3D bioprinting of in vitro full-thickness skin model with a rete ridge structure
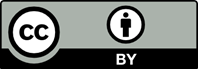
Rete ridges are undulating structures located between the epidermis and dermis that play a crucial role in maintaining the skin’s barrier function, protecting the body from pollutants and ultraviolet (UV) radiation. This formation of rete ridges within in vitro skin models is essential for evaluating the efficacy and safety of pharmaceuticals or cosmetics, replacing the need for animal models. Micro-molding is a prominent technique for recreating the rete ridge structure in in vitro skin models. However, this method is characterized by high technical difficulty. Although 3D bioprinting technology has demonstrated potential for fabricating skin models with the rete ridge structure, it is also limited by the extended time required for structural implementation. In this study, we introduce a methodology for fabricating full-thickness skin equivalents with rete ridges using preset extrusion bioprinting. A polycaprolactone frame was 3D-printed, and the dermal layer was bioprinted in the frame. Subsequently, furrowing steps were undertaken to create a substrate for the stable anchoring of strands produced by the preset extrusion bioprinting, fabricating a dermis layer with a rete ridge structure. Keratinocytes were then seeded and differentiated, forming the stratum corneum. We observed that protein expression was preserved in the valleys of the rete ridge after UV irradiation of the rete ridge full-thickness skin equivalent, similar to that of human ex vivo skin models. Therefore, the rete ridge full-thickness skin equivalent generated using our method exhibits potential as a promising model to replace animal experiments and human ex vivo skin models.

- Malaisse J, Pendaries V, Hontoir F, et al. Hyaluronan does not regulate human epidermal keratinocyte proliferation and differentiation. J Biol Chem. 2016;291(12):6347-6358. doi: 10.1074/jbc.M115.661348.
- Chamcheu JC, Afaq F, Syed DN, et al. Delphinidin, a dietary antioxidant, induces human epidermal keratinocyte differentiation but not apoptosis: studies in submerged and three-dimensional epidermal equivalent models. Exp Dermatol. 2013;22(5):342-348. doi: 10.1111/exd.12140.
- Mini CA, Dorta DJ, Maria-Engler SS, Oliveira DP. Immortalized equivalent human epidermis as a platform to evaluation hair dyes toxicity: efficiency comparison between 3D and monolayer culture. Chem Biol Interact. 2020;330:109227. doi: 10.1016/j.cbi.2020.109227.
- Jeong SH, Kim JH, Yi SM, et al. Assessment of penetration of quantum dots through in vitro and in vivo human skin using the human skin equivalent model and the tape stripping method. Biochem Biophys Res Commun. 2010; 394(3):612-615. doi: 10.1016/j.bbrc.2010.03.032.
- Liu N, Matsumura H, Kato T, et al. Stem cell competition orchestrates skin homeostasis and ageing. Nature. 2019;568(7752):344-350. doi: 10.1038/s41586-019-1085-7.
- Kim BS, Ahn M, Cho WW, Gao G, Jang J, Cho DW. Engineering of diseased human skin equivalent using 3D cell printing for representing pathophysiological hallmarks of type 2 diabetes in vitro. Biomaterials. 2021;272:120776. doi: 10.1016/j.biomaterials.2021.120776.
- Liu X, Michael S, Bharti K, Ferrer M, Song MJ. A biofabricated vascularized skin model of atopic dermatitis for preclinical studies. Biofabrication. 2020;12(3):035002. doi: 10.1088/1758-5090/ab76a1.
- Jang HJ, Lee JB, Yoon JK. Advanced in vitro three-dimensional skin models of atopic dermatitis. Tissue Eng Regen Med. 2023;20(4):539-552. doi: 10.1007/s13770-023-00532-1.
- Langton AK, Graham HK, McConnell JC, Sherratt MJ, Griffiths CEM, Watson REB. Organization of the dermal matrix impacts the biomechanical properties of skin. Br J Dermatol. 2017;177(3):818-827. doi: 10.1111/bjd.15353.
- Aleemardani M, Trikić MZ, Green NH, Claeyssens F. The importance of mimicking dermal-epidermal junction for skin tissue engineering: a review. Bioengineering (Basel). 2021;8(11):148. doi: 10.3390/bioengineering8110148.
- Giangreco A, Goldie SJ, Failla V, Saintigny G, Watt FM. Human skin aging is associated with reduced expression of the stem cell markers beta1 integrin and MCSP. J Invest Dermatol. 2010;130(2):604-608. doi: 10.1038/jid.2009.297.
- Liao YH, Kuo WC, Chou SY, et al. Quantitative analysis of intrinsic skin aging in dermal papillae by in vivo harmonic generation microscopy. Biomed Opt Express. 2014;5(9): 3266-3279. doi: 10.1364/BOE.5.003266.
- Krystyna JGK, Anna PJ, Markiewicz-Żukowska R, Socha K. The impact of ultraviolet radiation on skin photoaging – review of in vitro studies. J Cosmet Dermatol. 2021;20(11):3427-3431. doi: 10.1111/jocd.14033.
- Nagarajan MB, Ainscough AJ, Reynolds DS, et al. Biomimetic human skin model patterned with rete ridges. Biofabrication. 2023;16(1):015006. doi: 10.1088/1758-5090/acfc29.
- Shen Z, Cao Y, Li M, et al. Construction of tissue-engineered skin with rete ridges using co-network hydrogels of gelatin methacrylated and poly(ethylene glycol) diacrylate. Mater Sci Eng C Mater Biol Appl. 2021;129:112360. doi: 10.1016/j.msec.2021.112360.
- Bush KA, Pins GD. Development of microfabricated dermal epidermal regenerative matrices to evaluate the role of cellular microenvironments on epidermal morphogenesis. Tissue Eng Part A. 2012;18(21–22):2343-2353. doi: 10.1089/ten.TEA.2011.0479.
- Viswanathan P, Guvendiren M, Chua W, et al. Mimicking the topography of the epidermal-dermal interface with elastomer substrates. Integr Biol (Camb). 2016;8(1):21-29. doi: 10.1039/c5ib00238a.
- Blackstone BN, Malara MM, Baumann ME, McFarland KL, Supp DM, Powell HM. Fractional CO2 laser micropatterning of cell-seeded electrospun collagen scaffolds enables rete ridge formation in 3D engineered skin. Acta Biomater. 2020;102:287-297. doi: 10.1016/j.actbio.2019.11.051.
- Admane P, Gupta AC, Jois P, et al. Direct 3D bioprinted full-thickness skin constructs recapitulate regulatory signaling pathways and physiology of human skin. Bioprinting. 2019;15:e00051. doi: 10.1016/j.bprint.2019.e00051.
- Kang D, Ahn G, Kim D, et al. Pre-set extrusion bioprinting for multiscale heterogeneous tissue structure fabrication. Biofabrication. 2018;10(3):035008. doi: 10.1088/1758-5090/aac70b.
- Kang D, Hong G, An S, et al. Bioprinting of multiscaled hepatic lobules within a highly vascularized construct. Small. 2020;16(13):e1905505. doi: 10.1002/smll.201905505.
- Hong G, Kim J, Oh H, et al. Production of multiple cell-laden microtissue spheroids with a biomimetic hepatic-lobule-like structure. Adv Mater. 2021;33(36):e2102624. doi: 10.1002/adma.202102624.
- Kim JH, Park M, Shim JH, Yun WS, Jin S. Multi-scale vascularization strategy for 3D-bioprinted tissue using coaxial core-shell pre-set extrusion bioprinting and biochemical factors. Int J Bioprint. 2023; 9(4):726. doi: 10.18063/ijb.726.
- Girardeau-Hubert S, Lynch B, Zuttion F, et al. Impact of microstructure on cell behavior and tissue mechanics in collagen and dermal decellularized extra-cellular matrices. Acta Biomater. 2022;143:100-114. doi: 10.1016/j.actbio.2022.02.035.
- Samandari M, Alipanah F, Majidzadeh-A K, Alvarez MM, Trujillo-de Santiago G, Tamayol A. Controlling through designed 3D microcompartmentalization. Appl Phys Rev. 2021;8(2):021404. doi: 10.1063/5.0040732.
- Ober TJ, Foresti D, Lewis JA. Active mixing of complex fluids at the microscale. Proc Natl Acad Sci USA. 2015;112(40):12293-12298. doi: 10.1073/pnas.1509224112.
- Groisman A, Steinberg V. Efficient mixing at low Reynolds numbers using polymer additives. Nature. 2001;410(6831):905-908. doi: 10.1038/35073524.
- Timár F, Soós G, Szende B, Horváth A. Interdigitation index – a parameter for differentiating between young and older skin specimens. Skin Res Technol. 2000;6(1):17-20. doi: 10.1034/j.1600-0846.2000.006001017.x.
- Walton LA, Bradley RS, Withers PJ, et al. Morphological characterisation of unstained and intact tissue micro-architecture by X-ray computed micro- and nano-tomography. Sci Rep. 2015;5:10074. doi: 10.1038/srep10074.
- Battie C, Jitsukawa S, Bernerd F, Del Bino S, Marionnet C, Verschoore M. New insights in photoaging, UVA induced damage and skin types. Exp Dermatol. 2014; 23(Suppl 1):7-12. doi: 10.1111/exd.12388.
- Pageon H, Zucchi H, Rousset F, Monnier VM, Asselineau D. Skin aging by glycation: lessons from the reconstructed skin model. Clin Chem Lab Med. 2014;52(1):169-174. doi: 10.1515/cclm-2013-0091.
- Nishie W, Kiritsi D, Nyström A, Hofmann SC, Bruckner- Tuderman L. Dynamic interactions of epidermal collagen XVII with the extracellular matrix: laminin 332 as a major binding partner. Am J Pathol. 2011;179(2):829-837. doi: 10.1016/j.ajpath.2011.04.019.
- Clement AL, Moutinho TJ, Pins GD. Micropatterned dermal-epidermal regeneration matrices create functional niches that enhance epidermal morphogenesis. Acta Biomater. 2013;9(12):9474-9484. doi: 10.1016/j.actbio.2013.08.017.
- Malara MM, Blackstone BN, Baumann ME, Bailey JK, Supp DM, Powell HM. Cultured epithelial autograft combined with micropatterned dermal template forms rete ridges in vivo. Tissue Eng Part A. 2020;26(21–22):1138-1146. doi: 10.1089/ten.TEA.2020.0090.
- Tsatsou F, Trakatelli M, Patsatsi A, Kalokasidis K, Sotiriadis D. Extrinsic aging: UV-mediated skin carcinogenesis. Dermatoendocrinol. 2012;4(3):285-297. doi: 10.4161/derm.22519.
- Newton VL, Bradley RS, Seroul P, et al. Novel approaches to characterize age-related remodelling of the dermal-epidermal junction in 2D, 3D and in vivo. Skin Res Technol. 2017;23(2):131-148. doi: 10.1111/srt.12312.
- Meinhardt M, Krebs R, Anders A, Heinrich U, Tronnier H. Wavelength-dependent penetration depths of ultraviolet radiation in human skin. J Biomed Opt. 2008;13(4):044030. doi: 10.1117/1.2957970.
- Bosset S, Bonnet-Duquennoy M, Barre P, et al. Decreased expression of keratinocyte β1 integrins in chronically sun-exposed skin in vivo. Br J Dermatol. 2003;148(4):770-778. doi: 10.1046/j.1365-2133.2003.05159.x.
- Yaping X, Yingchun L, Yang Y, et al. Reduced expression of collagen 17A1 in naturally aged, photo aged, and UV-irradiated human skin in vivo: potential links to epidermal aging. J Cell Commun Signal. 2022;16(3):421-432. doi: 10.1007/s12079-021-00654-y.
- Marionnet C, Tricaud C, Bernerd F. Exposure to non-extreme solar UV daylight: spectral characterization, effects on skin and photoprotection. Int J Mol Sci. 2014;16(1):68-90. doi: 10.3390/ijms16010068.
- Marionnet C, de Dormael R, Marat X, et al. Sunscreens with the new MCE filter cover the whole UV spectrum: improved UVA1 photoprotection in vitro and in a randomized controlled trial. JID Innov. 2022;2(1):100070. doi: 10.1016/j.xjidi.2021.100070.
- Riccardo L, Tomasz J, Ruben GS, et al. From shape to function: the next step in bioprinting. Adv Mater. 2020;32(12):1906423. doi: 10.1002/adma.201906423.