3D-bioprinted bone for regenerative medicine: Current concepts and future perspectives
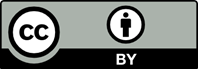
The shortages in human tissue and organ donors have made clinical therapy relatively challenging. Therefore, research has been initiated over the last decades to develop artificial tissues and organs, particularly from cell and tissue cultures. Three-dimensional (3D) bioprinting is a recent technology capable of building structures for implantation, and these constructs closely resemble native tissues, such as skin, liver, connective tissues, and supportive tissues (bone and cartilage). In this review, we briefly introduce the structure, function, and development of bone tissues, followed by a detailed discussion on 3D bioprinting techniques, materials, and their recent advancements for clinical applications.
- Oryan A, Alidadi S, Moshiri A, Maffulli N. Bone regenerative medicine: classic options, novel strategies, and future directions. J Orthop Surg Res. 2014;9(1):18. doi: 10.1186/1749-799X-9-18
- Maresca JA, DeMel DC, Wagner GA, Haase C, Geibel JP. Three-dimensional bioprinting applications for bone tissue engineering. Cells. 2023;12(9). doi: 10.3390/cells12091230
- Masaeli R, Zandsalimi K, Rasoulianboroujeni M, Tayebi L. Challenges in three-dimensional printing of bone substitutes. Tissue Eng Part B Rev. 2019;25(5):387-397. doi: 10.1089/ten.TEB.2018.0381
- Mirkhalaf M, Men Y, Wang R, No Y, Zreiqat H. Personalized 3D printed bone scaffolds: a review. Acta Biomater. 2023;156:110-124. doi: 10.1016/j.actbio.2022.04.014
- Do AV, Khorsand B, Geary SM, Salem AK. 3D printing of scaffolds for tissue regeneration applications. Adv Healthc Mater. 2015;4(12):1742-1762. doi: 10.1002/adhm.201500168
- Jariwala SH, Lewis GS, Bushman ZJ, Adair JH, Donahue HJ. 3D printing of personalized artificial bone scaffolds. 3D Print Addit Manuf. 2015;2(2):56-64. doi: 10.1089/3dp.2015.0001
- Stanco D, Urban P, Tirendi S, Ciardelli G, Barrero J. 3D bioprinting for orthopaedic applications: current advances, challenges and regulatory considerations. Bioprinting. 2020;20:None. doi: 10.1016/j.bprint.2020.e00103
- Wang X, Wang Y, Gou W, Lu Q, Peng J, Lu S. Role of mesenchymal stem cells in bone regeneration and fracture repair: a review. Int Orthop. 2013;37(12):2491-2498. doi: 10.1007/s00264-013-2059-2
- Florencio-Silva R, Sasso GR, Sasso-Cerri E, Simoes MJ, Cerri PS. Biology of bone tissue: structure, function, and factors that influence bone cells. Biomed Res Int. 2015;2015:421746. doi: 10.1155/2015/421746
- Sander PM, Klein N, Stein K, Wings O. Sauropod bone histology and its implications for sauropod biology. In: Klein N, Remes K, Sander M, Gee C, eds. Biology of the Sauropod Dinosaurs: Understanding the Life of Giants. Indiana University Press; 2011:276-302.
- Gerhardt LC, Boccaccini AR. Bioactive glass and glass-ceramic scaffolds for bone tissue engineering. Materials (Basel). 2010;3(7):3867-3910. doi: 10.3390/ma3073867
- Datta HK, Ng WF, Walker JA, Tuck SP, Varanasi SS. The cell biology of bone metabolism. J Clin Pathol. 2008;61(5):577-587. doi: 10.1136/jcp.2007.048868
- Lin X, Patil S, Gao YG, Qian A. The bone extracellular matrix in bone formation and regeneration. Front Pharmacol. 2020; 11:757. doi: 10.3389/fphar.2020.00757
- Kirby DJ, Young MF. Isolation, production, and analysis of small leucine-rich proteoglycans in bone. Methods Cell Biol. 2018;143:281-296. doi: 10.1016/bs.mcb.2017.08.016
- Nikitovic D, Aggelidakis J, Young MF, Iozzo RV, Karamanos NK, Tzanakakis GN. The biology of small leucine-rich proteoglycans in bone pathophysiology. J Biol Chem. 2012;287(41):33926-33933. doi: 10.1074/jbc.R112.379602
- Burnier JP, Borowski M, Furie BC, Furie B. Gamma-carboxyglutamic acid. Mol Cell Biochem. 1981;39:191-207. doi: 10.1007/BF00232574
- Wen L, Chen J, Duan L, Li S. Vitamin K‑dependent proteins involved in bone and cardiovascular health (Review). Mol Med Rep. 2018;18(1):3-15. doi: 10.3892/mmr.2018.8940
- Berendsen AD, Olsen BR. Bone development. Bone. 2015;80:14-18. doi: 10.1016/j.bone.2015.04.035
- Salhotra A, Shah HN, Levi B, Longaker MT. Mechanisms of bone development and repair. Nat Rev Mol Cell Biol. 2020;21(11):696-711. doi: 10.1038/s41580-020-00279-w
- Walmsley GG, Ransom RC, Zielins ER, et al. Stem cells in bone regeneration. Stem Cell Rev Rep. 2016;12(5):524-529. doi: 10.1007/s12015-016-9665-5
- Kolios G, Moodley Y. Introduction to stem cells and regenerative medicine. Respiration. 2013;85(1):3-10. doi: 10.1159/000345615
- Vereb Z, Mazlo A, Szabo A, et al. Vessel wall-derived mesenchymal stromal cells share similar differentiation potential and immunomodulatory properties with bone marrow-derived stromal cells. Stem Cells Int. 2020;2020:8847038. doi: 10.1155/2020/8847038
- Han Y, Li X, Zhang Y, Han Y, Chang F, Ding J. Mesenchymal stem cells for regenerative medicine. Cells. 2019;8(8). doi: 10.3390/cells8080886
- Vereb Z, Poliska S, Albert R, et al. Role of human corneal stroma-derived mesenchymal-like stem cells in corneal immunity and wound healing. Sci Rep. 2016;6:26227. doi: 10.1038/srep26227
- Galea GL, Zein MR, Allen S, Francis-West P. Making and shaping endochondral and intramembranous bones. Dev Dyn. 2021;250(3):414-449. doi: 10.1002/dvdy.278
- Kozhemyakina E, Lassar AB, Zelzer E. A pathway to bone: signaling molecules and transcription factors involved in chondrocyte development and maturation. Development. 2015;142(5):817-831. doi: 10.1242/dev.105536
- Park IK, Cho CS. Stem cell-assisted approaches for cartilage tissue engineering. Int J Stem Cells. 2010;3(2):96-102. doi: 10.15283/ijsc.2010.3.2.96
- Guasto A, Cormier-Daire V. Signaling pathways in bone development and their related skeletal dysplasia. Int J Mol Sci. 2021;22(9). doi: 10.3390/ijms22094321
- Martin V, Bettencourt A. Bone regeneration: Biomaterials as local delivery systems with improved osteoinductive properties. Mater Sci Eng C Mater Biol Appl. 2018;82:363-371. doi: 10.1016/j.msec.2017.04.038
- Yan L, Cinar A, Ma S, Abel R, Hansen U, Marrow TJ. A method for fracture toughness measurement in trabecular bone using computed tomography, image correlation and finite element methods. J Mech Behav Biomed Mater. 2020;109:103838. doi: 10.1016/j.jmbbm.2020.103838
- Haleem A, Javaid M, Khan RH, Suman R. 3D printing applications in bone tissue engineering. J Clin Orthop Trauma. 2020;11(Suppl 1):S118-S124. doi: 10.1016/j.jcot.2019.12.002
- Yi S, Ding F, Gong L, Gu X. Extracellular matrix scaffolds for tissue engineering and regenerative medicine. Curr Stem Cell Res Ther. 2017;12(3):233-246. doi: 10.2174/1574888X11666160905092513
- Zhu W, Ma X, Gou M, Mei D, Zhang K, Chen S. 3D printing of functional biomaterials for tissue engineering. Curr Opin Biotechnol. 2016;40:103-112. doi: 10.1016/j.copbio.2016.03.014
- Szűcs D, Fekete Z, Guba, et al. Toward better drug development: three-dimensional bioprinting in toxicological research. Int J Bioprint. 2023;9(2):663. doi: 10.18063/ijb.v9i2.663
- Xu J, Zheng S, Hu X, et al. Advances in the research of bioinks based on natural collagen, polysaccharide and their derivatives for skin 3D bioprinting. Polymers (Basel). 2020;12(6). doi: 10.3390/polym12061237
- Lee VK, Dai G. Three-dimensional bioprinting and tissue fabrication: prospects for drug discovery and regenerative medicine. Adv Healthcare Technol. 2015;1:23-35. doi: 10.2147/AHCT.S69191
- Sundaramurthi D, Rauf S, Hauser CAE. 3D bioprinting technology for regenerative medicine applications. Int J Bioprint. 2016;2(2):9-26. doi: 10.18063/IJB.2016.02.010
- Vijayavenkataraman S, Yan WC, Lu WF, Wang CH, Fuh JYH. 3D bioprinting of tissues and organs for regenerative medicine. Adv Drug Deliv Rev. 2018;132:296-332. doi: 10.1016/j.addr.2018.07.004
- Zhou D, Chen J, Liu B, Zhang X, Li X, Xu T. Bioinks for jet-based bioprinting. Bioprinting. 2019;16:e00060. doi: 10.1016/j.bprint.2019.e00060
- He Y, Gu Z, Xie M, Fu J, Lin H. Why choose 3D bioprinting? Part II: methods and bioprinters. Bio-Des Manuf. 2020;3:1-4. doi: 10.1007/s42242-020-00064-w
- Fontes A, Marcomini RF. 3D bioprinting: a review of materials, processes and bioink properties. J Eng Exact Sci. 2020;6(5):0617-0639. doi: 10.18540/jcecvl6iss5pp0617-0639
- Chowdhury SR, Lokanathan Y, Xian LJ, et al. 3D printed bioscaffolds for developing tissue-engineered constructs. In: Yasa E, Mhadhbi M, Santecchia E, eds. Design and Manufacturing. 2020. doi: 10.5772/intechopen.92418
- Gao D, Zhou JG. Designs and applications of electrohydrodynamic 3D printing. Int J Bioprint. 2019;5(1):172. doi: 10.18063/ijb.v5i1.172
- Gudapati H, Dey M, Ozbolat I. A comprehensive review on droplet-based bioprinting: past, present and future. Biomaterials. 2016;102:20-42. doi: 10.1016/j.biomaterials.2016.06.012
- Ventura RD. An overview of laser-assisted bioprinting (LAB) in tissue engineering applications. Med Lasers. 2021;10(2):76-81. doi: 10.25289/ML.2021.10.2.76
- Leberfinger AN, Ravnic DJ, Dhawan A, Ozbolat IT. Concise review: bioprinting of stem cells for transplantable tissue fabrication. Stem Cells Transl Med. 2017;6(10):1940-1948. doi: 10.1002/sctm.17-0148
- Lee JM, Sing SL, Zhou M, Yeong WY. 3D bioprinting processes: a perspective on classification and terminology. Int J Bioprint. 2018;4(2):151. doi: 10.18063/IJB.v4i2.151
- Ng WL, Lee JM, Zhou M, et al. Vat polymerization-based bioprinting-process, materials, applications and regulatory challenges. Biofabrication. 2020;12(2):022001. doi: 10.1088/1758-5090/ab6034
- Faraji Rad Z, Prewett PD, Davies GJ. High-resolution two-photon polymerization: the most versatile technique for the fabrication of microneedle arrays. Microsyst Nanoeng. 2021;7:71. doi: 10.1038/s41378-021-00298-3
- Liu F, Chen Q, Liu C, et al. Natural polymers for organ 3D bioprinting. Polymers (Basel). 2018;10(11):1278. doi: 10.3390/polym10111278
- Wang C, Huang W, Zhou Y, et al. 3D printing of bone tissue engineering scaffolds. Bioact Mater. 2020;5(1):82-91. doi: 10.1016/j.bioactmat.2020.01.004
- Eshraghi S, Das S. Mechanical and microstructural properties of polycaprolactone scaffolds with one-dimensional, two-dimensional, and three-dimensional orthogonally oriented porous architectures produced by selective laser sintering. Acta Biomater. 2010;6(7):2467-2476. doi: 10.1016/j.actbio.2010.02.002
- Lu L, Zhang Q, Wootton DM, et al. Mechanical study of polycaprolactone-hydroxyapatite porous scaffolds created by porogen-based solid freeform fabrication method. J Appl Biomater Funct Mater. 2014;12(3):145-154. doi: 10.5301/JABFM.5000163
- Koch F, Thaden O, Conrad S, et al. Mechanical properties of polycaprolactone (PCL) scaffolds for hybrid 3D-bioprinting with alginate-gelatin hydrogel. J Mech Behav Biomed Mater. 2022;130:105219. doi: 10.1016/j.jmbbm.2022.105219
- Jakus AE, Rutz AL, Jordan SW, et al. Hyperelastic “bone”: a highly versatile, growth factor-free, osteoregenerative, scalable, and surgically friendly biomaterial. Sci Transl Med. 2016;8(358):358ra127. doi: 10.1126/scitranslmed.aaf7704
- Gentile P, Chiono V, Carmagnola I, Hatton PV. An overview of poly(lactic-co-glycolic) acid (PLGA)-based biomaterials for bone tissue engineering. Int J Mol Sci. 2014;15(3): 3640-3659. doi: 10.3390/ijms15033640
- Petros S, Tesfaye T, Ayele M. A review on gelatin based hydrogels for medical textile applications. J Eng. 2020;2020:8866582. doi: 10.1155/2020/8866582
- Sanchez-Fernandez JA, Presbitero-Espinosa G, Pena- Paras L, et al. Characterization of sodium alginate hydrogels reinforced with nanoparticles of hydroxyapatite for biomedical applications. Polymers (Basel). 2021; 13(17). doi: 10.3390/polym13172927
- Tan JJY, Lee CP, Hashimoto M. Preheating of gelatin improves its printability with transglutaminase in direct ink writing 3D printing. Int J Bioprint. 2020;6(4):296. doi: 10.18063/ijb.v6i4.296
- Mancha Sanchez E, Gomez-Blanco JC, Lopez Nieto E, et al. Hydrogels for bioprinting: a systematic review of hydrogels synthesis, bioprinting parameters, and bioprinted structures behavior. Front Bioeng Biotechnol. 2020;8:776. doi: 10.3389/fbioe.2020.00776
- Yue K, Trujillo-de Santiago G, Alvarez MM, Tamayol A, Annabi N, Khademhosseini A. Synthesis, properties, and biomedical applications of gelatin methacryloyl (GelMA) hydrogels. Biomaterials. 2015;73:254-271. doi: 10.1016/j.biomaterials.2015.08.045
- Piao Y, You H, Xu T, et al. Biomedical applications of gelatin methacryloyl hydrogels. Eng Regener. 2021;2:47-56. doi: 10.1016/j.engreg.2021.03.002
- Lazaridou M, Bikiaris DN, Lamprou DA. 3D bioprinted chitosan-based hydrogel scaffolds in tissue engineering and localised drug delivery. Pharmaceutics. 2022;14(9):1978. doi: 10.3390/pharmaceutics14091978
- Khunmanee S, Jeong Y, Park H. Crosslinking method of hyaluronic-based hydrogel for biomedical applications. J Tissue Eng. 2017;8:2041731417726464. doi: 10.1177/2041731417726464
- Zheng H, Zuo B. Functional silk fibroin hydrogels: preparation, properties and applications. J Mater Chem B. 2021;9(5):1238-1258. doi: 10.1039/d0tb02099k
- Amirazad H, Dadashpour M, Zarghami N. Application of decellularized bone matrix as a bioscaffold in bone tissue engineering. J Biol Eng. 2022;16(1):1. doi: 10.1186/s13036-021-00282-5
- Boso D, Maghin E, Carraro E, Giagante M, Pavan P, Piccoli M. Extracellular matrix-derived hydrogels as biomaterial for different skeletal muscle tissue replacements. Materials (Basel). 2020;13(11):2483. doi: 10.3390/ma13112483
- Kim YS, Majid M, Melchiorri AJ, Mikos AG. Applications of decellularized extracellular matrix in bone and cartilage tissue engineering. Bioeng Transl Med. 2019;4(1):83-95. doi: 10.1002/btm2.10110
- Pati F, Jang J, Ha DH, et al. Printing three-dimensional tissue analogues with decellularized extracellular matrix bioink. Nat Commun. 2014;5:3935. doi: 10.1038/ncomms4935
- Hickey RJ, Pelling AE. Cellulose biomaterials for tissue engineering. Front Bioeng Biotechnol. 2019;7:45. doi: 10.3389/fbioe.2019.00045
- Choi JR, Yong KW, Choi JY, Cowie AC. Recent advances in photo-crosslinkable hydrogels for biomedical applications. Biotechniques. 2019;66(1):40-53. doi: 10.2144/btn-2018-0083
- Kumar A, Kargozar, Baino F, Han SS. Additive manufacturing methods for producing hydroxyapatite and hydroxyapatite-based composite scaffolds: a review. Front Mater. 2019;6:313. doi: 10.3389/fmats.2019.00313
- Habibah TU, Amlani DV, Brizuela M. Hydroxyapatite Dental Material. Treasure Island (FL): StatPearls; 2022.
- Zhang M, Lin R, Wang X, et al. 3D printing of Haversian bone-mimicking scaffolds for multicellular delivery in bone regeneration. Sci Adv. 2020;6(12):eaaz6725. doi: 10.1126/sciadv.aaz6725
- Brazete D, Torres PMC, Abrantes JCC, Ferreira JMF. Influence of the Ca/P ratio and cooling rate on the allotropic α↔β-tricalcium phosphate phase transformations. Ceram Int. 2018;44(7):8249-8256. doi: 10.1016/j.ceramint.2018.02.005
- Kim WJ, Yun HS, Kim GH. An innovative cell-laden alpha- TCP/collagen scaffold fabricated using a two-step printing process for potential application in regenerating hard tissues. Sci Rep. 2017;7(1):3181. doi: 10.1038/s41598-017-03455-9
- Bouler JM, Pilet P, Gauthier O, Verron E. Biphasic calcium phosphate ceramics for bone reconstruction: a review of biological response. Acta Biomater. 2017;53:1-12. doi: 10.1016/j.actbio.2017.01.076
- Dukle A, Murugan D, Nathanael AJ, Rangasamy L, Oh TH. Can 3D-printed bioactive glasses be the future of bone tissue engineering? Polymers (Basel). 2022;14(8). doi: 10.3390/polym14081627
- Heid S, Boccaccini AR. Advancing bioinks for 3D bioprinting using reactive fillers: a review. Acta Biomater. 2020;113:1-22. doi: 10.1016/j.actbio.2020.06.040
- Alawi SA, Matschke J, Muallah D, Gelinksy M, Dragu A. 3D bioprinting in plastic and reconstructive surgery: current concepts, progress, and clinical application. Eur J Plast Surg. 2023;46:833-843. doi: 10.1007/s00238-023-02108-7
- Yazdanpanah Z, Johnston JD, Cooper DML, Chen XB. 3D bioprinted scaffolds for bone tissue engineering: state-of-the-art and emerging technologies. Front Bioeng Biotech. 2022;10:824156. doi: 10.3389/fbioe.2022.824156
- Lim W, Kim B, Moon YL. Three-dimensional bioprinting for bone and cartilage transplantation. Ann Joint. 2019;4(1). doi: 10.21037/aoj.2018.12.06
- Genova T, Roato I, Carossa M, Motta C, Cavagnetto D, Mussano F. Advances on bone substitutes through 3D bioprinting. Int J Mol Sci. 2020;21(19). doi: 10.3390/ijms21197012
- Abu Owida H. Developments and clinical applications of biomimetic tissue regeneration using 3D bioprinting technique. Appl Bionics Biomech. 2022;2022: 2260216. doi: 10.1155/2022/2260216
- Su X, Wang T, Guo S. Applications of 3D printed bone tissue engineering scaffolds in the stem cell field. Regen Ther. 2021;16:63-72. doi: 10.1016/j.reth.2021.01.007
- Hao Y, Cao B, Deng L, et al. The first 3D-bioprinted personalized active bone to repair bone defects: a case report. Int J Bioprint. 2023;9(2):654. doi: 10.18063/ijb.v9i2.654
- Liu C, Wang L, Lu W, et al. Computer vision-aided bioprinting for bone research. Bone Res. 2022;10(1):21. doi: 10.1038/s41413-022-00192-2
- Wan Z, Zhang P, Liu Y, Lv L, Zhou Y. Four-dimensional bioprinting: current developments and applications in bone tissue engineering. Acta Biomater. 2020;101:26-42. doi: 10.1016/j.actbio.2019.10.038
- Kotturi H, Abuabed A, Zafar H, et al. Evaluation of polyethylene glycol diacrylate-polycaprolactone scaffolds for tissue engineering applications. J Funct Biomater. 2017;8(3). doi: 10.3390/jfb8030039
- Khalaf AT, Wei Y, Wan J, et al. Bone tissue engineering through 3D bioprinting of bioceramic scaffolds: a review and update. Life (Basel). 2022;12(6). doi: 10.3390/life12060903
- Liu W, Jing X, Xu Z, Teng C. PEGDA/HA mineralized hydrogel loaded with Exendin4 promotes bone regeneration in rat models with bone defects by inducing osteogenesis. J Biomater Appl. 2021;35(10):1337-1346. doi: 10.1177/088532822098704
- Yang F, Williams CG, Wang DA, Lee H, Manson PN, Elisseeff J. The effect of incorporating RGD adhesive peptide in polyethylene glycol diacrylate hydrogel on osteogenesis of bone marrow stromal cells. Biomaterials. 2005;26(30): 5991-5998. doi: 10.1016/j.biomaterials.2005.03.018
- Sousa AC, Biscaia S, Alvites R, et al. Assessment of 3D-printed polycaprolactone, hydroxyapatite nanoparticles and diacrylate poly(ethylene glycol) scaffolds for bone regeneration. Pharmaceutics. 2022;14(12):2643. doi: 10.3390/pharmaceutics14122643
- Stillman ZS, Jarai BM, Raman N, Patel P, Fromen CA. Degradation profiles of poly(ethylene glycol) diacrylate (PEGDA)-based hydrogel nanoparticles. Polym Chem. 2020;11(2):568-580. doi: 10.1039/c9py01206k
- Thrivikraman G, Athirasala A, Twohig C, Boda SK, Bertassoni LE. Biomaterials for craniofacial bone regeneration. Dent Clin North Am. 2017;61(4):835-856. doi: 10.1016/j.cden.2017.06.003
- Ahmed AG, Awartani FA Niazy AA, Jansen JA, Alghamdi HS. A combination of biphasic calcium phosphate (Maxresorb®) and hyaluronic acid gel (Hyadent®) for repairing osseous defects in a rat model. Appl Sci. 2020;10(5):1651. doi: 10.3390/app10051651
- Genova T, Roato I, Carossa M, Motta C, Cavagnetto D, Mussano F. Advances on bone substitutes through 3D bioprinting. Int J Mol Sci. 2020;21(19):7012. doi: 10.3390/ijms21197012
- Beheshtizadeh N, Azami M, Abbasi H, Farzin A. Applying extrusion-based 3D printing technique accelerates fabricating complex biphasic calcium phosphate-based scaffolds for bone tissue regeneration. J Adv Res. 2022;40:69-94. doi: 10.1016/j.jare.2021.12.012
- Fedorovich NE, Schuurman W, Wijnberg HM, et al. Biofabrication of osteochondral tissue equivalents by printing topologically defined, cell-laden hydrogel scaffolds. Tissue Eng Part C Methods. 2012;18(1):33-44. doi: 10.1089/ten.TEC.2011.0060
- Jiao X, Sun X, Li W, et al. 3D-printed beta-tricalcium phosphate scaffolds promote osteogenic differentiation of bone marrow-deprived mesenchymal stem cells in an N6-methyladenosine-dependent manner. Int J Bioprint. 2022;8(2):544. doi: 10.18063/ijb.v8i2.544
- Cunniffe GM, Gonzalez-Fernandez T, Daly A, et al. (*) Three-dimensional bioprinting of polycaprolactone reinforced gene activated bioinks for bone tissue engineering. Tissue Eng Part A. 2017;23(17-18):891-900. doi: 10.1089/ten.tea.2016.0498
- Cidonio G, Glinka M, Kim YH, et al. Nanoclay-based 3D printed scaffolds promote vascular ingrowth ex vivo and generate bone mineral tissue in vitro and in vivo. Biofabrication. 2020;12(3):035010. doi: 10.1088/1758-5090/ab8753
- Sun X, Ma Z, Zhao X, et al. Three-dimensional bioprinting of multicell-laden scaffolds containing bone morphogenic protein-4 for promoting M2 macrophage polarization and accelerating bone defect repair in diabetes mellitus. Bioact Mater. 2021;6(3):757-769. doi: 10.1016/j.bioactmat.2020.08.030
- Kang HW, Lee SJ, Ko IK, Kengla C, Yoo JJ, Atala A. A 3D bioprinting system to produce human-scale tissue constructs with structural integrity. Nat Biotechnol. 2016;34(3):312-319. doi: 10.1038/nbt.3413
- Rukavina P, Koch F, Wehrle M, et al. In vivo evaluation of bioprinted prevascularized bone tissue. Biotechnol Bioeng. 2020;117(12):3902-3911. doi: 10.1002/bit.27527
- Loozen LD, Wegman F, Oner FC, Dhert WJA, Alblas J. Porous bioprinted constructs in BMP-2 non-viral gene therapy for bone tissue engineering. J Mater Chem B. 2013;1(48):6619-6626. doi: 10.1039/c3tb21093f
- Dubey N, Ferreira JA, Malda J, Bhaduri SB, Bottino MC. Extracellular matrix/amorphous magnesium phosphate bioink for 3D bioprinting of craniomaxillofacial bone tissue. ACS Appl Mater Interfaces. 2020;12(21):23752-23763. doi: 10.1021/acsami.0c05311
- Zhai X, Ruan C, Ma Y, et al. 3D-bioprinted osteoblast-laden nanocomposite hydrogel constructs with induced microenvironments promote cell viability, differentiation, and osteogenesis both in vitro and in vivo. Adv Sci (Weinh). 2018;5(3):1700550. doi: 10.1002/advs.201700550
- Keriquel V, Oliveira H, Remy M, et al. In situ printing of mesenchymal stromal cells, by laser-assisted bioprinting, for in vivo bone regeneration applications. Sci Rep. 2017;7(1):1778. doi: 10.1038/s41598-017-01914-x
- Park JY, Shim JH, Choi SA, et al. 3D printing technology to control BMP-2 and VEGF delivery spatially and temporally to promote large-volume bone regeneration. J Mater Chem B. 2015;3(27):5415-5425. doi: 10.1039/c5tb00637f
- Keller L, Regiel-Futyra A, Gimeno M, et al. Chitosan-based nanocomposites for the repair of bone defects. Nanomedicine. 2017;13(7):2231-2240. doi: 10.1016/j.nano.2017.06.007
- Korn P, Ahlfeld T, Lahmeyer F, et al. 3D printing of bone grafts for cleft alveolar osteoplasty - in vivo evaluation in a preclinical model. Front Bioeng Biotechnol. 2020; 8:217. doi: 10.3389/fbioe.2020.00217
- Liu X, Miao Y, Liang H, et al. 3D-printed bioactive ceramic scaffolds with biomimetic micro/nano-HAp surfaces mediated cell fate and promoted bone augmentation of the bone-implant interface in vivo. Bioact Mater. 2022;12:120-132. doi: 10.1016/j.bioactmat.2021.10.016
- Nulty J, Freeman FE, Browe DC, et al. 3D bioprinting of prevascularised implants for the repair of critically-sized bone defects. Acta Biomater. 2021;126:154-169. doi: 10.1016/j.actbio.2021.03.003
- Piard C, Baker H, Kamalitdinov T, Fisher J. Bioprinted osteon-like scaffolds enhance in vivo neovascularization. Biofabrication. 2019;11(2):025013. doi: 10.1088/1758-5090/ab078a
- Daly AC, Pitacco P, Nulty J, Cunniffe GM, Kelly DJ. 3D printed microchannel networks to direct vascularisation during endochondral bone repair. Biomaterials. 2018;162:34-46. doi: 10.1016/j.biomaterials.2018.01.057