3D-bioprinted gelatin methacryloyl hydrogel culture system emulating the oviduct environment for enhanced preimplantation embryo development
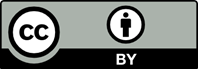
Oviducts have specific biomechanical properties that support fertilization and preimplantation embryo development, both of which are essential for successful pregnancy. However, conventional plastic-based human embryo culture does not recapitulate the biomechanical environment of the oviduct. Therefore, oviduct mimic culture systems that accurately emulate biophysical conditions for reproductive cells are a significant unmet clinical need. In the present study, we designed a three-dimensional (3D)-bioprinted optimal soft hydrogel system that accurately mimics the oviduct environment and investigated signaling factors during embryo development. We developed an oviduct tube-mimic hydrogel culture dish using gelatin methacryloyl (GelMA) 3D-bioprinted hydrogel. Quantitative assessment of hydrogel mechanical properties depended on the stiffness of the GelMA 3D-bioprinted hydrogel. Embryo quality was evaluated based on cleavage speed and blastocyst ratio on the GelMA hydrogel. Whole-transcriptome next-generation sequencing (NGS) analysis of embryos was used to identify biomechanical signaling factors. Our findings revealed that 10 kPa GelMA hydrogel culture conditions performed better with respect to development speed, blastocyst ratio, and hatching ratio than the control condition. Whole transcriptome NGS revealed up-regulation of mRNA processing genes and protein transport genes by the 7 and 10 kPa hydrogels. Furthermore, the inner cell mass and the number of Oct4+ cells were significantly higher in blastocysts cultured on 10 kPa hydrogel dishes than in those cultured on conventional hard plastic dishes. These findings demonstrate that optimized oviduct-mimic hydrogel-based 3D GelMA culture dishes could improve in vitro embryo development. Hence, 3D GelMA culture dishes may be useful as human embryo culture systems for assisted reproductive techniques.
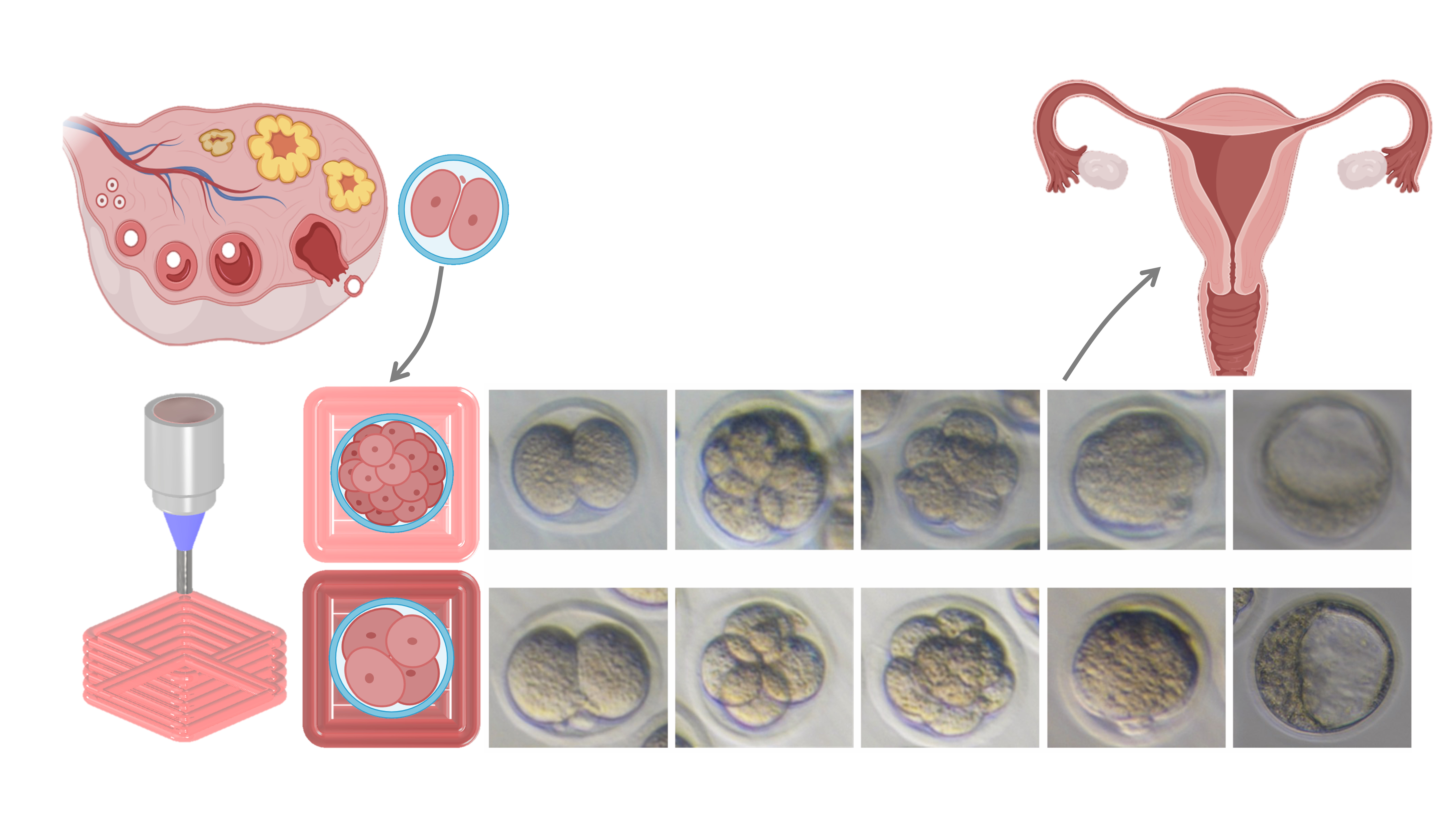
- Jain M, Singh M. Assisted reproductive technology (ART) techniques. In: StatPearls. Treasure Island (FL): StatPearls; 2023.
- Howie R, Kay V. Controlled ovarian stimulation for in-vitro fertilization. Br J Hosp Med (Lond). 2018;79:194-199. doi: 10.12968/hmed.2018.79.4.194
- Palermo GD, O’Neill CL, Chow S, et al. Intracytoplasmic sperm injection: state of the art in humans. Reproduction. 2017;154:F93-F110. doi: 10.1530/REP-17-0374
- Casciani V, Monseur B, Cimadomo D, Alvero R, Rienzi L. Oocyte and embryo cryopreservation in assisted reproductive technology: past achievements and current challenges. Fertil Steril. 2023;120:506-520. doi: 10.1016/j.fertnstert.2023.06.005
- Matsuzaki S. Mechanobiology of the female reproductive system. Reprod Med Biol. 2021;20:371-401. doi: 10.1002/rmb2.12404
- Hawkins J, Miao X, Cui W, Sun Y. Biophysical optimization of preimplantation embryo culture: what mechanics can offer ART. Mol Hum Reprod. 2021;27:gaaa087. doi: 10.1093/molehr/gaaa087
- Gu Z, Guo J, Wang H, Wen Y, Gu Q. Bioengineered microenvironment to culture early embryos. Cell Prolif. 2020;53:e12754. doi: 10.1111/cpr.12754
- Perez-Cerezales S, Ramos-Ibeas P, Acuna OS, et al. The oviduct: from sperm selection to the epigenetic landscape of the embryo. Biol Reprod. 2018;98:262-276. doi: 10.1093/biolre/iox173
- Korenaga M, Kadota, T. Changes in mechanical properties of the circular muscle of the isthmus of the human fallopian tube in relation to hormonal domination and postovulatory time. Fertil Steril. 1981;36:343-350. doi: 10.1016/s0015-0282(16)45736-5
- Aviles M, Gutierrez-Adan A, Coy P. Oviductal secretions: will they be key factors for the future ARTs? Mol Hum Reprod. 2010;16:896-906. doi: 10.1093/molehr/gaq056
- Gruber I, Klein M. Embryo culture media for human IVF: which possibilities exist? J Turk Ger Gynecol Assoc. 2011;12:110-117. doi: 10.5152/jtgga.2011.25
- Jafarbeglou F, Nazari MA, Keikhab F, Amanpour S, Azadi M. Visco-hyperelastic characterization of the mechanical properties of human fallopian tube tissue using atomic force microscopy. Materialia. 2021;16:101074. doi: 10.1016/j.mtla.2021.101074
- Di X, Gao X, Peng L, et al. Cellular mechanotransduction in health and diseases: from molecular mechanism to therapeutic targets. Signal Transduct Target Ther. 2023;8:282. doi: 10.1038/s41392-023-01501-9
- Luo C, Ding Z, Tu Y, Tan J, Luo Q, Song G. Biomaterial-based platforms for cancer stem cell enrichment and study. Cancer Biol Med. 2021;18:458-469. doi: 10.20892/j.issn.2095-3941.2020.0420
- d’Angelo M, Benedetti E, Tupone MG, et al. The role of stiffness in cell reprogramming: a potential role for biomaterials in inducing tissue regeneration. Cells. 2019;8(9):1036. doi: 10.3390/cells8091036.
- Janmey PA, Fletcher DA, Reinhart-King CA. Stiffness sensing by cells. Physiol Rev. 2020;100:695-724. doi: 10.1152/physrev.00013.2019.
- Miller CJ, Davidson LA. The interplay between cell signalling and mechanics in developmental processes. Nat Rev Genet. 2013;14:733-744. doi: 10.1038/nrg3513
- Shah JS, Sabouni R, Cayton Vaught KC, Owen CM, Albertini DF, Segars JH. Biomechanics and mechanical signaling in the ovary: a systematic review. J Assist Reprod Genet. 2018;35:1135-1148. doi: 10.1007/s10815-018-1180-y
- Vijayavenkataraman S, Yan WC, Lu WF, Wang CH, Fuh JYH. 3D bioprinting of tissues and organs for regenerative medicine. Adv Drug Deliv Rev. 2018;132:296-332. doi: 10.1016/j.addr.2018.07.004
- Mandrycky C, Wang Z, Kim K, Kim DH. 3D bioprinting for engineering complex tissues. Biotechnol Adv. 2016;34:422-434. doi: 10.1016/j.biotechadv.2015.12.011
- Chung JHY, Naficy S, Yue Z, et al. Bio-ink properties and printability for extrusion printing living cells. Biomater Sci. 2013;1:763-773. doi: 10.1039/c3bm00012e
- Schwab A, Levato R, D’Este M, Piluso S, Eglin D, Malda J. Printability and shape fidelity of bioinks in 3D bioprinting. Chem Rev. 2020;120:11028-11055. doi: 10.1021/acs.chemrev.0c00084
- Lee JM, Yeong WY. Design and printing strategies in 3d bioprinting of cell-hydrogels: a review. Adv Healthc Mater. 2016;5:2856-2865. doi: 10.1002/adhm.201600435
- Ying G, Manriquez J, Wu D, et al. An open-source handheld extruder loaded with pore-forming bioink for in situ wound dressing. Mater Today Bio. 2020;8:100074. doi: 10.1016/j.mtbio.2020.100074
- Wu T, Gao YY, Su J, et al. Three-dimensional bioprinting of artificial ovaries by an extrusion-based method using gelatin-methacryloyl bioink. Climacteric. 2022;25:170-178. doi: 10.1080/13697137.2021.1921726
- Amorim CA, Shikanov A. The artificial ovary: current status and future perspectives. Future Oncol. 2016;12:2323-2332. doi: 10.2217/fon-2016-0202
- Celikkin N, Mastrogiacomo S, Jaroszewicz J, Walboomers XF, Swieszkowski W. Gelatin methacrylate scaffold for bone tissue engineering: the influence of polymer concentration. J Biomed Mater Res A. 2018;106:201-209. doi: 10.1002/jbm.a.36226.
- Fathi E, Farahzadi R, Valipour B. Alginate/gelatin encapsulation promotes NK cells differentiation potential of bone marrow resident C-kit(+) hematopoietic stem cells. Int J Biol Macromol. 2021;177:317-327. doi: 10.1016/j.ijbiomac.2021.02.131
- McBeth C, Lauer J, Ottersbach M, Campbell J, Sharon A, Sauer-Budge AF. 3D bioprinting of GelMA scaffolds triggers mineral deposition by primary human osteoblasts. Biofabrication. 2017;9:015009. doi: 10.1088/1758-5090/aa53bd
- Krishnamoorthy S, Noorani B, Xu C. Effects of encapsulated cells on the physical-mechanical properties and microstructure of gelatin methacrylate Hydrogels. Int J Mol Sci. 2019;20(20):5061. doi: 10.3390/ijms20205061
- Martin JA, Wang Z. Next-generation transcriptome assembly. Nat Rev Genet. 2011;12(10):671-682. doi: 10.1038/nrg3068
- Jiang H, Lei R, Ding SW, Zhu S. Skewer: a fast and accurate adapter trimmer for next-generation sequencing paired-end reads. BMC Bioinformatics. 2014;15:182. doi: 10.1186/1471-2105-15-182
- Dobin A, Davis CA, Schlesinger F, et al. STAR: ultrafast universal RNA-seq aligner. Bioinformatics. 2013;29:15-21. doi: 10.1093/bioinformatics/bts635
- Trapnell C, Williams BA, Pertea G, et al. Transcript assembly and quantification by RNA-Seq reveals unannotated transcripts and isoform switching during cell differentiation. Nat Biotechnol. 2010;28:511-515. doi: 10.1038/nbt.1621
- Wu T, Hu E, Xu S, et al. clusterProfiler 4.0: a universal enrichment tool for interpreting omics data. Innovation (Camb). 2021;2:100141. doi: 10.1016/j.xinn.2021.100141
- Liu P, Shen H, Zhi Y, et al. 3D bioprinting and in vitro study of bilayered membranous construct with human cells- laden alginate/gelatin composite hydrogels. Colloids Surf B Biointerfaces. 2019;181:1026-1034. doi: 10.1016/j.colsurfb.2019.06.069
- Liu W, Heinrich MA, Zhou Y, et al. Extrusion bioprinting of shear-thinning gelatin methacryloyl bioinks. Adv Healthc Mater. 2017;6(12):10.1002/adhm.201601451. doi: 10.1002/adhm.201601451
- Li Y, Yang C, Khan M, et al. Nanostructured PEG-based hydrogels with tunable physical properties for gene delivery to human mesenchymal stem cells. Biomaterials. 2012;33: 6533-6541. doi: 10.1016/j.biomaterials.2012.05.043
- Seliktar D. Designing cell-compatible hydrogels for biomedical applications. Science. 2012;336(6085):1124-1128. doi: 10.1126/science.1214804
- Wang X, Wei C, Cao B, Jiang L, Hou Y, Chang J. Fabrication of multiple-layered hydrogel scaffolds with elaborate structure and good mechanical properties via 3D printing and ionic reinforcement. ACS Appl Mater Interfaces. 2018;10: 18338-18350. doi: 10.1021/acsami.8b04116
- Marikawa Y, Alarcon VB. Establishment of trophectoderm and inner cell mass lineages in the mouse embryo. Mol Reprod Dev. 2009;76:1019-1032. doi: 10.1002/mrd.21057
- Leesch F, Lorenzo-Orts L, Pribitzer C, et al. A molecular network of conserved factors keeps ribosomes dormant in the egg. Nature. 2023;613:712-720. doi: 10.1038/s41586-022-05623-y
- Wang D, Bu F, Zhang W. The role of ubiquitination in regulating embryonic stem cell maintenance and cancer development. Int J Mol Sci. 2019;20(11): 2667. doi: 10.3390/ijms20112667
- Chen L, Wang D, Wu Z, et al. Molecular basis of the first cell fate determination in mouse embryogenesis. Cell Res. 2010;20(9):982-993. doi: 10.1038/cr.2010.106
- Nelson CM. Mechanical control of cell differentiation: insights from the early embryo. Annu Rev Biomed Eng. 2022;24:307-322. doi: 10.1146/annurev-bioeng-060418-052527
- Carreiro LE, Dos Santos GS, Luedke FE, Goissis MD. Cell differentiation events in pre-implantation mouse and bovine embryos. Anim Reprod. 2022;7(18):e20210054. doi: 10.1590/1984-3143-AR2021-0054
- Ivec M, Kovacic B, Vlaisavljevic V. Prediction of human blastocyst development from morulas with delayed and/ or incomplete compaction, Fertil Steril. 2011;96(6): 1473-1478.e2. doi: 10.1016/j.fertnstert.2011.09.015
- Richter KS. Quantitative grading of a human blastocyst optimal inner cell mass size and shape. Fertil Steril. 2001;76:1157-1167. doi: 10.1016/s0015-0282(01)02870-9