Investigating the effect of pregabalin on neuronal development using ultrashort self-assembling peptides: Assessing 3D neuronal cultures with high throughput robotic 3D bioprinting
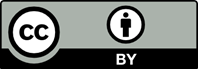
Pregabalin is a widely prescribed drug for various neurological disorders, yet its effects on embryonic cortical neuron development when given to pregnant women remain inadequately explored. In this study, we employed advanced three-dimensional (3D) culturing and in-house developed high-throughput robotic 3D bioprinting technologies to evaluate their potential in neuropharmacology applications, using pregabalin as a model compound. Using a robotic 3D bioprinter and tetrameric IIZK peptide hydrogel as bioink, we created constructs with pregabalin-treated and untreated primary mouse embryonic cortical neurons. This setup allowed us to study the drug’s effects on cell viability, expression of neuronal markers, and neuron development. Our comparative analysis between 2D and 3D peptide-based cell culture models revealed that at a therapeutic concentration of 10 μM, pregabalin does not affect neuronal viability or the morphogenesis of cortical neurons. However, it significantly alters adenosine triphosphate (ATP) release, suggesting potential disruptions in mitochondrial function. Moreover, gene expression analysis of key genes involved in the development of the forebrain and the differentiation and maturation of neurons revealed significant alterations, including the downregulation of Dlx2, Nhlh2, Otp, and Gad67. These findings, together with observed alterations in neuronal activity and oscillations, emphasize the complex impact of pregabalin on neuronal development and function. They highlight the necessity for comprehensive clinical evaluations of its use during pregnancy. Furthermore, our research demonstrates the feasibility and value of integrating 3D cultures with high-throughput 3D bioprinting in neuropharmacology, opening new avenues for investigating drug effects on neuronal development and function, and contributing to safer clinical practices.
- Alles SR, Cain SM, Snutch TP. Pregabalin as a pain therapeutic: beyond calcium channels. Front Cell Neurosci. 2020;14:83. doi: 10.3389/fncel.2020.00083
- Cross AL, Viswanath O, Sherman Al, Pregabalin I. StatPearls. Treasure Island, FL, USA: StatPearls Publishing; 2023. Available online: (accessed on 1 June, 2023). https://www.ncbi.nlm.nih.gov/books/NBK470341/
- Bidari A, Moazen-Zadeh E, Ghavidel-Parsa B, Rahmani S, Hosseini S, Hassankhani A. Comparing duloxetine and pregabalin for treatment of pain and depression in women with fibromyalgia: an open-label randomized clinical trial. DARU. 2019;27(1):149-158. doi: 10.1007/s40199-019-00257-4
- Moulin D, Boulanger A, Clark A, et al. Pharmacological management of chronic neuropathic pain: revised consensus statement from the Canadian Pain Society. Pain Res Manag. 2014;19(6):328-335. doi: 10.1155/2014/754693
- Dworkin RH, Corbin AE, Young JP, et al. Pregabalin for the treatment of postherpetic neuralgia: a randomized, placebo-controlled trial. Neurology. 2003;60(8):1274-1283. doi: 10.1212/01.wnl.0000055433.55136.55
- Lesser H, Sharma U, LaMoreaux L, Poole RM. Pregabalin relieves symptoms of painful diabetic neuropathy: a randomized controlled trial. Neurology. 2004;63(11): 2104-2110. doi: 10.1212/01.wnl.0000145767.36287.a1
- Elgazzar FM, Elseady WS, Hafez AS. Neurotoxic effects of pregabalin dependence on the brain frontal cortex in adult male albino rats. Neurotoxicology. 2021;83:146-155. doi: 10.1016/j.neuro.2021.01.004
- Morse DC. Embryo‐fetal developmental toxicity studies with pregabalin in mice and rabbits. Birth Defects Res B Dev Reprod Toxicol. 2016;107(2):85-93. doi: 10.1002/bdrb.21174
- Kułak-Bejda A, Waszkiewicz N, Popławska R, Bejda G. The impact of high doses of pregabalin on pregnancy–case report. Psychiatry Clin Psychopharmacol. 2019;29(1):97-99. doi: 10.1080/24750573.2018.1505452
- Cross AL, Viswanath O, Sherman AL. Pregabalin. StatPearls [Internet]; 2021. Available online: (accessed April 17, 2023). https://www.ncbi.nlm.nih.gov/books/NBK470341/
- Tassone DM, Boyce E, Guyer J, Nuzum D. Pregabalin: a novel γ-aminobutyric acid analogue in the treatment of neuropathic pain, partial-onset seizures, and anxiety disorders. Clin Ther. 2007;29(1):26-48. doi: 10.1016/j.clinthera.2007.01.013
- Dudukina E, Szépligeti SK, Karlsson P, et al. Prenatal exposure to pregabalin, birth outcomes and neurodevelopment – a population-based cohort study in four Nordic countries. Drug Saf. 2023;46(7):661-675. doi: 10.1007/s40264-023-01307-2
- Winterfeld U, Merlob P, Baud D, et al. Pregnancy outcome following maternal exposure to pregabalin may call for concern. Neurology. 2016;86(24):2251-2257. doi: 10.1212/WNL.0000000000002767
- Richardson JL, Damkier P, Diav-Citrin O, et al. A critical appraisal of controlled studies investigating malformation risks following pregabalin use in early pregnancy. Br J Clin Pharmacol. 2023;89(2):630-640. doi: 10.1111/bcp.15607
- Black E, Khor KE, Kennedy D, et al. Medication use and pain management in pregnancy: a critical review. Pain Pract. 2019;19(8):875-899. doi: 10.1111/papr.12814.
- Bonnet U, Scherbaum N. How addictive are gabapentin and pregabalin? A systematic review. Eur Neuropsychopharmacol. 2017;27(12):1185-1215. doi: 10.1016/j.euroneuro.2017.08.430
- Patorno E, Bateman BT, Huybrechts KF, et al. Pregabalin use early in pregnancy and the risk of major congenital malformations. Neurology. 2017;88(21):2020-2025. doi: 10.1212/WNL.0000000000003959
- Althobaiti YS, Almutairi FM, Alshehri FS, et al. Involvement of the dopaminergic system in the reward-related behavior of pregabalin. Sci Rep. 2021;11(1):10577. doi: 10.1038/s41598-021-88429-8
- Alsanie WF, Alhomrani M, Gaber A, et al. The effects of prenatal exposure to pregabalin on the development of ventral midbrain dopaminergic neurons. Cells. 2022;11(5);852. doi: 10.3390/cells11050852
- Lemon RN, Baker SN, Kraskov A. Classification of cortical neurons by spike shape and the identification of pyramidal neurons. Cereb Cortex. 2021;31(11):5131-5138. doi: 10.1093/cercor/bhab147.
- Yook C, Kim K, Kim D, et al. A TBR1-K228E mutation induces Tbr1 upregulation, altered cortical distribution of interneurons, increased inhibitory synaptic transmission, and autistic-like behavioral deficits in mice. Front Mol Neurosci. 2019;12:241. doi: 10.3389/fnmol.2019.00241
- Klionsky DJ, Abdalla FC, Abeliovich H, Abraham RT, Acevedo-Arozena A, Adeli K, et al. Guidelines for the use and interpretation of assays for monitoring autophagy. Autophagy. 2012;8(4):445–544 doi: 10.4161/auto.19496
- Bedogni F, Hodge RD, Elsen GE, et al. Tbr1 regulates regional and laminar identity of postmitotic neurons in developing neocortex. Proc Natl Acad Sci USA. 2010;107(29): 13129-13134. doi: 10.1073/pnas.1002285107
- Hevner RF, Shi L, Justice N, et al. Tbr1 regulates differentiation of the preplate and layer 6. Neuron. 2001;29(2):353-366. doi: 10.1016/s0896-6273(01)00211-2
- Abdelrahman S, Ge R, Susapto HH, et al. The impact of mechanical cues on the metabolomic and transcriptomic profiles of human dermal fibroblasts cultured in ultrashort self-assembling peptide 3d scaffolds. ACS Nano. 2023;17(15):14508-14531. doi: 10.1021/acsnano.3c01176
- Hauser CA, Deng R, Mishra A, et al. Natural tri- to hexapeptides self-assemble in water to amyloid beta-type fiber aggregates by unexpected alpha-helical intermediate structures. Proc Natl Acad Sci USA. 2011;108(4):1361-1366. doi: 10.1073/pnas.1014796108
- Mishra A, Loo Y, Deng R, et al. Ultrasmall natural peptides self-assemble to strong temperature-resistant helical fibers in scaffolds suitable for tissue engineering. Nano Today. 2011;6(3):232-239. doi: 10.1016/j.nantod.2011.05.001
- Abdelrahman S, Alsanie WF, Khan ZN, et al. A Parkinson’s disease model composed of 3D bioprinted dopaminergic neurons within a biomimetic pep-tide scaffold. Biofabrication. 2022;14(4):044103. doi: 10.1088/1758-5090/ac7eec.
- Susapto HH, Alhattab D, Abdelrahman S, et al. ultrashort peptide bioinks support automated printing of large-scale constructs assuring long-term survival of printed tissue constructs. Nano Lett. 2021;21(7):2719-2729. doi: 10.1021/acs.nanolett.0c04426
- Alhattab DM, Isaioglou I, Alshehri S, et al. Fabrication of a three-dimensional bone marrow niche-like acute myeloid leukemia disease model by an automated and controlled process using a robotic multicellular bioprinting system. Biomater Res. 2023;27(1):111. doi: 10.1186/s40824-023-00457-9.
- Hayashi H, Yamada M, Kumai J, Takagi N, Nomizu, M. Biological activities of laminin-111-derived peptide-chitosan matrices in a primary culture of rat cortical neurons. Arch Biochem Biophys. 2018;648:53-59. doi: 10.1016/j.abb.2018.04.010
- Alsanie WF, Bahri OA, Habeeballah HH, et al. Generating homogenous cortical preplate and deep-layer neurons using a combination of 2D and 3D differentiation cultures. Sci Rep. 2020;10(1):6272. doi: 10.1038/s41598-020-62925-9
- Alsanie W, Penna V, Schachner M, Thompson L, Parish C. Homophilic binding of the neural cell adhesion molecule CHL1 regulates development of ventral midbrain dopaminergic pathways. Sci Rep. 2017;7:9368. doi: 10.1038/s41598-017-09599-y
- Kahin K, Khan Z, Albagami M, et al. Development of a robotic 3D bioprinting and microfluidic pumping system for tissue and organ engineering. In: Microfluidics, BioMEMS, and Medical Microsystems XIX. SPIE-Intl Soc Optical Eng; 2019. doi: 10.1117/12.2507237
- Khan Z, Kahin K, Rauf S, et al. Optimization of a 3D bioprinting process using ultrashort peptide bioinks. Int J Bioprint. 2018;5(1):173. doi: 10.18063/ijb.v5i1.173
- Khan Z, Kahin K, Hauser CAE. Time-dependent pulsing of microfluidic pumps to enhance 3D bioprinting of peptide bioinks. In: Microfluidics, BioMEMS, and Medical Microsystems XIX. SPIE-Intl Soc Optical Eng.; 2021. doi: 10.1117/12.2578830
- Goriounova NA, Mansvelder HD. Genes, cells and brain areas of intelligence. Front Hum Neurosci. 2019;13:44. doi: 10.3389/fnhum.2019.00044
- Coleman JR, Bryois J, Gaspar HA, et al. Biological annotation of genetic loci associated with intelligence in a meta-analysis of 87,740 individuals. Mol Psychiatry. 2019;24:182-197. doi: 10.1038/s41380-018-0040-6
- Trampush JW, Yang MLZ, Yu J., et al. GWAS meta-analysis reveals novel loci and genetic correlates for general cognitive function: a report from the COGENT consortium. Mol Psychiatry. 2017;22:336-345. doi: 10.1038/mp.2016.244
- Won H, Huang J, Opland CK, Hartl CL, Geschwind DH. Human evolved regulatory elements modulate genes in-volved in cortical expansion and neurodevelopmental disease susceptibility. Nat Commun. 2019; 0:2396. doi: 10.1038/s41467-019-10248-3
- Alsanie WF, Abdelrahman S, Alhomrani M, et al. The influence of prenatal exposure to quetiapine fumarate on the development of dopaminergic neurons in the ventral midbrain of mouse embryos. Int J Mol Sci. 2022;23:12352. doi: 10.3390/ijms232012352
- Yuan F, Fang K-H, Cao S-Y, et al. Efficient generation of region-specific forebrain neurons from human pluripotent stem cells under highly defined condition. Sci Rep. 2015;5(1):18550. doi: 10.1038/srep18550
- Kriegstein A, Noctor S, Martínez-Cerdeño V. Patterns of neural stem and progenitor cell division may underlie evolutionary cortical expansion. Nat Rev Neurosci. 2006;7(11):883-890. doi: 10.1038/nrn2008
- Rakic P. Evolution of the neocortex: a perspective from developmental biology. Nat Rev Neurosci. 2009;10(10): 724-735. doi: 10.1038/nrn2719
- Lu I-L, Chen C, Tung C-Y, et al. Identification of genes associated with cortical malformation using a transposon-mediated somatic mutagenesis screen in mice. Nat Commun. 2018;9(1):2498. doi: 10.1038/s41467-018-04880-8
- Desikan RS, Barkovich AJ. Malformations of cortical development. Ann Neurol. 2016;80(6):797-810. doi: 10.1002/ana.24793
- Blümcke I, Vinters HV, Armstrong D, Aronica E, Thom M, Spreafico R. Malformations of cortical development and epilepsies. Epileptic Disord. 2009;11:181-193. doi: 10.1101/cshperspect.a022392
- Ikeda H, Yonemochi N, Ardianto C, Yang L, Kamei J. Pregabalin increases food intake through dopaminergic systems in the hypothalamus. Brain Res. 2018;1701:219-226. doi: 10.1016/j.brainres.2018.09.026
- Chagury AA, Tavares KR, Camargo RM, Martins DV, Marques LH, Mahmoud A. Pregabalin treatment in a pregnant woman with glossopharyngeal neuralgia. Braz J Otorhinolaryngol. 2020;86:S17-S19. doi: 10.1016/j.bjorl.2017.01.010
- Wettermark B, Brandt L, Kieler H, Bodén R. Pregabalin is increasingly prescribed for neuropathic pain, generalised anxiety disorder and epilepsy but many patients discontinue treatment. Int J Clin Pract. 2014;68(1):104-110. doi: 10.1111/ijcp.12182
- Derry S, Bell RF, Straube S, Wiffen PJ, Aldington D, Moore RA. Pregabalin for neuropathic pain in adults. Cochrane Database Syst Rev. 2019;2019(1):CD007076. doi: 10.1002/14651858.CD007076.pub3
- Ben-Menachem E. Pregabalin pharmacology and its relevance to clinical practice. Epilepsia. 2004;45:13-18. doi: 10.1111/j.0013-9580.2004.455003.x
- Sałat K, Librowski T, Nawiesniak B, Gluch-Lutwin M. Evaluation of analgesic, antioxidant, cytotoxic and metabolic effects of pregabalin for the use in neuropathic pain. Neurol Res. 2013;35(9):948-958. doi: 10.1179/1743132813Y.0000000236
- Margulis AV, Hernandez-Diaz S, McElrath T, et al. Relation of in-utero exposure to antiepileptic drugs to pregnancy duration and size at birth. PLoS One. 2019;14(8):e0214180. doi: 10.1371/journal.pone.0214180
- Andrade C. Safety of pregabalin in pregnancy. J Clin Psychiatry. 2018;79(5):18f12568. doi: 10.4088/JCP.18f12568
- Alsanie WF, Abdelrahman S, Alhomrani M, et al. Prenatal exposure to gabapentin alters the development of ventral midbrain dopaminergic neurons. Front Pharmacol. 2022;13:923113. doi: 10.3389/fphar.2022.923113
- Saino‐Saito S, Berlin R, Baker H. Dlx‐1 and Dlx‐2 expression in the adult mouse brain: relationship to dopaminergic phenotypic regulation. J Comp Neurol. 2003;461(1):18-30. doi: 10.1002/cne.10611
- Cecchi C. Emx2: a gene responsible for cortical development, regionalization and area specification. Gene. 2002; 291(1-2):1-9. doi: 10.1016/s0378-1119(02)00623-6
- Pei Z, Wang B, Chen G, Nagao M, Nakafuku M, Campbell K. Homeobox genes Gsx1 and Gsx2 differentially regulate telencephalic progenitor maturation. Proc Natl Acad Sci USA. 2011;108(4):1675-1680. doi: 10.1073/pnas.1008824108
- Bishop JH, Faerman A, Geoly A, et al. Altered neurotransmitter ratio in the prefrontal cortex is associated with pain in fibromyalgia syndrome. medRxiv. 2021;2021:21265618. doi: 10.1101/2021.10.28.21265618
- Jiang H, Fang D, Kong L-Y, et al. Sensitization of neurons in the central nucleus of the amygdala via the decreased GABAergic inhibition contributes to the development of neuropathic pain-related anxiety-like behaviors in rats. Mol Brain. 2014;7:72. doi: 10.1186/s13041-014-0072-z
- Alifragis P, Liapi A. Parnavelas JG. Lhx6 regulates the migration of cortical interneurons from the ventral telencephalon but does not specify their GABA phenotype. J Neurosci. 2004;24:5643-5648. doi: 10.1523/JNEUROSCI.1245-04.2004
- Good DJ, Braun T. NHLH2: at the intersection of obesity and fertility. Trends Endocrinol Metab. 2013;24(8):385-390. doi: 10.1016/j.tem.2013.04.003
- Takebayashi H, Yoshida S, Sugimori M, et al. Dynamic expression of basic helix-loop-helix Olig family members: implication of Olig2 in neuron and oligodendrocyte differentiation and identification of a new member, Olig3. Mech Dev. 2000;99:143-148. doi: 10.1016/s0925-4773(00)00466-4
- Meijer DH, Kane MF, Mehta S, et al. Separated at birth? The functional and molecular divergence of OLIG1 and OLIG2. Nat Rev Neurosci. 2012;13(12):819-831. doi: 10.1038/nrn3386
- Wang H, Xu L, Lai C, et al. Region-specific distribution of Olig2-expressing astrocytes in adult mouse brain and spinal cord. Mol Brain. 2021;14:1-14. doi: 10.1186/s13041-021-00747-0
- Jakovcevski I, Zecevic N. Olig transcription factors are expressed in oligodendrocyte and neuronal cells in human fetal CNS. J Neurosci. 2005;25(44):10064-10073. doi: 10.1523/JNEUROSCI.2324-05.2005
- Aruga J. The role of Zic genes in neural development. Mol Cell Neurosci. 2004;26(2):205-221. doi: 10.1016/j.mcn.2004.01.004
- Inoue T, Ota M, Ogawa M, Mikoshiba K, Aruga J. Zic1 and Zic3 regulate medial forebrain development through expansion of neuronal progenitors. J Neurosci. 2007;27(20):5461-5473. doi: 10.1523/JNEUROSCI.4046-06.2007
- Butt SJ, Sousa VH, Fuccillo MV, et al. The requirement of Nkx2-1 in the temporal specification of cortical interneuron subtypes. Neuron. 2008;59(5):722-732. doi: 10.1016/j.neuron.2008.07.031
- Hundehege P, Fernandez-Orth J, Römer P, et al. Targeting voltage-dependent calcium channels with pregabalin exerts a direct neuroprotective effect in an animal model of multiple sclerosis. Neurosignals. 2018;26(1):77-93. doi: 10.1159/000495425.
- Ablinger C, Geisler SM, Stanika RI, Klein CT, Obermair GJ. Neuronal α2δ proteins and brain disorders. Pflügers Arch. 2020;472(7):845-863. doi: 10.1007/s00424-020-02420-2
- Bockbrader HN, Wesche D, Miller R, Chapel S, Janiczek N, Burger P. A comparison of the pharmacokinetics and pharmacodynamics of pregabalin and gabapentin. Clin Pharmacokinet. 2010;49(10):661-669. doi: 10.2165/11536200-000000000-00000
- Mazzocchi A, Soker S, Skardal A. 3D bioprinting for high-throughput screening: Drug screening, disease modeling, and precision medicine applications. Appl Phys Rev. 2019;6(1):011302. doi: 10.1063/1.5056188
- Satpathy A, Datta P, Wu Y, Ayan B, Bayram E, Ozbolat IT. Developments with 3D bioprinting for novel drug discovery. Expert Opin Drug Discov. 2018;13(12):1115-1129. doi: 10.1080/17460441.2018.1542427
- Hwang DG, Choi YM, Jang J. 3D bioprinting-based vascularized tissue models mimicking tissue-specific architecture and pathophysiology for in vitro studies. Front Bioeng Biotechnol. 2021;9:685507. doi: 10.3389/fbioe.2021.685507
- Wu BX, Wu Z, Hou YY, et al. Application of three-dimensional (3D) bioprinting in anti-cancer therapy. Heliyon. 2023;9(10):e20475. doi: 10.1016/j.heliyon.2023.e20475
- Peng W, Datta P, Ayan B, Ozbolat V, Sosnoski D, Ozbolat IT. 3D bioprinting for drug discovery and development in pharmaceutics. Acta Biomat. 2017;57:26-46. doi: 10.1016/j.actbio.2017.05.025
- Jorfi M, D’Avanzo C, Kim DY, Irimia, D. Three-dimensional models of the human brain development and diseases. Adv Healthc Mater. 2018;7(1):10.1002/adhm.201700723. doi: 10.1002/adhm.201700723
- Edelman DB, Keefer EW. A cultural renaissance: in vitro cell biology embraces three-dimensional context. Exp Neurol. 2005;192(1):1-6. doi: 10.1016/j.expneurol.2004.10.005
- Zhao X, Bhattacharyya A. Human models are needed for studying human neurodevelopmental disorders. Am J Hum Genet. 2018;103(6):829-857. doi: 10.1016/j.ajhg.2018.10.009