Advancements in bioprinting for the treatment of hearing loss: A review
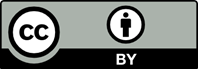
Hearing loss is a prevalent worldwide health concern, characterized by structural or functional abnormalities in any part of the ear resulting in varying degrees of auditory dysfunction. Despite significant progress, there exists a notable deficiency in reliable and safe therapeutic interventions for addressing hearing loss. Bioprinting, a novel method in the realm of advanced tissue engineering, exhibits significant potential in addressing ear-related ailments. This technology provides a wide range of repair mechanisms specifically designed to address the distinct anatomical and functional requirements of the outer ear, middle ear, and inner ear, potentially leading to advancements in the treatment of different types of hearing loss. Furthermore, bioprinting holds promise for producing tissue constructs that are both anatomically accurate and functionally suitable, potentially revolutionizing the current treatment landscape. Nevertheless, thorough research and development efforts are necessary to fully harness the capabilities of bioprinting and establish it as a widely accepted treatment option for hearing loss.
- Deafness and Hearing Loss; 2024. https://www.who.int/news-room/fact-sheets/detail/ deafness-and-hearing-loss.
- Cunningham LL, Tucci DL. Hearing loss in adults. N Engl J Med. 2017;377:2465-2473. doi: 10.1056/NEJMra1616601
- Nieman CL, Oh ES. Hearing loss. Ann Intern Med. 2020;173:ITC81-ITC96. doi: 10.7326/AITC202012010
- Zoccali F, Colizza A, Cialente F, et al. 3D printing in otolaryngology surgery: descriptive review of literature to define the state of the art. Healthcare (Basel). 2022; 11(1):108. doi: 10.3390/healthcare11010108
- Smith RJ, Bale JF, Jr., White KR. Sensorineural hearing loss in children. Lancet. 2005;365:879-890. doi: 10.1016/S0140-6736(05)71047-3
- Augustine R. Skin bioprinting: a novel approach for creating artificial skin from synthetic and natural building blocks. Prog Biomater. 2018;7:77-92. doi: 10.1007/s40204-018-0087-0
- Vijayavenkataraman S, Yan WC, Lu WF, Wang CH, Fuh JYH. 3D bioprinting of tissues and organs for regenerative medicine. Adv Drug Deliv Rev. 2018;132: 296-332. doi: 10.1016/j.addr.2018.07.004
- Carou-Senra P, Rodríguez-Pombo L, Awad A, Basit AW, Alvarez-Lorenzo C, Goyanes A. Inkjet printing of pharmaceuticals. Adv Mater. 2024;36:e2309164. doi: 10.1002/adma.202309164
- Ostrovidov S, Salehi S, Costantini M, et al. 3D bioprinting in skeletal muscle tissue engineering. Small. 2019;15(24):e1805530. doi: 10.1002/smll.201805530
- Matai I, Kaur G, Seyedsalehi A, McClinton A, Laurencin CT. Progress in 3D bioprinting technology for tissue/ organ regenerative engineering. Biomaterials. 2020; 226:119536. doi: 10.1016/j.biomaterials.2019.119536
- Chartrain NA, Williams CB, Whittington AR. A review on fabricating tissue scaffolds using vat photopolymerization. Acta Biomater. 2018;74:90-111. doi: 10.1016/j.actbio.2018.05.010
- Bezek LB, Chatham CA, Dillard DA, Williams CB. Mechanical properties of tissue-mimicking composites formed by material jetting additive manufacturing. J Mech Behav Biomed Mater. 2022;125:104938. doi: 10.1016/j.jmbbm.2021.104938
- Ng WL, Shkolnikov V. Optimizing cell deposition for inkjet-based bioprinting. Int J Bioprint. 2024;10(2):2135. doi: 10.36922/ijb.2135
- Boularaoui S, Al Hussein G, Khan KA. An overview of extrusion-based bioprinting with a focus on induced shear stress and its effect on cell viability. Bioprinting. 2020;20(24):20e00093/1-e00093/17. doi: 10.1016/j.bprint.2020.e00093
- Boularaoui S, Shanti A, Khan KA. Harnessing shear stress preconditioning to improve cell viability in 3D post-printed biostructures using extrusion bioprinting. Bioprinting. 2022;25:e00184/1-e00184/9. doi: 10.1016/j.bprint.2021.e00184
- Ng WL, Huang X, Shkolnikov V, Goh GL, Suntornnond R, Yeong WY. Controlling droplet impact velocity and droplet volume: key factors to achieving high cell viability in sub-nanoliter droplet-based bioprinting. Int J Bioprint. 2021;8(1):424. doi: 10.18063/ijb.v8i1.424
- Samavedi S, Whittington AR, Goldstein AS. Calcium phosphate ceramics in bone tissue engineering: a review of properties and their influence on cell behavior. Acta Biomater. 2013;9(9):8037-8045. doi: 10.1016/j.actbio.2013.06.014
- Suntornnond R, Ng WL, Huang X, Yeow CHE, Yeong WY. Improving printability of hydrogel-based bio-inks for thermal inkjet bioprinting applications via saponification and heat treatment processes. J Mater Chem B. 2022;10: 5989-6000. doi: 10.1039/d2tb00442a
- Ng WL, May WYY, Naing W. Society for Biomaterials Annual Meeting and Exposition 2017: Where Materials Become Medicine: Society for Biomaterials 40th Annual Meeting and Exposition, vol. 627. Minneapolis, MN, USA: Society for Biomaterials; 2017.
- Ng WL, Yeong WY, Naing MW. Polyvinylpyrrolidone-based bio-ink improves cell viability and homogeneity during drop-on-demand printing. Materials (Basel). 2017;10(2):190. doi: 10.3390/ma10020190
- Ng WL, Huang X, Shkolnikov V, et al. Polyvinylpyrrolidone-based bioink: influence of bioink properties on printing performance and cell proliferation during inkjet-based bioprinting. Bio-des Manuf. 2023;6(2):676-690. doi: 10.1007/s42242-023-00245-3
- Zhuang P, Ng WL, An J, Chua CK, Tan LP. Layer-by-layer ultraviolet assisted extrusion-based (UAE) bioprinting of hydrogel constructs with high aspect ratio for soft tissue engineering applications. PLoS One. 2019;14:e0216776. doi: 10.1371/journal.pone.0216776
- Ng WL, Goh MH, Yeong WY, Naing MW. Applying macromolecular crowding to 3D bioprinting: fabrication of 3D hierarchical porous collagen-based hydrogel constructs. Biomater Sci. 2018;6:562-574. doi: 10.1039/c7bm01015j
- Fedorovich NE, Haverslag RT, Dhert WJ, Alblas J. The role of endothelial progenitor cells in prevascularized bone tissue engineering: development of heterogeneous constructs. Tissue Eng Part A. 2010;16(7):2355-2367. doi: 10.1089/ten.TEA.2009.0603
- Mao, Q, Wang Y, Li Y, et al. Fabrication of liver microtissue with liver decellularized extracellular matrix (dECM) bioink by digital light processing (DLP) bioprinting. Mater Sci Eng C Mater Biol Appl. 2020; 109:110625. doi: 10.1016/j.msec.2020.110625
- Leberfinger AN, Ravnic DJ, Dhawan A, Ozbolat IT. Concise review: bioprinting of stem cells for transplantable tissue fabrication. Stem Cells Transl Med. 2017;6(10):1940-1948. doi: 10.1002/sctm.17-0148
- Sekar MP, Budharaju H, Zennifer A, et al. Current standards and ethical landscape of engineered tissues-3D bioprinting perspective. J Tissue Eng. 2021;12:20417314211027677. doi: 10.1177/20417314211027677
- Tashman JW, Shiwarski DJ, Feinberg AW. Development of a high-performance open-source 3D bioprinter. Sci Rep. 2022;12:22652. doi: 10.1038/s41598-022-26809-4
- Jeon B, Lee C, Kim M, Choi TH, Kim S, Kim S. Fabrication of three-dimensional scan-to-print ear model for microtia reconstruction. J Surg Res. 2016;206:490-497. doi: 10.1016/j.jss.2016.08.004
- Joo OY, Kim TH, Kim YS, et al. Fabrication of 3D-printed implant for two-stage ear reconstruction surgery and its clinical application. Yonsei Med J. 2023;64:291-296. doi: 10.3349/ymj.2022.0547
- Andrews J, Kopacz AA, Hohman MH. Ear microtia. In: StatPearls [Internet]. Treasure Island (FL): StatPearls Publishing; 2024.
- Romano JJ, Iliff NT, Manson PN. Use of Medpor porous polyethylene implants in 140 patients with facial fractures. J Craniofac Surg. 1993;4(3):142-147. doi: 10.1097/00001665-199307000-00007
- Ma Y, Lloyd MS. Systematic review of Medpor versus autologous ear reconstruction. J Craniofac Surg. 2022;33(2):602-606. doi: 10.1097/SCS.0000000000008130
- Zielinska D, Fisch P, Moehrlen U, et al. Combining bioengineered human skin with bioprinted cartilage for ear reconstruction. Sci Adv. 2023;9(40):eadh1890. doi: 10.1126/sciadv.adh1890
- Wang X, Neu CP, Pierce DM. Advances toward multiscale computational models of cartilage mechanics and mechanobiology. Curr Opin Biomed Eng. 2019;11:51-57.
- Xie, X, Wu S, Mou S, et al. Microtissue-based bioink as a chondrocyte microshelter for DLP bioprinting. Adv Healthc Mater. 2022;11(22):e2201877. doi: 10.1002/adhm.202201877
- Hirano N, Kusuhara H, Sueyoshi Y, et al. Ethanol treatment of nanoPGA/PCL composite scaffolds enhances human chondrocyte development in the cellular microenvironment of tissue-engineered auricle constructs. PLoS One. 2021;16(7):e0253149. doi: 10.1371/journal.pone.0253149
- He A, Xia H, Xiao K, et al. Cell yield, chondrogenic potential, and regenerated cartilage type of chondrocytes derived from ear, nasoseptal, and costal cartilage. J Tissue Eng Regen Med. 2018;12(4):1123-1132. doi: 10.1002/term.2613
- Cao T, Chang S, Wang Y, Wang B, Zhang Q. Review of 602 microtia reconstructions: revisions and specific recommendations for each subtype. Plast Reconstr Surg. 2021;148(2):307e-308e. doi: 10.1097/PRS.0000000000008399
- Wersenyi G, Scheper V, Spagnol S, Eixelberger T, Wittenberg T. Cost-effective 3D scanning and printing technologies for outer ear reconstruction: current status. Head Face Med. 2023;19:46. doi: 10.1186/s13005-023-00394-x
- Lee JS, Hong JM, Jung JW, et al. 3D printing of composite tissue with complex shape applied to ear regeneration. Biofabrication. 2014;6(2):024103. doi: 10.1088/1758-5082/6/2/024103
- Zhou G, Jiang H, Yin Z, et al. In vitro regeneration of patient-specific ear-shaped cartilage and its first clinical application for auricular reconstruction. EBioMedicine. 2018;28: 287-302. doi: 10.1016/j.ebiom.2018.01.011
43 Mannoor MS, Jiang Z, James T, et al. 3D printed bionic ears. Nano Lett. 2013;13(6):2634-2639. doi: 10.1021/nl4007744
- Chen Y, Zhang J, Liu X, et al. Noninvasive in vivo 3D bioprinting. Sci Adv. 2020;6(23):eaba7406. doi: 10.1126/sciadv.aba7406
- Della Volpe A, Ippolito V, Pastore V, et al. Use of a 3D reconstruction model in a patient with severe atresia auris for optimal placement of Bonebridge transcutaneous bone conduction implant. Eur Arch Otorhinolaryngol. 2021;278(9):3559-3564. doi: 10.1007/s00405-020-06528-0
- Hu H, Chen J, Li S, Xu T, Li Y. 3D printing technology and applied materials in eardrum regeneration. J Biomater Sci Polym Ed. 2023;34(7):950-985. doi: 10.1080/09205063.2022.2147350
- Maharajan N, Cho GW, Jang CH. Application of mesenchymal stem cell for tympanic membrane regeneration by tissue engineering approach. Int J Pediatr Otorhinolaryngol. 2020;133:109969. doi: 10.1016/j.ijporl.2020.109969
- Jang CH, Ahn S, Lee JW, Lee BH, Lee H, Kim G. Mesenchymal stem cell-laden hybrid scaffold for regenerating subacute tympanic membrane perforation. Mater Sci Eng C Mater Biol Appl. 2017;72:456-463. doi: 10.1016/j.msec.2016.11.094
- Kuo CY, Wilson E, Fuson A, et al. Repair of tympanic membrane perforations with customized bioprinted ear grafts using Chinchilla models. Tissue Eng Part A. 2018;24(5–6):527-535. doi: 10.1089/ten.TEA.2017.0246
- Jang CH, Kim W, Moon C, Kim G. Bioprinted collagen-based cell-laden scaffold with growth factors for tympanic membrane regeneration in chronic perforation model. IEEE Trans Nanobioscience. 2022;21(3):370-379. doi: 10.1109/TNB.2021.3085599
- Luers JC, Huttenbrink KB. Surgical anatomy and pathology of the middle ear. J Anat. 2016;228(2):338-353. doi: 10.1111/joa.12389
- Gu Y, Pigeot S, Ahrens L, et al. Toward 3D bioprinting of osseous tissue of predefined shape using single-matrix cell-bioink constructs. Adv Healthc Mater. 2003;12(9): e2202550. doi: 10.1002/adhm.202202550
- Forget A, Gianni-Barrera R, Uccelli A, et al. Mechanically defined microenvironment promotes stabilization of microvasculature, which correlates with the enrichment of a novel Piezo-1(+) population of circulating CD11b(+)/ CD115(+) monocytes. Adv Mater. 2019;31(21):e1808050. doi: 10.1002/adma.201808050
- Lethaus B, Poort L, Böckmann R, et al. Additive manufacturing for microvascular reconstruction of the mandible in 20 patients. J Craniomaxillofac Surg. 2012;40(1):43-46. doi: 10.1016/j.jcms.2011.01.007
- Li C, Yang M, Xie Y, et al. Application of the polystyrene model made by 3-D printing rapid prototyping technology for operation planning in revision lumbar discectomy. J Orthop Sci. 2015;20(3):475-480. doi: 10.1007/s00776-015-0706-8
- Heikkinen AK, Lähde S, Rissanen V, et al. Feasibility of 3D-printed middle ear prostheses in partial ossicular chain reconstruction. Int J Bioprint. 2023;9(4):727. doi: 10.18063/ijb.727
- Wood CB, Yawn R, Lowery AS, O’Connell BP, Haynes D, Wanna GB. Long-term hearing outcomes following total ossicular reconstruction with titanium prostheses. Otolaryngol Head Neck Surg. 2019;161(1);123-129. doi: 10.1177/0194599819831284
- Yung M. Long-term results of ossiculoplasty: reasons for surgical failure. Otol Neurotol. 2006;27(1):20-26. doi: 10.1097/01.mao.0000176173.94764.f5
- Kaftan H, Bohme A, Martin H. Geometric parameters of the ossicular chain as a function of its integrity: a micro- CT study in human temporal bones. Otol Neurotol. 2015;36(1):178-183. doi: 10.1097/MAO.0000000000000315
- Watson J, Hatamleh MM. Complete integration of technology for improved reproduction of auricular prostheses. J Prosthet Dent. 2014;111(5):430-436. doi: 10.1016/j.prosdent.2013.07.018
- Kozin ED, Remenschneider AK, Cheng S, Nakajima HH, Lee DJ. Three-dimensional printed prosthesis for repair of superior canal dehiscence. Otolaryngol Head Neck Surg. 2015;153(4):616-619. doi: 10.1177/0194599815592602
- Kuru I, Maier H, Muller M, Lenarz T, Lueth TC. A 3D-printed functioning anatomical human middle ear model. Hear Res. 2016;340:204-213. doi: 10.1016/j.heares.2015.12.025
- Hirsch JD, Vincent RL, Eisenman DJ. Surgical reconstruction of the ossicular chain with custom 3D printed ossicular prosthesis. 3D Print Med. 2017;3(1):7. doi: 10.1186/s41205-017-0015-2
- Dalchow CV, Grun D, Stupp HF. Reconstruction of the ossicular chain with titanium implants. Otolaryngol Head Neck Surg. 2001;125(6):628-630. doi: 10.1067/mhn.2001.120397
- Hillman TA, Shelton C. Ossicular chain reconstruction: titanium versus plastipore. Laryngoscope. 2003;113(10): 1731-1735. doi: 10.1097/00005537-200310000-00013
- He H, Li D, Lin Z, et al. Temperature-programmable and enzymatically solidifiable gelatin-based bioinks enable facile extrusion bioprinting. Biofabrication. 2020;12(4): 045003. doi: 10.1088/1758-5090/ab9906
- Wang J, Puel JL. Toward cochlear therapies. Physiol Rev. 2018;98(4):2477-2522. doi: 10.1152/physrev.00053.2017
- Xiao Y, Li D. The role of epigenetic modifications in sensory hair cell development, survival, and regulation. Front Cell Neurosci. 2023;17:1210279. doi: 10.3389/fncel.2023.1210279
- Schreiber BE, Agrup C, Haskard DO, Luxon LM. Sudden sensorineural hearing loss. Lancet. 2010;375(9721): 1203-1211. doi: 10.1016/S0140-6736(09)62071-7
- He Z, Ding Y, Xu X, et al. Stem cell-based therapies in hearing loss. Front Cell Dev Biol. 2021;9:730042. doi: 10.3389/fcell.2021.730042
- Li M, Mu Y, Cai H, Wu H, Ding Y. Application of new materials in auditory disease treatment. Front Cell Neurosci. 2021;15:831591. doi: 10.3389/fncel.2021.831591
- Liu C, Campbell SB, Li J, et al. High throughput omnidirectional printing of tubular microstructures from elastomeric polymers. Adv Healthc Mater. 2022;11(23):e2201346. doi: 10.1002/adhm.202201346
- Lei IM, Jiang C, Lei CL, et al. 3D printed biomimetic cochleae and machine learning co-modelling provides clinical informatics for cochlear implant patients. Nat Commun. 2021;12(1):6260. doi: 10.1038/s41467-021-26491-6
- Haobo Li, J L., Yin H. Organoids induce hair cell regeneration: 3D bio-printed inner ear organ of Corti. CNKI 2024;02:001282. doi: 10.27012/d.cnki.gdhuu.2023.001282
- Junming Zhang XL. Auditory cell injury to study the protective mechanism of consturction of organ of Corti organgoids. CNKI 2024;02:000415. doi: 10.27012/d.cnki.gdhuu.2023.000415.
- Jaffredo M, Duchamp O, Touya N, et al. Proof of concept of intracochlear drug administration by laser-assisted bioprinting in mice. Hear Res. 2023; 438:108880. doi: 10.1016/j.heares.2023.108880
- Xu X, Zhao J, Wang M, Wang L, Yang J. 3D printed polyvinyl alcohol tablets with multiple release profiles. Sci Rep. 2019;9:12487. doi: 10.1038/s41598-019-48921-8
- Rizzo ML, Turco S, Spina F, et al. 3D printing and 3D bioprinting technology in medicine: ethical and legal issues. Clin Ter. 2023;174(1):80-84. doi: 10.7417/CT.2023.2501
- Zhang L, Yang G, Johnson BN, Jia X. Three-dimensional (3D) printed scaffold and material selection for bone repair. Acta Biomater. 2019;84:16-33. doi: 10.1016/j.actbio.2018.11.039
- Sahranavard M, Sarkari S, Safavi S, Ghorbani F. Three-dimensional bio-printing of decellularized extracellular matrix-based bio-inks for cartilage regeneration: a systematic review. Biomater Transl. 2022;3(2):105-115. doi: 10.12336/biomatertransl.2022.02.004
- Karabulut H, Ulag S, Dalbayrak B, et al. A novel approach for the fabrication of 3D-printed dental membrane scaffolds including antimicrobial pomegranate extract. Pharmaceutics. 2023;15(3):737. doi: 10.3390/pharmaceutics15030737
- VanKoevering KK, Malloy KM. Emerging role of three-dimensional printing in simulation in otolaryngology. Otolaryngol Clin North Am. 2017;50(5):947-958. doi: 10.1016/j.otc.2017.05.006
- Tack P, Victor J, Gemmel P, Annemans L. 3D-printing techniques in a medical setting: a systematic literature review. Biomed Eng Online. 2016;15:115. doi: 10.1186/s12938-016-0236-4
- Lin HH, Lonic D, Lo LJ. 3D printing in orthognathic surgery – a literature review. J Formos Med Assoc. 2018;117(7): 547-558. doi: 10.1016/j.jfma.2018.01.008
- Da Cruz MJ, Francis HW. Face and content validation of a novel three-dimensional printed temporal bone for surgical skills development. J Laryngol Otol 2015; 129(suppl 3):S23-S29. doi: 10.1017/S0022215115001346
- Rose AS, Webster CE, Harrysson OL, et al. Pre-operative simulation of pediatric mastoid surgery with 3D-printed temporal bone models. Int J Pediatr Otorhinolaryngol. 2015;79(5): 740-744. doi: 10.1016/j.ijporl.2015.03.004
- Rose AS, Kimbell JS, Webster CE, et al. Multi-material 3D models for temporal bone surgical simulation. Ann Otol Rhinol Laryngol. 2015;124(7):528-536. doi: 10.1177/0003489415570937
- Ayan, B. et al. Aspiration-assisted bioprinting for precise positioning of biologics. Sci Adv. 2020;6(10):eaaw5111. doi: 10.1126/sciadv.aaw5111
- Dey M, Ozbolat IT. 3D bioprinting of cells, tissues and organs. Sci Rep. 2020;10(1):14023. doi: 10.1038/s41598-020-70086-y
- Pignataro L, Mantovani M, Torretta S, Felisati G, Sambataro G. ENT assessment in the integrated management of candidate for (maxillary) sinus lift. Acta Otorhinolaryngol Ital. 2008;28(3):110-119.
- Ammirati M, Ma J, Cheatham ML, et al. Drilling the posterior wall of the petrous pyramid: a microneurosurgical anatomical study. J Neurosurg. 1993;78(3):452-455. doi: 10.3171/jns.1993.78.3.0452
- Kemp P, Stralen JV, De Graaf P, et al. Cone-beam CT compared to multi-slice CT for the diagnostic analysis of conductive hearing loss: a feasibility study. J Int Adv Otol. 2020;16(2):222-226. doi: 10.5152/iao.2020.5883
- Emerman AB, Zhang ZR, Chakrabarti O, Hegde RS. Compartment-restricted biotinylation reveals novel features of prion protein metabolism in vivo. Mol Biol Cell. 2010;21(24):4325-4337. doi: 10.1091/mbc.E10-09-0742
- McMillan A, McMillan N, Gupta N, Kanotra SP, Salem AK. 3D bioprinting in otolaryngology: a review. Adv Healthc Mater. 2023;12(19): e2203268. doi: 10.1002/adhm.202203268
- Dodziuk H. Applications of 3D printing in healthcare. Kardiochir Torakochirurgia Pol. 2016;13(3):283-293. doi: 10.5114/kitp.2016.62625
- Johansson M, Asp F, Berninger E. Children with congenital unilateral sensorineural hearing loss: effects of late hearing aid amplification-a pilot study. Ear Hear. 2020;41:55-66. doi: 10.1097/AUD.0000000000000730
- Johnstone PM, Yeager KR, Noss E. Spatial hearing in a child with auditory neuropathy spectrum disorder and bilateral cochlear implants. Int J Audiol. 2013;52(6):400-408. doi: 10.3109/14992027.2013.779755
- Jorgensen AM, Yoo JJ, Atala A. Solid organ bioprinting: strategies to achieve organ function. Chem Rev. 2020;120(19):11093-11127. doi: 10.1021/acs.chemrev.0c00145
- Naghieh S, Lindberg G, Tamaddon M, Liu C. Biofabrication strategies for musculoskeletal disorders: evolution towards clinical applications. Bioengineering (Basel). 2021; 8(9):123. doi: 10.3390/bioengineering8090123
- Langridge B, Griffin M, Butler PE. Regenerative medicine for skeletal muscle loss: a review of current tissue engineering approaches. J Mater Sci Mater Med. 2021;32(1):15. doi: 10.1007/s10856-020-06476-5
- Shah Mohammadi M, Buchen JT, Pasquina PF, et al. Critical considerations for regeneration of vascularized composite tissues. Tissue Eng Part B Rev. 2021;27(4):366-381. doi: 10.1089/ten.TEB.2020.0223
- Jain P, Kathuria H, Dubey N. Advances in 3D bioprinting of tissues/organs for regenerative medicine and in-vitro models. Biomaterials. 2022;287:121639. doi: 10.1016/j.biomaterials.2022.121639
- Goddard E, Dodds S. Ethics and policy for bioprinting. Methods Mol Biol. 2020;2140:43-64. doi: 10.1007/978-1-0716-0520-2_4
- Ivanovski S, Breik O, Carluccio D, et al. 3D printing for bone regeneration: challenges and opportunities for achieving predictability. Periodontol. 2000;93(1):358-384. doi: 10.1111/prd.12525
- Merlo A, Mokkapati V, Pandit S, Mijakovic I. Boron nitride nanomaterials: biocompatibility and bio-applications. Biomater Sci. 2018;6(9):2298-2311. doi: 10.1039/c8bm00516h
- Kus-Liskiewicz M, Fickers P, Ben Tahar I. Biocompatibility and cytotoxicity of gold nanoparticles: recent advances in methodologies and regulations. Int J Mol Sci. 2021;22(20):10952. doi: 10.3390/ijms222010952
- Adabi M, Naghibzadeh M, Adabi M, et al. Biocompatibility and nanostructured materials: applications in nanomedicine. Artif Cells Nanomed Biotechnol. 2017;45(4):833-842. doi: 10.1080/21691401.2016.1178134
- Naz S, Gul A, Zia M, Javed R. Synthesis, biomedical applications, and toxicity of CuO nanoparticles. Appl Microbiol Biotechnol. 2023;107(4): 1039-1061. doi: 10.1007/s00253-023-12364-z
- Przygoda RT. Safety assessment and global regulatory requirements for genetic toxicity evaluations of medical devices. Environ Mol Mutagen. 2017; 58(5):375-379. doi: 10.1002/em.22102
- Ali Zahid A, Chakraborty A, Shamiya Y, Ravi SP, Paul A. Leveraging the advancements in functional biomaterials and scaffold fabrication technologies for chronic wound healing applications. Mater Horiz. 2022;9(7):1850-1865. doi: 10.1039/d2mh00115b
- Delalat B, Harding F, Gundsambuu B, et al. 3D printed lattices as an activation and expansion platform for T cell therapy. Biomaterials. 2017;140:58-68. doi: 10.1016/j.biomaterials.2017.05.009
- Lindner N, Blaeser A. Scalable biofabrication: a perspective on the current state and future potentials of process automation in 3D-bioprinting applications. Front Bioeng Biotechnol. 2022;10:855042. doi: 10.3389/fbioe.2022.855042
- Ng WL, Lee JM, Zhou M, et al. Vat polymerization-based bioprinting-process, materials, applications and regulatory challenges. Biofabrication. 2020;12(2):022001. doi: 10.1088/1758-5090/ab6034
- Chan WW, Yu F, Le QB, et al. Towards biomanufacturing of cell-derived matrices. Int J Mol Sci. 2021;22(21):11929. doi: 10.3390/ijms222111929
- Murphy SV, De Coppi P, Atala A. Opportunities and challenges of translational 3D bioprinting. Nat Biomed Eng. 2020;4:370-380. doi: 10.1038/s41551-019-0471-7
- Bai J, Ge G, Wang Q, et al. Engineering stem cell recruitment and osteoinduction via bioadhesive molecular mimics to improve osteoporotic bone-implant integration. Research (Wash D C). 2022;2022:9823784. doi: 10.34133/2022/9823784
- Chen S, Wang H, Liu D, et al. Early osteoimmunomodulation by mucin hydrogels augments the healing and revascularization of rat critical-size calvarial bone defects. Bioact Mater. 2023;25:176-188. doi: 10.1016/j.bioactmat.2023.01.022
- Maruyama M, Rhee C, Utsunomiya T, et al. Modulation of the inflammatory response and bone healing. Front Endocrinol (Lausanne). 2020;11:386. doi: 10.3389/fendo.2020.00386
- Rahmati M, Stötzel S, Khassawna TE, et al. Early osteoimmunomodulatory effects of magnesium-calcium-zinc alloys. J Tissue Eng. 2021;12:20417314211047100. doi: 10.1177/20417314211047100
120 Wang M, Yu Y, Dai K, et al. Improved osteogenesis and angiogenesis of magnesium-doped calcium phosphate cement via macrophage immunomodulation. Biomater Sci. 2016;4(11):1574-1583. doi: 10.1039/c6bm00290k
- Zhou Y, Liao S, Chu Y, et al. An injectable bioink with rapid prototyping in the air and in-situmild polymerization for 3D bioprinting. Biofabrication. 2021;13(4). doi: 10.1088/1758-5090/ac23e4
- Park TY, Yang YJ, Ha DH, Cho DW, Cha HJ. Marine-derived natural polymer-based bioprinting ink for biocompatible, durable, and controllable 3D constructs. Biofabrication. 2019;11:035001. doi: 10.1088/1758-5090/ab0c6f
- Sun Y, Yu K, Nie J, et al. Modeling the printability of photocuring and strength adjustable hydrogel bioink during projection-based 3D bioprinting. Biofabrication. 2021;13(3). doi: 10.1088/1758-5090/aba413
- Singh YP, Bandyopadhyay A, Mandal BB. 3D bioprinting using cross-linker-free silk-gelatin bioink for cartilage tissue engineering. ACS Appl Mater Interfaces. 2019:11:33684-33696. doi: 10.1021/acsami.9b11644
- Lee M, Bae K, Levinson C, Zenobi-Wong M. Nanocomposite bioink exploits dynamic covalent bonds between nanoparticles and polysaccharides for precision bioprinting. Biofabrication. 2020;12: 025025. doi: 10.1088/1758-5090/ab782d
- Wei Y, Li L, Xie C, et al. Current status of auricular reconstruction strategy development. J Craniofac Surg. 2023; 10-1097. doi: 10.1097/SCS.0000000000009908.
- Ikeda AK, Bhrany AD, Sie KCY, Bly RA. Management of patients with unilateral microtia and aural atresia: recent advances and updates. Curr Opin Otolaryngol Head Neck Surg. 2021;29(6):526-533. doi: 10.1097/MOO.0000000000000758
- Kim B, Kim J, Lee S. Unleashing the power of undifferentiated induced pluripotent stem cell bioprinting: current progress and future prospects. Int J Stem Cells. 2024;17(1):38-50. doi: 10.15283/ijsc23146
- Cho S, Lee C, Skylar-Scott MA, Heilshorn SC, Wu JC. Reconstructing the heart using iPSCs: engineering strategies and applications. J Mol Cell Cardiol. 2021;157:56-65. doi: 10.1016/j.yjmcc.2021.04.006
- Gu Q, Tomaskovic-Crook E, Wallace GG, Crook JM. 3D bioprinting human induced pluripotent stem cell constructs for in situ cell proliferation and successive multilineage differentiation. Adv Healthc Mater. 2017;6(17):1700175. doi: 10.1002/adhm.201700175
- Sullivan MA, Sullivan MA, Lane S, Volkerling A, et al. Three-dimensional bioprinting of stem cell-derived central nervous system cells enables astrocyte growth, vasculogenesis, and enhances neural differentiation/function. Biotechnol Bioeng. 2023;120(10):3079-3091. doi: 10.1002/bit.28470
- Prominski A, Li P, Miao BA, Tian B. Nanoenabled bioelectrical modulation. Acc Mater Res. 2021;2(10): 895-906. doi: 10.1021/accountsmr.1c00132
- Pesantez Torres F, Tokranova N, Amodeo E, et al. Interfacing neural cells with typical microelectronics materials for future manufacturing. Biosens Bioelectron. 2023;242: 115749. doi: 10.1016/j.bios.2023.115749
- Du X, Wei D, Huang L, Zhu M, Zhang Y, Zhu Y. 3D printing of mesoporous bioactive glass/silk fibroin composite scaffolds for bone tissue engineering. Mater Sci Eng C Mater Biol Appl. 2019:103;109731. doi: 10.1016/j.msec.2019.05.016
- Chen H, Xue H, Zeng H, Dai M, Tang C, Liu L. 3D printed scaffolds based on hyaluronic acid bioinks for tissue engineering: a review. Biomater Res. 2023;27(1):137. doi: 10.1186/s40824-023-00460-0
- Miri AK, Mostafavi E, Khorsandi D, Hu SK, Malpica M, Khademhosseini A. Bioprinters for organs-on-chips. Biofabrication. 2019;11(4):042002. doi: 10.1088/1758-5090/ab2798
- Bosmans C, Ginés Rodriguez N, Karperien M, et al. Towards single-cell bioprinting: micropatterning tools for organ-on-chip development. Trends Biotechnol. 2024;42(6): 739-759. doi: 10.1016/j.tibtech.2023.11.014
- Vera D, García-Díaz M, Torras N, et al. A 3D bioprinted hydrogel gut-on-chip with integrated electrodes for transepithelial electrical resistance (TEER) measurements. Biofabrication. 2024;16(3):035008. doi: 10.1088/1758-5090/ad3aa4
- Tolabi H, Davari N, Khajehmohammadi M, et al. Progress of microfluidic hydrogel-based scaffolds and organ-on-chips for the cartilage tissue engineering. Adv Mater. 2023;35:e2208852. doi: 10.1002/adma.202208852
- Wei X, Zhou W, Tang Z, et al. Magnesium surface-activated 3D printed porous PEEK scaffolds for in vivo osseointegration by promoting angiogenesis and osteogenesis. Bioact Mater. 2023;20:16-28. doi: 10.1016/j.bioactmat.2022.05.011
- Arakawa CK, Badeau BA, Zheng Y, DeForest CA. Multicellular vascularized engineered tissues through user-programmable biomaterial photodegradation. Adv Mater. 2017;29(37):1703156. doi: 10.1002/adma.201703156
- Madl CM, Heilshorn SC, Blau HM. Bioengineering strategies to accelerate stem cell therapeutics. Nature. 2018;557(7705):335-342. doi: 10.1038/s41586-018-0089-z
- Yang J, Yang K, Man W, et al. 3D bio-printed living nerve-like fibers refine the ecological niche for long-distance spinal cord injury regeneration. Bioact Mater. 2023;25:160-175. doi: 10.1016/j.bioactmat.2023.01.023
- Kamperman T, Karperien M, Le Gac S, Leijten J. Single-cell microgels: technology, challenges, and applications. Trends Biotechnol. 2018;36(8):850-865. doi: 10.1016/j.tibtech.2018.03.001
- Malki M, Fleischer S, Shapira A, Dvir T. Gold nanorod-based engineered cardiac patch for suture-free engraftment by near IR. Nano Lett. 2018;18(7):4069-4073. doi: 10.1021/acs.nanolett.7b04924
- Bhamare N, Tardalkar K, Parulekar P, Khadilkar A, Joshi M. 3D printing of human ear pinna using cartilage specific ink. Biomed Mater. 2021;16(5):055008. doi: 10.1088/1748-605X/ac15b0.
- Lai J, Liu Y, Lu G, et al. 4D bioprinting of programmed dynamic tissues. Bioact Mater. 2024;37:348-377. doi: 10.1016/j.bioactmat.2024.03.033
- Zhou W, Qiao Z, Zare EN, et al. 4D-printed dynamic materials in biomedical applications: chemistry, challenges, and their future perspectives in the clinical sector. J Med Chem. 2020;63(15):8003-8024. doi: 10.1021/acs.jmedchem.9b02115
- Yarali E, Mirzaali MJ, Ghalayaniesfahani A, et al. 4D printing for biomedical applications. Adv Mater. 2024;4:2402301. doi: 10.1002/adma.202402301
- Chen A, Wang W, Mao Z, He Y. Multimaterial 3D and 4D bioprinting of heterogenous constructs for tissue engineering. Adv Mater. 2023;9:2307686. doi: 10.1002/adma.202307686
- Miri AK, Khalilpour A, Cecen B, Maharjan S, Shin SR, Khademhosseini A. Multiscale bioprinting of vascularized models. Biomaterials. 2019;198:204-216. doi: 10.1016/j.biomaterials.2018.08.006
- Kirillova A, Maxson R, Stoychev G, Gomillion CT, Ionov L. 4D biofabrication using shape-morphing hydrogels. Adv Mater. 2017;29(46). doi: 10.1002/adma.201703443
- Neumann M, di Marco G, Iudin D, et al. Stimuli-responsive hydrogels: the dynamic smart biomaterials of tomorrow. Macromolecules. 2023;56(21):8377-8392. doi: 10.1021/acs.macromol.3c00967
- Lui YS, Sow WT, Tan LP, Wu Y, Lai Y, Li H. 4D printing and stimuli-responsive materials in biomedical aspects. Acta Biomater. 2019;92:19-36. doi: 10.1016/j.actbio.2019.05.005
- Keshavarz M, Jahanshahi M, Hasany M, et al. Smart alginate inks for tissue engineering applications. Mater Today Bio. 2023;23:100829. doi: 10.1016/j.mtbio.2023.100829
- Tournier P, Saint-Pé G, Lagneau N, et al. Clickable dynamic bioinks enable post-printing modifications of construct composition and mechanical properties controlled over time and space. Adv Sci (Weinh). 2023;10(30):e2300055. doi: 10.1002/advs.202300055