A functionally graded gyroid-type three-periodic minimal surface framework applied to implant-supported fixed complete dentures
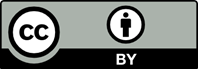
Titanium alloy, particularly Ti6Al4V, is commonly used for constructing the framework of implant-supported fixed complete dentures (IFCDs) but exhibits poor specific strength and impact toughness. Three-periodic minimal surface (TPMS) porous structures have the advantages of high specific strength, lightweight, and shock and energy absorption. Therefore, the functionally graded TPMS porous structure was adopted to design the framework for IFCDs in this study. Nine types of TPMS-based lattice structures with radial gradient variations were designed. Finite element analysis and experimental results indicate that the relative density increases outward and the cell size decreases outward from the center. The B-I porous structure has the highest strength and impact toughness compared to other gradient porous structure types. Moreover, the IFCD framework, utilizing the B-I porous structure, exhibited a 50% reduction in weight compared to the solid framework. When compared to the hollow framework with the same weight, the B-I framework demonstrated a 42.81% lower maximum equivalent stress under normal chewing conditions without undergoing plastic deformation. Therefore, the B-I framework meets the mechanical performance requirements for daily chewing and exhibits superior mechanical properties over conventional structures.
- Guo Y-q, Ma Y, Cai S-n, Yu H. Optimal impression materials for implant-supported fixed complete dentures: a systematic review and meta-analysis. J Prosthet Dent. 2023;Inpress. doi: 10.1016/j.prosdent.2023.06.024
- Cinquini C, Alfonsi F, Marchio V, et al. The use of zirconia for implant-supported fixed complete dental prostheses: a narrative review. Dent J (Basel). 2023;11(6):144. doi: 10.3390/dj11060144
- Vahnström M, Johansson PH, Svanborg P, Stenport VF. Comparison of porcelain veneer fracture in implant‐supported fixed full‐arch prostheses with a framework of either titanium, cobalt–chromium, or zirconia: an in vitro study. Clin Exp Dent Res. 2022;8(2):544-551. doi: 10.1002/cre2.558
- Alberto LHJ, Kalluri L, Esquivel-Upshaw JF, Duan Y. Three-dimensional finite element analysis of different connector designs for all-ceramic implant-supported fixed dental prostheses. Ceramics. 2022; 5(1):34-43. doi: 10.3390/ceramics5010004
- Thompson J, Schoenbaum TR, Pannu D, Knoernschild K. Survival analysis of zirconia implant-supported, fixed complete dentures: a 5-year retrospective cohort study. J Prosthet Dent. 2023;Inpress. doi: 10.1016/j.prosdent.2023.04.031
- Messias A, Nicolau P, Guerra F. Different interventions for rehabilitation of the edentulous maxilla with implant-supported prostheses: an overview of systematic reviews. Int J Prosthodont. 2021;34(Suppl):s63-s84. doi: 10.11607/ijp.7162
- Zhang L, Liu H, Yao H, Zeng Y, Chen J. Preparation, microstructure, and properties of ZrO2(3Y)/Al2O3 bioceramics for 3D printing of all-ceramic dental implants by vat photopolymerization. Chin J Mech Eng: Addit Manuf Front. 2022;1(2):100023. doi: 10.1016/j.cjmeam.2022.100023
- Baltatu MS, Țugui CA, Perju MC, et al. Biocompatible titanium alloys used in medical applications. Rev Chim. 2019;70(4):1302-1306. doi: 10.37358/RC.19.4.7114
- Nouri A, Hodgson PD, Wen C. Biomimetic porous titanium scaffolds for orthopedic and dental applications. Biomimetics Learn Nat. 2010;21:415-450. doi: 10.5772/8787
- Al-Meraikhi H, Yilmaz B, McGlumphy E, Brantley W, Johnston WM. In vitro fit of CAD-CAM complete arch screw-retained titanium and zirconia implant prostheses fabricated on 4 implants. J Prosthet Dent. 2018;119(3):409-416. doi: 10.1016/j.prosdent.2017.04.023
- Kanazawa M, Iwaki M, Minakuchi S, Nomura N. Fabrication of titanium alloy frameworks for complete dentures by selective laser melting. J Prosthet Dent. 2014;112(6): 1441-1447. doi: 10.1016/j.prosdent.2014.06.017
- Tribst JPM, Dal Piva AMO, Borges ALS, Rodrigues VA, Bottino MA, Kleverlaan CJ. Does the prosthesis weight matter? 3D finite element analysis of a fixed implant-supported prosthesis at different weights and implant numbers. J Adv Prosthodont. 2020;12(2):67-74. doi: 10.4047/jap.2020.12.2.67
- Zhang L, Feih S, Daynes S, et al. Energy absorption characteristics of metallic triply periodic minimal surface sheet structures under compressive loading. Addit Manuf. 2018;23:505-515. doi: 10.1016/j.addma.2018.08.007
- Tribst J, Rodrigues V, Dal Piva A, Borges A, Nishioka R. The importance of correct implants positioning and masticatory load direction on a fixed prosthesis. J Clin Exp Dent. 2018;10(1):e81-e87. doi: 10.4317/jced.54489
- Edelhoff D, Schubert O, Stimmelmayr M, Schweiger J. CAD/CAM full-mouth rehabilitation of an elderly patient: one-piece digital complete denture meets multilayered zirconia with gradient technology. J Esthet Restor Dent. 2024;36(1):174-185. doi: 10.1111/jerd.13031
- Ventura J, Jiménez-Castellanos E, Romero J, Enrile F. Tooth fractures in fixed full-arch implant-supported acrylic resin prostheses: a retrospective clinical study. Int J Prosthodont. 2016;29(2):161-165. doi: 10.11607/ijp.4400
- Mackert J, El-Shewy M, Pannu DS, Schoenbaum TR. Prosthetic complications and survival rates of metal-acrylic implant fixed complete dental prostheses: a retrospective study up to 10 years. J Prosthet Dent. 2022;Inpress. doi: 10.1016/j.prosdent.2022.06.019
- Fischer K, Stenberg T. Prospective 10-year cohort study based on a randomized, controlled trial (RCT) on implant-supported full-arch maxillary prostheses. part II: prosthetic outcomes and maintenance. Clin Implant Dent Relat Res. 2013;15(4):498-508. doi: 10.1111/j.1708-8208.2011.00383.x
- Melchels FPW, Bertoldi K, Gabbrielli R, Velders AH, Feijen J, Grijpma DW. Mathematically defined tissue engineering scaffold architectures prepared by stereolithography. Biomaterials. 2010;31(27):6909-6916. doi: 10.1016/j.biomaterials.2010.05.068
- Toosi S, Javid-Naderi MJ, Tamayol A, Ebrahimzadeh MH, Yaghoubian S, Mousavi Shaegh SA. Additively manufactured porous scaffolds by design for treatment of bone defects. Front Bioeng Biotechnol. 2024;11:1252636. doi: 10.3389/fbioe.2023.1252636
- Catchpole-Smith S, Sélo RRJ, Davis A, Ashcroft I, Tuck CJ, Clare AT. Thermal conductivity of TPMS lattice structures manufactured via laser powder bed fusion. Addit Manuf. 2019;30(4):100846. doi: 10.1016/j.addma.2019.100846
- Wei SS, Zhang JL, Zhang L, et al. Laser powder bed fusion additive manufacturing of NiTi shape memory alloys: a review. Int J Extreme Manuf. 2023;5(3):032001. doi: 10.1088/2631-7990/acc7d9
- Dong Z, Han CJ, Zhao YZ, et al. Role of heterogenous microstructure and deformation behavior in achieving superior strength-ductility synergy in zinc fabricated via laser powder bed fusion. Int J Extrem Manuf. 2024;6(4):045003. doi: 10.1088/2631-7990/ad3929
- Kapfer SC, Hyde ST, Mecke K, Arns CH, Schröder-Turk GE. Minimal surface scaffold designs for tissue engineering. Biomaterials. 2011;32(29):6875-6882. doi: 10.1016/j.biomaterials.2011.06.012
- Dwivedi A, Khurana MK, Bala YG. Heat-treated nickel alloys produced using laser powder bed fusion-based additive manufacturing methods: a review. Chinese J Mech Eng Additive Manuf Front. 2023;2(3):100087. doi: 10.1016/j.cjmeam.2023.100087
- Wu LY, Xue J, Tian X, Liu T, Li D. 3D-printed metamaterials with versatile functionalities. Chin J Mech Eng Additive Manuf Front. 2023;2(3):100091. doi: 10.1016/j.cjmeam.2023.100091
- Jin Y, Zou S, Pan B, Li G, Shao L, Du J. Biomechanical properties of cylindrical and twisted triply periodic minimal surface scaffolds fabricated by laser powder bed fusion. Addit Manuf. 2022;56:102899. doi: 10.1016/j.addma.2022.102899
- Xue L, Atli KC, Picak S, et al. Controlling martensitic transformation characteristics in defect-free NiTi shape memory alloys fabricated using laser powder bed fusion and a process optimization framework. Acta Mater. 2021; 215:117017. doi: 10.1016/j.actamat.2021.117017
- Zhang X-Y, Yan X-C, Fang G, Liu M. Biomechanical influence of structural variation strategies on functionally graded scaffolds constructed with triply periodic minimal surface. Addit Manuf. 2020;32:101015. doi: 10.1016/j.addma.2019.101015
- Novak N, Al‐Ketan O, Borovinšek M, et al. Development of novel hybrid TPMS cellular lattices and their mechanical characterisation. J Mater Res Technol. 2021; 15:1318-1329. doi: 10.1016/j.jmrt.2021.08.092
- Zhang X-Y, Fang G, Leeflang S, Zadpoor AA, Zhou J. Topological design, permeability and mechanical behavior of additively manufactured functionally graded porous metallic biomaterials. Acta Biomater. 2019; 84:437-452. doi: 10.1016/j.actbio.2018.12.013
- Jiulu J, Siqi W, Lei Y, et al. Ni–Ti multicell interlacing gyroid lattice structures with ultra-high hyperelastic response fabricated by laser powder bed fusion. Int J Mach Tools Manuf. 2024;195:0890-6955. doi: 10.1016/j.ijmachtools.2023.104099
- Al‐Ketan O, Lee D-W, Rowshan R, Abu Al-Rub RK. Functionally graded and multi-morphology sheet TPMS lattices: design, manufacturing, and mechanical properties. J Mech Behav Biomed Mater. 2019;102:103520. doi: 10.1016/j.jmbbm.2019.103520
- Castro APG, Ruben RB, Gonçalves SB, Pinheiro J, Guedes JM, Fernandes PR. Numerical and experimental evaluation of TPMS Gyroid scaffolds for bone tissue engineering. Comput Methods Biomech Biomed Engin. 2019;22(6): 567-573. doi: 10.1080/10255842.2019.1569638
- Zhang Y-R, Du W-P, Zhou X, Yu H-y. Review of research on the mechanical properties of the human tooth. Int J Oral Sci. 2014;6(2):61-69. doi: 10.1038/ijos.2014.21
- Gabrieli R, Wenger R, Mazza M, Verné E, Baino F. Design, stereolithographic 3D printing, and characterization of TPMS scaffolds. Materials (Basel). 2024;17(3):654. doi: 10.3390/ma17030654
- Wang X, Xu S, Zhou S, et al. Topological design and additive manufacturing of porous metals for bone scaffolds and orthopaedic implants: a review. Biomaterials. 2016;83: 127-141. doi: 10.1016/j.biomaterials.2016.01.012
- Hu J, Wang S, Li B, Li F, Luo Z, Liu L. Efficient representation and optimization for TPMS-based porous structures. IEEE Trans Vis Comput Graph. 2022;28(7):2615-2627. doi: 10.1109/TVCG.2020.3037697
- Al‐Ketan O, Abu Al-Rub RK. MSLattice: a free software for generating uniform and graded lattices based on triply periodic minimal surfaces. Mater Des Process Commun. 2021;3(6):e205. doi: 10.1002/mdp2.205
- Fan Z. On the young’s moduli of Ti6Al4V alloys. Scrip Metall Material. 1993;29(11):1427-1432. doi: 10.1016/0956-716X(93)90331-L
- Bai L. Multi-objective structural optimization design of Ti6Al4V lattice structure formed by SLM. J Mech Eng. 2018;54(5):156-165. doi: 10.3901/JME.2018.05.156
- Yang L, Yan C, Han C, Chen P, Yang S, Shi Y. Mechanical response of a triply periodic minimal surface cellular structures manufactured by selective laser melting. Int J Mech Sci. 2018;148:149-157. doi: 10.1016/j.ijmecsci.2018.08.039
- Rene JS, Smita S, Satish S, Buvaneshwari A, Velmurugan N. Impact of Contracted Endodontic Access Cavities on the Fracture Resistance of Endodontically Treated Teeth After Mechanical Aging by Simulated Chewing Forces. J Endodont. 2023;49(9):1176-1182. doi: 10.1016/j.joen.2023.06.018
- Guo W, Yang Y, Liu C, et al. 3D printed TPMS structural PLA/GO scaffold: process parameter optimization, porous structure, mechanical and biological properties. J Mech Behav Biomed Mater. 2023;142:105848. doi: 10.1016/j.jmbbm.2023.105848
- Sun Q, Sun J, Guo K, Wang L. Compressive mechanical properties and energy absorption characteristics of SLM fabricated Ti6Al4V triply periodic minimal surface cellular structures. Mech Mater. 2022;166:104241. doi: 10.1016/j.mechmat.2022.104241
- Dumas M, Terriault P, Brailovski VJM. Modeling and characterization of a porosity graded lattice structure for additively manufactured biomaterials. Mater Design. 2017;121:383-392. doi: 10.1016/j.matdes.2017.02.021
- Na Q, Yuheng W, Yijun S, Jianguang F. Experimental and numerical studies on mechanical properties of TPMS structures. Int J Mech Sci. 2024;261:108657. doi: 10.1016/j.ijmecsci.2023.108657
- Papazoglou DP, Neidhard-Doll AT, Pinnell MF, Erdahl DS, Osborn TH. Compression and tensile testing of L-PBF Ti- 6Al-4V lattice structures with biomimetic porosities and strut geometries for orthopedic implants. Metals. 2024;2:232. doi: 10.3390/met14020232