Development of embedded bioprinting for fabricating zonally stratified articular cartilage
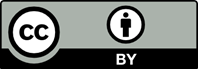
Embedded bioprinting enables direct deposition of bioinks in three dimensions inside a support bath with shear-thinning and self-healing capabilities, and it has been used to fabricate complex tissues and organs for several biomedical applications. In this study, a support bath comprising gelatin/alginate microparticles and oxidized alginate solution was developed and crosslinked in situ with carbonyl hydrazide-modified gelatin bioink via the Schiff base reaction. The numerical model of embedded printing was established to analyze the extrusion process and disturbance of the support bath. The process window (e.g., extrusion pressure, nozzle moving speed, nozzle size, and support bath composition) was established experimentally to ensure stable fiber formation. In addition, the compressive modulus of the printed construct has been reinforced due to the formation of interpenetrating polymer networks in the microparticles. Based on the process investigation, a zonally stratified artificial cartilage with a three-layered structure was designed: vertically printed fibers in the bottom, oblique fibers in the middle, and horizontally printed fibers in the superficial layer. The bioprinted cartilage supported cell survival, proliferation, and spreading, with the observed deposition of cartilage-specific proteins, offering a new strategy for developing tissue-engineered cartilage constructs with biological and histological relevance.
- Pap T, Korb-Pap A. Cartilage damage in osteoarthritis and rheumatoid arthritis--two unequal siblings. Nat Rev Rheumatol. 2015;11(10):606-615. doi: 10.1038/nrrheum.2015.95
- Farr J, Cole B, Dhawan A, et al. Clinical cartilage restoration: evolution and overview. Clin Orthop Relat Res. 2011;469(10):2696-2705. doi: 10.1007/s11999-010-1764-z
- Zeng Y, Wan Y, Yuan Z, et al. Healthcare-seeking behavior among Chinese older adults: patterns and predictive factors. Int J Environ Res Public Health. 2021; 18(6):2969. doi: 10.3390/ijerph18062969
- Harris JD, Siston RA, Pan X, et al. Autologous chondrocyte implantation: a systematic review. J Bone Joint Surg Am. 2010;92(12): 2220-2233. doi: 10.2106/JBJS.J.00049
- Makris EA, Gomoll AH, Malizos KN, et al. Repair and tissue engineering techniques for articular cartilage. Nat Rev Rheumatol. 2015;11(1):21-34. doi: 10.1038/nrrheum.2014.157
- Wu Y, Ayan B, Moncal KK, et al. Hybrid bioprinting of zonally stratified human articular cartilage using scaffold-free tissue strands as building blocks. Adv Healthc Mater. 2020;9(22):e2001657. doi: 10.1002/adhm.202001657
- Mardones R, Jofré CM, Minguell JJ. Cell therapy and tissue engineering approaches for cartilage repair and/or regeneration. Int J Stem Cells. 2015;8(1):48-53. doi: 10.15283/ijsc.2015.8.1.48
- Wu Y, Kennedy P, Bonazza N, et al. Three-dimensional bioprinting of articular cartilage: a systematic review. Cartilage. 2021;12(1):76-92. doi: 10.1177/1947603518809410
- Markstedt K, Mantas A, Tournier I, et al. 3D bioprinting human chondrocytes with nanocellulose-alginate bioink for cartilage tissue engineering applications. Biomacromolecules. 2015;16(5):1489-1496. doi: 10.1021/acs.biomac.5b00188
- Cui X, Breitenkamp K, Finn M, et al. Direct human cartilage repair using three-dimensional bioprinting technology. Tissue Eng Part A. 2012;18(11-12):1304-1312. doi: 10.1089/ten.TEA.2011.0543
- Zhu W, Cui H, Boualam B, et al. 3D bioprinting mesenchymal stem cell-laden construct with core-shell nanospheres for cartilage tissue engineering. Nanotechnology. 2018;29(18):185101. doi: 10.1088/1361-6528/aaafa1
- Ayan B, Wu Y, Karuppagounder V, et al. Aspiration-assisted bioprinting of the osteochondral interface. Sci Rep. 2020;10(1): 13148. doi: 10.1038/s41598-020-69960-6
- Ren X, Wang F, Chen C, et al. Engineering zonal cartilage through bioprinting collagen type II hydrogel constructs with biomimetic chondrocyte density gradient. BMC Musculoskelet Disord. 2016;17:1-10. doi: 10.1186/s12891-016-1130-8
- Tamaddon M, Blunn G, Tan R, et al. In vivo evaluation of additively manufactured multi-layered scaffold for the repair of large osteochondral defects. Bio-des Manuf. 2022;5(3):481-496. doi: 10.1007/s42242-021-00177-w
- Levato R, Visser J, Planell JA, et al. Biofabrication of tissue constructs by 3D bioprinting of cell-laden microcarriers. Biofabrication. 2014;6(3):035020. doi: 10.1088/1758-5082/6/3/035020
- Shim JH, Jang KM, Hahn SK, et al. Three-dimensional bioprinting of multilayered constructs containing human mesenchymal stromal cells for osteochondral tissue regeneration in the rabbit knee joint. Biofabrication. 2016;8(1):014102. doi: 10.1088/1758-5090/8/1/014102
- Lee A, Hudson A, Shiwarski D, et al. 3D bioprinting of collagen to rebuild components of the human heart. Science. 2019;365(6452):482-487. doi: 10.1126/science.aav9051
- Fang Y, Guo Y, Wu B, et al. Expanding embedded 3D bioprinting capability for engineering complex organs with freeform vascular networks. Adv Mater. 2023;35(22):e2205082. doi: 10.1002/adma.202205082
- Ren B, Song K, Sanikommu AR, et al. Study of sacrificial ink-assisted embedded printing for 3D perfusable channel creation for biomedical applications. Appl Phys Rev. 2022;9(1):011408. doi: 10.1063/5.0068329
- Kajtez J, Wesseler MF, Birtele M, et al. Embedded 3D printing in self‐healing annealable composites for precise patterning of functionally mature human neural constructs. Adv Sci (Weinh). 2022;9(25):e2201392. doi: 10.1002/advs.202201392
- Zhang S, Qi C, Zhang W, et al. In situ endothelialization of free-form 3d network of interconnected tubular channels via interfacial coacervation by aqueous-in-aqueous embedded bioprinting. Adv Mater. 2023;35(7): e2209263. doi: 10.1002/adma.202209263
- Chen Z, Huang C, Liu H, et al. 3D bioprinting of complex biological structures with tunable elastic modulus and porosity using freeform reversible embedding of suspended hydrogels. Bio-des Manuf. 2023;6(5):550-562. doi: 10.1007/s42242-023-00251-5
- Hinton TJ, Jallerat Q, Palchesko RN, et al., 2015, Three-dimensional printing of complex biological structures by freeform reversible embedding of suspended hydrogels. Sci Adv. 2015;1(9):e1500758. doi: 10.1126/sciadv.1500758
- Bhattacharjee T, Zehnder SM, Rowe KG, et al. Writing in the granular gel medium. Sci Adv. 2015;1(8):e1500655. doi: 10.1126/sciadv.1500655
- Hinton TJ, Hudson A, Pusch K, et al. 3D printing PDMS elastomer in a hydrophilic support bath via freeform reversible embedding. ACS Biomater Sci Eng. 2016;2(10):1781-1786. doi: 10.1021/acsbiomaterials.6b00170
- Yang J, He H, Li D, et al. Advanced strategies in the application of gelatin-based bioink for extrusion bioprinting. Bio-des Manuf. 2023;6(5):586-608. doi: 10.1007/s42242-023-00236-4
- Heo DN, Alioglu MA, Wu Y, et al. 3D bioprinting of carbohydrazide-modified gelatin into microparticle-suspended oxidized alginate for the fabrication of complex-shaped tissue constructs. ACS Appl Mater Interfaces. 2020;12(18):20295-20306. doi: 10.1021/acsami.0c05096
- Hozumi T, Kageyama T, Ohta S, et al. Injectable hydrogel with slow degradability composed of gelatin and hyaluronic acid cross-linked by Schiff ’s base formation. Biomacromolecules. 2018;19(2):288-297. doi: 10.1021/acs.biomac.7b01133
- Friedrich LM, Seppala JE. Simulated filament shapes in embedded 3D printing. Soft Matter. 2021;17(35): 8027-8046. doi: 10.1039/D1SM00731A
- Xu Y, Qi J, Zhou W, et al. Generation of ring-shaped human iPSC-derived functional heart microtissues in a Möbius strip configuration. Bio-des Manuf. 2022; 5(4):687-699. doi: 10.1007/s42242-022-00204-4
- Sophia Fox AJ, Bedi A, Rodeo SA. The basic science of articular cartilage: structure, composition, and function. Sports Health. 2009;1(6):461-468. doi: 10.1177/1941738109350438
- Mow VC, Ratcliffe A, Poole AR. Cartilage and diarthrodial joints as paradigms for hierarchical materials and structures. Biomaterials. 1992;13(2):67-97. doi: 10.1016/0142-9612(92)90001-5
- Kiani C, Chen L, Wu YJ, et al. Structure and function of aggrecan. Cell Res. 2002;12(1):19-32. doi: 10.1038/sj.cr.7290106
- Hua W, Mitchell K, Raymond L, et al. Fluid bath-assisted 3D printing for biomedical applications: from pre-to postprinting stages. ACS Biomater Sci Eng. 2021;7(10): 4736-4756. doi: 10.1021/acsbiomaterials.1c00910
- Tang G, Luo Z, Lian L, et al. Liquid-embedded (bio) printing of alginate-free, standalone, ultrafine, and ultrathin-walled cannular structures. Proc Natl Acad Sci U S A. 2023;120(7):e2206762120. doi: 10.1073/pnas.2206762120
- Wen C, Lu L, and Li X. Mechanically robust gelatin-A lginate IPN hydrogels by a combination of enzymatic and ionic crosslinking approaches. Macromol Mater Eng. 2014;299(4):504-513. doi: 10.1002/mame.201300274
- Chen S, Tan WS, Bin Juhari MA, et al. Freeform 3D printing of soft matters: recent advances in technology for biomedical engineering. Biomed Eng Lett. 2020;10:453-479. doi: 10.1007/s13534-020-00171-8
- Becker M, Gurian M, Schot M, et al. Aqueous two-phase enabled low viscosity 3d (LoV3D) bioprinting of living matter. Adv Sci (Weinh). 2023;10(8):2204609. doi: 10.1002/advs.202370046
- O’Bryan C S, Bhattacharjee T, Niemi SR, et al. Three-dimensional printing with sacrificial materials for soft matter manufacturing. MRS Bull. 2017;42(8):571-577. doi: 10.1557/mrs.2017.167
- Bakht SM, Gomez‐Florit M, Lamers T, et al. 3D bioprinting of miniaturized tissues embedded in self-assembled nanoparticle-based fibrillar platforms. Adv Funct Mater. 2021;31(46):2104245. doi: 10.1002/adfm.202104245
- Huo X, Zhang B, Han Q, et al. Numerical simulation and printability analysis of fused deposition modeling with dual-temperature control. Bio-des Manuf. 2023;6(2):174-188. doi: 10.1007/s42242-023-00239-1
- LeBlanc KJ, Niemi SR, Bennett AI, et al. Stability of high speed 3D printing in liquid-like solids. ACS Biomater Sci Eng. 2016;2(10):1796-1799. doi: 10.1021/acsbiomaterials.6b00184
- Li Q, Ma L, Gao Z, et al. Regulable supporting baths for embedded printing of soft biomaterials with variable stiffness. ACS Appl Mater Interfaces. 2022;14(37):41695-41711. doi: 10.1021/acsami.2c09221