Bioprinting native-like 3D micro breast cancer tissues utilizing existing cancer cell lines
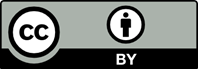
3D cancer cell models provide a more accurate representation of in vivo conditions than traditional 2D cultures. Many cancer cell lines, while stable and extensively characterized in 2D environments, often underperform compared to primary cells in 3D models due to the inherent resource constraints and variability of the latter. To bridge this gap and harness the full potential of established cancer cell lines, we adopted the innovative direct volumetric drop-on-demand (DVDOD) bioprinting methodology that we have developed previously, leading to the inception of printed micro-cancer tissues (PMCaTs). Our method, notable for its bioink droplet scattering technique, enables the generation of intricate features within a droplet, allowing for the creation of typical architectures of breast cancer tissue. We created PMCaTs that captured the essence of micro breast cancer tissues, from native-like ductal structures and cancer nests to the intricate cancer microenvironment. This encompasses elements like cancer-associated fibroblasts, detailed microvasculature, and regions marked by both intensive proliferation and hypoxia. These bioprinted models demonstrate long-term viability and are instrumental for diverse research areas—from exploring cancer growth dynamics and hypoxia-induced behaviors to investigating the nuances of microvasculature, drug penetration capabilities, immune responses, metastatic trends, and clinical drug response predictions. Ultimately, our groundbreaking DVDOD bioprinting technique holds the promise of reshaping the landscape of cancer research, introducing advanced in vitro models poised to transform therapeutic exploration.
- Hidalgo M, Amant F, Biankin AV, et al. Patient-derived xenograft models: an emerging platform for translational cancer research. Cancer Discov. 2014;4(9):998–1013. doi: 10.1158/2159-8290.CD-14-0001
- Langhans SA. Three-dimensional in vitro cell culture models in drug discovery and drug repositioning. Front Pharmacol. 2018;9:6. doi: 10.3389/fphar.2018.00006
- Nusinow DP, Szpyt J, Ghandi M, et al. Quantitative proteomics of the cancer cell line encyclopedia. Cell. 2020;180(2):387–402.e16. doi: 10.1016/j.cell.2019.12.023
- Cancer Therapeutics Response Portal. https://portals. broadinstitute.org/ctrp.v2.1/. Accessed August 13, 2023.
- Dai X, Cheng H, Bai Z, Li J. Breast cancer cell line classification and its relevance with breast tumor subtyping. J Cancer. 2017;8(16):3131–3141. doi: 10.7150/jca.18457
- Li Y, Kilian KA. Bridging the gap: from 2D cell culture to 3D microengineered extracellular matrices. Adv Healthc Mater. 2015;4(18):2780–2796.
- Egeblad M, Nakasone ES, Werb Z. Tumors as organs: complex tissues that interface with the entire organism. Dev Cell. 2010;18(6):884–901. doi: 10.1016/j.devcel.2010.05.012
- Sachs N, Clevers H. Organoid cultures for the analysis of cancer phenotypes. Curr Opin Genet Dev. 2014;24:68–73. doi: 10.1016/j.gde.2013.11.012
- Clevers H. Modeling development and disease with organoids. Cell. 2016;165(7):1586–1597. doi: 10.1016/j.cell.2016.05.082
- Ramesh S, Harrysson OLA, Rao PK, et al. Extrusion bioprinting: recent progress, challenges, and future opportunities. Bioprinting. 2021;21:e00116. doi: 10.1016/j.bprint.2020.e00116
- Chartrain NA, Williams CB, Whittington AR. A review on fabricating tissue scaffolds using vat photopolymerization. Acta Biomater. 2018;74:90–111. doi: 10.1016/j.actbio.2018.05.010
- Ng WL, Shkolnikov V. Optimizing cell deposition for inkjet-based bioprinting. IJB. 2024;0(0):2135. doi: 10.36922/ijb.2135
- Grottkau BE, Hui Z, Pang Y. A novel 3D bioprinter using direct-volumetric drop-on-demand technology for fabricating micro-tissues and drug-delivery. IJMS. 2020;21(10):3482. doi: 10.3390/ijms21103482
- Grottkau BE, Hui Z, Pang Y. Cellular patterning alone using bioprinting regenerates articular cartilage through native-like cartilagenesis. Research Square. 2023. doi: 10.21203/rs.3.rs-3380357/v1
- Bashar MA, Begam N. Breast cancer surpasses lung cancer as the most commonly diagnosed cancer worldwide. Indian J Cancer. 2022;59(3):438–439. doi: 10.4103/ijc.IJC_83_21
- Arnold M, Morgan E, Rumgay H, et al. Current and future burden of breast cancer: Global statistics for 2020 and 2040. Breast. 2022;66:15–23. doi: 10.1016/j.breast.2022.08.010
- Pang Y, Ucuzian AA, Matsumura A, et al. The temporal and spatial dynamics of microscale collagen scaffold remodeling by smooth muscle cells. Biomaterials. 2009;30(11): 2023–2031. doi: 10.1016/j.biomaterials.2008.12.064
- Chen Y, McAndrews KM, Kalluri R. Clinical and therapeutic relevance of cancer-associated fibroblasts. Nat Rev Clin Oncol. 2021;18(12):792–804. doi: 10.1038/s41571-021-00546-5
- Weiss L, Orr FW, Honn KV. Interactions of cancer cells with the microvasculature during metastasis. FASEB J. 1988;2(1):12–21. doi: 10.1096/fasebj.2.1.3275560
- Strzyz P. Cancer biology: hypoxia as an off switch for gene expression. Nat Rev Mol Cell Biol. 2016;17(10):610. doi: 10.1038/nrm.2016.119
- Koshiji M, Kageyama Y, Pete EA, Horikawa I, Barrett JC, Huang LE. HIF-1α induces cell cycle arrest by functionally counteracting Myc. EMBO J. 2004;23(9):1949–1956. doi: 10.1038/sj.emboj.7600196
- Hubbi ME, Kshitiz, Gilkes DM, et al. A Nontranscriptional Role for HIF-1α as a Direct Inhibitor of DNA Replication. Sci Signal. 2013;6(262):ra10. doi: 10.1126/scisignal.2003417
- Zhang Y, Zhang H, Wang M, et al. Hypoxia in breast cancer—scientific translation to therapeutic and diagnostic clinical applications. Front Oncol. 2021;11:652266. doi: 10.3389/fonc.2021.652266
- Chen X, Song E. Turning foes to friends: targeting cancer-associated fibroblasts. Nat Rev Drug Discov. 2019;18(2): 99–115. doi: 10.1038/s41573-018-0004-1
- Pepin F, Bertos N, Laferrière J, et al. Gene-expression profiling of microdissected breast cancer microvasculature identifies distinct tumor vascular subtypes. Breast Cancer Res. 2012;14(4):R120. doi: 10.1186/bcr3246
- Frentzas S, Simoneau E, Bridgeman VL, et al. Vessel co-option mediates resistance to anti-angiogenic therapy in liver metastases. Nat Med. 2016;22(11):1294–1302. doi: 10.1038/nm.4197
- Jain RK. Lessons from multidisciplinary translational trials on anti-angiogenic therapy of cancer. Nat Rev Cancer. 2008;8(4):309–316. doi: 10.1038/nrc2346
- Yu M, Tannock IF. Targeting tumor architecture to favor drug penetration: a new weapon to combat chemoresistance in pancreatic cancer? Cancer Cell. 2012;21(3):327–329. doi: 10.1016/j.ccr.2012.03.002
- Wang C, Chen S, Wang Y, et al. Lipase-triggered water-responsive “Pandora’s Box” for cancer therapy: toward induced neighboring effect and enhanced drug penetration. Adv Mater. 2018;30(14):e1706407. doi: 10.1002/adma.201706407
- Di Cosimo S. Advancing immunotherapy for early-stage triple-negative breast cancer. Lancet. 2020;396(10257):1046–1048. doi: 10.1016/S0140-6736(20)31962-0
- Riley RS, June CH, Langer R, Mitchell MJ. Delivery technologies for cancer immunotherapy. Nat Rev Drug Discov. 2019;18(3):175–196. doi: 10.1038/s41573-018-0006-z
- Becker JC, Andersen MH, Schrama D, Thor Straten P. Immune-suppressive properties of the tumor microenvironment. Cancer Immunol Immunother. 2013;62(7):1137–1148. doi: 10.1007/s00262-013-1434-6
- Wirtz D, Konstantopoulos K, Searson PC. The physics of cancer: the role of physical interactions and mechanical forces in metastasis. Nat Rev Cancer. 2011;11(7):512–522. doi: 10.1038/nrc3080
- Murphy PM. Chemokines and the molecular basis of cancer metastasis. N Engl J Med. 2001;345(11):833–835. doi: 10.1056/NEJM200109133451113
- Zardavas D, Maetens M, Irrthum A, et al. The AURORA initiative for metastatic breast cancer. Br J Cancer. 2014;111(10):1881–1887. doi: 10.1038/bjc.2014.341
- Haddad TC, Suman VJ, D’Assoro AB, et al. Evaluation of alisertib alone or combined with fulvestrant in patients with endocrine-resistant advanced breast cancer: the Phase 2 TBCRC041 randomized clinical trial. JAMA Oncol. 2023;9(6):815–824. doi: 10.1001/jamaoncol.2022.7949
- Melichar B, Adenis A, Lockhart AC, et al. Safety and activity of alisertib, an investigational aurora kinase A inhibitor, in patients with breast cancer, small-cell lung cancer, non-small-cell lung cancer, head and neck squamous-cell carcinoma, and gastro-oesophageal adenocarcinoma: a five-arm phase 2 study. Lancet Oncol. 2015;16(4): 395–405. doi: 10.1016/S1470-2045(15)70051-3
- Li JP, Yang YX, Liu QL, et al. The investigational Aurora kinase A inhibitor alisertib (MLN8237) induces cell cycle G2/M arrest, apoptosis, and autophagy via p38 MAPK and Akt/mTOR signaling pathways in human breast cancer cells. Drug Des Devel Ther. 2015;9:1627–1652. doi: 10.2147/DDDT.S75378