 3D bioprinted multicellular vascular models. Adv Healthc Mater. 2021;10(21):2101141. doi: 10.1002/adhm.202101141
- Boada C, Zinger A, Tsao C, et al. Rapamycin-loaded biomimetic nanoparticles reverse vascular inflammation. Circ Res. 2020;126(1):25-37. doi: 10.1161/CIRCRESAHA.119.315185
- Betala J, Bae S, Langan III EM, LaBerge M, Lee JS. Combinatorial therapy of sirolimus and heparin by nanocarrier inhibits restenosis after balloon angioplasty ex vivo. Nanomedicine. 2020;15(12):1205-1220. doi: 10.2217/nnm-2020-0028
- Rosner D, McCarthy N, Bennett M. Rapamycin inhibits human in stent restenosis vascular smooth muscle cells independently of pRB phosphorylation and p53. Cardiovasc Res. 2005;66(3):601-610. doi: 10.1016/j.cardiores.2005.01.006
- Hauser PV, Chang H-M, Nishikawa M, Kimura H, Yanagawa N, Hamon M. Bioprinting scaffolds for vascular tissues and tissue vascularization. Bioengineering. 2021;8(11):178. doi: 10.3390/bioengineering8110178
- Choi J, Lee EJ, Lim HJ, et al. Development of 3D-bioprinted artificial blood vessels loaded with rapamycin-nanoparticles for ischemic repair. Int J Bioprin. 2024;10(2):1465. doi: 10.36922/ijb.1465
- Ge G, Wu H, Xiong F, et al. The cytotoxicity evaluation of magnetic iron oxide nanoparticles on human aortic endothelial cells. Nanoscale Res Lett. 2013;8:1-10. doi: 10.1186/1556-276X-8-215
- Wu X, Tan Y, Mao H, Zhang M. Toxic effects of iron oxide nanoparticles on human umbilical vein endothelial cells. Int J Nanomedicine. 2010;5:385-399. doi: 10.2147/ijn.s10458
- Nemmar A, Beegam S, Yuvaraju P, et al. Ultrasmall superparamagnetic iron oxide nanoparticles acutely promote thrombosis and cardiac oxidative stress and DNA damage in mice. Part Fibre Toxicol. 2015;13:1-11. doi: 10.1186/s12989-016-0132-x
- Ning L, Zanella S, Tomov ML, et al. Targeted rapamycin delivery via magnetic nanoparticles to address stenosis in a 3D bioprinted in vitro model of pulmonary veins. Adv Sci (Weinh). 2024;11(26):e2400476. doi: 10.1002/advs.202400476
- Lee EJ, Choi J, Lim HJ, et al. 3D-bioprinted cell-laden blood vessel with dual drug delivery nanoparticles for advancing vascular regeneration. Int J Bioprint. 2024;10(2):1857. doi: 10.36922/ijb.1857
- Song R, Fullerton DA, Ao L, et al. Altered micro RNA expression is responsible for the pro‐osteogenic phenotype of interstitial cells in calcified human aortic valves. J Am Heart Assoc. 2017;6(4):e005364. doi: 10.1161/JAHA.116.005364
- Voicu G, Mocanu CA, Safciuc F, et al. Nanocarriers of shRNA-Runx2 directed to collagen IV as a nanotherapeutic system to target calcific aortic valve disease. Mater Today Bio. 2023;20:100620. doi: 10.1016/j.mtbio.2023.100620
- van der Ven CFT, Tibbitt MW, Conde J, et al. Controlled delivery of gold nanoparticle-coupled miRNA therapeutics via an injectable self-healing hydrogel. Nanoscale. 2021;13(48):20451-20461. doi: 10.1039/d1nr04973a
- Ning L, Gil CJ, Hwang B, et al. Biomechanical factors in three-dimensional tissue bioprinting. Appl Phys Rev. 2020;7(4):041319. doi: 10.1063/5.0023206
- Byambaa B, Annabi N, Yue K, et al. Bioprinted osteogenic and vasculogenic patterns for engineering 3D bone tissue. Adv Healthc Mater. 2017;6(16):1700015. doi: 10.1002/adhm.201700015
- Gonzalez-Fernandez T, Tenorio AJ, Campbell KT, Silva EA, Leach JK. Alginate-based bioinks for 3D bioprinting and fabrication of anatomically accurate bone grafts. Tissue Eng Part A. 2021;27(17–18):1168-1181. doi: 10.1089/ten.TEA.2020.0305
- Fischetti T, Di Pompo G, Baldini N, Avnet S, Graziani G. 3D printing and bioprinting to model bone cancer: the role of materials and nanoscale cues in directing cell behavior. Cancers. 2021;13(16):4065. doi: 10.3390/cancers13164065
- Holmes B, Bulusu K, Plesniak M, Zhang LG. A synergistic approach to the design, fabrication and evaluation of 3D printed micro and nano featured scaffolds for vascularized bone tissue repair. Nanotechnology. 2016;27(6): 064001. doi: 10.1088/0957-4484/27/6/064001
- Baino F, Minguella-Canela J, Korkusuz F, et al. In vitro assessment of bioactive glass coatings on alumina/ zirconia composite implants for potential use in prosthetic applications. Int J Mol Sci. 2019;20(3):722. doi: 10.3390/ijms20030722
- Chen YW, Shen YF, Ho CC, et al. Osteogenic and angiogenic potentials of the cell-laden hydrogel/mussel-inspired calcium silicate complex hierarchical porous scaffold fabricated by 3D bioprinting. Mate Sci Eng C, Mater Biol Appl. 2018;91:679-687. doi: 10.1016/j.msec.2018.06.005
- Yang Z, Yi P, Liu Z, et al. Stem cell-laden hydrogel-based 3D bioprinting for bone and cartilage tissue engineering. Front Bioeng Biotechnol. 2022;10:865770. doi: 10.3389/fbioe.2022.865770
- Zhang J, Eyisoylu H, Qin X-H, Rubert M, Müller R. 3D bioprinting of graphene oxide-incorporated cell-laden bone mimicking scaffolds for promoting scaffold fidelity, osteogenic differentiation and mineralization. Acta Biomaterialia. 2021;121:637-652. doi: 10.1016/j.actbio.2020.12.026
- Zhang X, Cui J, Cheng L, Lin K. Enhancement of osteoporotic bone regeneration by strontium-substituted 45S5 bioglass via time-dependent modulation of autophagy and the Akt/mTOR signaling pathway. J Mater Chem B. 2021;9(16):3489-3501. doi: 10.1039/d0tb02991b
- Souza L, Lopes JH, Encarnação D, et al. Comprehensive in vitro and in vivo studies of novel melt-derived Nb-substituted 45S5 bioglass reveal its enhanced bioactive properties for bone healing. Sci Rep. 2018;8(1):12808. doi: 10.1038/s41598-018-31114-0
- Bellucci D, Cannillo V, Sola A, Chiellini F, Gazzarri M, Migone C. Macroporous Bioglass®-derived scaffolds for bone tissue regeneration. Ceramics international. 2011;37(5):1575-1585. doi: 10.1016/j.ceramint.2011.01.023
- Wang X, Tolba E, Schröder HC, et al. Effect of bioglass on growth and biomineralization of SaOS-2 cells in hydrogel after 3D cell bioprinting. PLoS One. 2014;9(11):e112497. doi: 10.1371/journal.pone.0112497
- Raveendran N, Vaswani K, Han P, Basu S, Moran CS, Ivanovski S. Modeling inflammatory response using 3D bioprinting of polarized macrophages Int J Bioprint. 2024;10(2):2116. doi: 10.36922/ijb.2116
- Roh TT, Chen Y, Paul HT, Guo C, Kaplan DL. 3D bioengineered tissue model of the large intestine to study inflammatory bowel disease. Biomaterials. 2019;225:119517. doi: 10.1016/j.biomaterials.2019.119517
- Torras N, Zabalo J, Abril E, Carré A, García-Díaz M, Martínez E. A bioprinted 3D gut model with crypt-villus structures to mimic the intestinal epithelial-stromal microenvironment. Biomater Adv. 2023;153:213534. doi: 10.1016/j.bioadv.2023.213534
- Murphy SV, De Coppi P, Atala A. Opportunities and challenges of translational 3D bioprinting. Nat Biomed Eng. 2020;4(4):370-380. doi: 10.1038/s41551-019-0471-7
- Dickman CTD, Russo V, Thain K, et al. Functional characterization of 3D contractile smooth muscle tissues generated using a unique microfluidic 3D bioprinting technology. FASEB J. 2020;34(1):1652-1664. doi: 10.1096/fj.201901063RR
- Maes L, Szabó A, Van Haevermaete J, et al. P035 3D bioprinting of gelatin derivatives: towards novel small intestinal in vitro models. J Crohns Colitis. 2024;18(Supplement_1):i293-i293. doi: 10.1093/ecco-jcc/jjad212.0165
- Mon A, Gervacio S, Aidnik H, Oxford S, Rosette C, Piu F. Evaluation of the clinical stage FXR Agonist FXR314 in human primary cell 3D models of Crohn’s disease and ulcerative colitis. Inflamm Bowel Dis. 2024;30(Supplement_1):S59-S59. doi: 10.1093/ibd/izae020.121
- Lee JM, Yeong WY. Design and printing strategies in 3D bioprinting of cell-hydrogels: a review. Adv Healthc Mater. 2016;5(22):2856-2865. doi: 10.1002/adhm.201600435
- Almutary AG, Alnuqaydan AM, Almatroodi SA, Bakshi HA, Chellappan DK, Tambuwala MM. Development of 3D-bioprinted colitis-mimicking model to assess epithelial barrier function using albumin nano-encapsulated anti-inflammatory drugs. Biomimetics (Basel). 2023;8(1):41. doi: 10.3390/biomimetics8010041
- Garcés M, Cáceres L, Chiappetta D, Magnani N, Evelson P. Current understanding of nanoparticle toxicity mechanisms and interactions with biological systems. New J Chem. 2021;45(32):14328-14344. doi: 10.1039/D1NJ01415C
- Abbasi R, Shineh G, Mobaraki M, Doughty S, Tayebi L. Structural parameters of nanoparticles affecting their toxicity for biomedical applications: a review. J Nanopart Res. 2023;25(3):43. doi: 10.1007/s11051-023-05690-w
- Buzea C, Pacheco I. 28 – Toxicity of nanoparticles. In: Pacheco-Torgal F, Diamanti MV, Nazari A, Granqvist CG, Pruna A, Amirkhanian S, eds. Nanotechnology in Eco- Efficient Construction (Second Edition). Sawston, UK: Woodhead Publishing; 2019:705-754. doi: 10.1016/B978-0-08-102641-0.00028-1
- Egbuna C, Parmar VK, Jeevanandam J, et al. Toxicity of nanoparticles in biomedical application: nanotoxicology. J Toxicol. 2021;2021(1):9954443. doi: 10.1155/2021/9954443
- Ajdary M, Moosavi MA, Rahmati M, et al. Health concerns of various nanoparticles: a review of their in vitro and in vivo toxicity. Nanomaterials. 2018;8(9):634. doi: 10.3390/nano8090634
- Wang M, Yang Q, Long J, et al. A comparative study of toxicity of TiO2, ZnO, and Ag nanoparticles to human aortic smooth-muscle cells. Int J Nanomedicine. 2018: 8037-8049. doi: 10.2147/IJN.S188175
- Sanches PL, Geaquinto LRdO, Cruz R, et al. Toxicity evaluation of TiO2 nanoparticles on the 3D skin model: a systematic review. Frontiers in Bioengineering and Biotechnology. 2020;8:575. doi: 10.3389/fbioe.2020.00575
- Dubiak-Szepietowska M, Karczmarczyk A, Jönsson- Niedziółka M, Winckler T, Feller KH. Development of complex-shaped liver multicellular spheroids as a human-based model for nanoparticle toxicity assessment in vitro. Toxicol Appl Pharmacol. 2016;294:78-85. doi: 10.1016/j.taap.2016.01.016
- Chen L, Wu M, Jiang S, et al. Skin toxicity assessment of silver nanoparticles in a 3D epidermal model compared to 2D keratinocytes. Int J Nanomedicine. 2019:9707-9719. doi: 10.2147/IJN.S225451
- Gelamo EL, Tabak M. Spectroscopic studies on the interaction of bovine (BSA) and human (HSA) serum albumins with ionic surfactants. Spectrochim Acta Part A Mol Biomol Spectrosc. 2000;56(11):2255-2271. doi: 10.1016/S1386-1425(00)00313-9
- Miller KL, Sit I, Xiang Y, et al. Evaluation of CuO nanoparticle toxicity on 3D bioprinted human iPSC-derived cardiac tissues. Bioprinting. 2023;32:e00284. doi: 10.1016/j.bprint.2023.e00284
- Saufi SASA, Zuhri MYM, Dezaki ML, et al. Compression behaviour of bio-inspired honeycomb reinforced starfish shape structures using 3D printing technology. Polymers. 2021;13(24):4388. doi: 10.3390/polym13244388
- Bilberg K, Hovgaard MB, Besenbacher F, Baatrup E. In vivo toxicity of silver nanoparticles and silver ions in zebrafish (danio rerio). J Toxicol. 2012;2012:293784. doi: 10.1155/2012/293784
- Gerbolés AG, Galetti M, Rossi S, et al. Three-dimensional bioprinting of organoid-based scaffolds (OBST) for long-term nanoparticle toxicology investigation. Int J Mol Sci. 2023;24(7):6595. doi: 10.3390/ijms24076595
- Hossen S, Hossain MK, Basher M, Mia M, Rahman M, Uddin MJ. Smart nanocarrier-based drug delivery systems for cancer therapy and toxicity studies: a review. J Adv Res. 2019;15:1-18. doi: 10.1016/j.jare.2018.06.005
- Kashkooli FM, Soltani M, Souri M. Controlled anti-cancer drug release through advanced nano-drug delivery systems: static and dynamic targeting strategies. J Control Release. 2020;327:316-349. doi: 10.1016/j.jconrel.2020.08.012
- Hussain Z, Arooj M, Malik A, et al. Nanomedicines as emerging platform for simultaneous delivery of cancer therapeutics: new developments in overcoming drug resistance and optimizing anticancer efficacy. Artif Cells Nanomed Biotechnol. 2018;46(sup2):1015-1024. doi: 10.1080/21691401.2018.1478420
- Ahmad J, Garg A, Mustafa G, Mohammed AA, Ahmad MZ. 3D printing technology as a promising tool to design nanomedicine-based solid dosage forms: contemporary research and future scope. Pharmaceutics. 2023;15(5):1448. doi: 10.3390/pharmaceutics15051448
- Chakka JL, Salem AK. 3D Printing in Drug Delivery Systems. Taylor & Francis; 2019:59-62. doi: 10.2217/3dp-2019-0005
- Staros R, Michalak A, Rusinek K, Mucha K, Pojda Z, Zagożdżon R. Perspectives for 3D-bioprinting in modeling of tumor immune evasion. Cancers. 2022;14(13):3126. doi: 10.3390/cancers14133126
- Garrido-Mesa N, Zarzuelo A, Gálvez J. Minocycline: far beyond an antibiotic. Br J Pharmacol. 2013;169(2):337-352. doi: 10.1111/bph.12139
- Fu Z, Hai N, Zhong Y, Sun W. Printing GelMA bioinks: a strategy for buildingin vitromodel to study nanoparticle-based minocycline release and cellular protection under oxidative stress. Biofabrication. 2024;16(2):025040. doi: 10.1088/1758-5090/ad30c3
- Zhu H, Monavari M, Zheng K, et al. 3D bioprinting of multifunctional dynamic nanocomposite bioinks incorporating Cu‐doped mesoporous bioactive glass nanoparticles for bone tissue engineering. Small. 2022;18(12):2104996. doi: 10.1002/smll.202104996
- Zhu W, Cui H, Boualam B, et al. 3D bioprinting mesenchymal stem cell-laden construct with core–shell nanospheres for cartilage tissue engineering. Nanotechnology. 2018;29(18):185101. doi: 10.1088/1361-6528/aaafa1
- Rizzi F, Castaldo R, Latronico T, et al. High surface area mesoporous silica nanoparticles with tunable size in the sub-micrometer regime: insights on the size and porosity control mechanisms. Molecules (Basel, Switzerland). 2021;26(14):4247. doi: 10.3390/molecules26144247
- Theus AS, Ning L, Kabboul G, et al. 3D bioprinting of nanoparticle-laden hydrogel scaffolds with enhanced antibacterial and imaging properties. Iscience. 2022;25(9):104947. doi: 10.1016/j.isci.2022.1049
- Cleetus CM, Alvarez Primo F, Fregoso G, et al. Alginate hydrogels with embedded ZnO nanoparticles for wound healing therapy. Int J Nanomedicine. 2020: 5097-5111. doi: 10.2147/IJN.S255937
- Li Z, Zheng A, Mao Z, et al. Silk fibroin–gelatin photo-crosslinked 3D-bioprinted hydrogel with MOF-methylene blue nanoparticles for infected wound healing. Int J Bioprint. 2023;9(5):773. doi: 10.18063/ijb.773
- Su Y, Liu Y, Hu X, et al. Caffeic acid-grafted chitosan/sodium alginate/nanoclay-based multifunctional 3D-printed hybrid scaffolds for local drug release therapy after breast cancer surgery. Carbohydr Polym. 2024;324:121441. doi: 10.1016/j.carbpol.2023.121441
- Puertas-Bartolomé M, Włodarczyk-Biegun MK, del Campo A, Vázquez-Lasa B, San Román J. Development of bioactive catechol functionalized nanoparticles applicable for 3D bioprinting. Mater Sci Eng C. 2021;131:112515. doi: 10.1016/j.msec.2021.112515