4D printing and simulation of body temperature-responsive shape-memory polymers for advanced biomedical applications
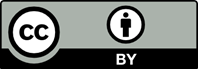
Four-dimensional (4D) bioprinting holds significant promise in precision medicine, enabling the emulation of dynamic changes in the human body and tissues to provide personalized treatments. Smart materials for 4D printing (i.e., responsive to specific stimuli) should exhibit properties, such as biodegradability, biocompatibility, and ease of processing, while also enabling the prediction of time-dependent behavior in programmed geometry. This study focused on the development of a body temperature-responsive shape-memory polymer (SMP). The thermal properties of the SMP were analyzed, and its biodegradability and biocompatibility were assessed through structures fabricated by three-dimensional printing techniques. The simulation calculated with this finite element (FE) solution was in good agreement with those measured in experiments. The findings contribute to our understanding of the behavior of SMP under various conditions, validating the effectiveness of the developed material for potential applications in precision medicine and 4D bioprinting. It has the potential for use in medical devices, such as catheters, stents, and artificial scaffolds, prepared by 4D bioprinting.
- Tibbits S. 4D printing: multi-material shape change. Archit Des. 2014;84(1):116-121. doi: 10.1002/ad.1710
- Gao B, Yang Q, Zhao X, Jin G, Ma Y, Xu F. 4D bioprinting for biomedical applications. Trends Biotechnol. 2016;34(9): 746-756. doi: 10.1016/j.tibtech.2016.03.004
- Lui YS, Sow WT, Tan LP, Wu Y, Lai Y, Li H. 4D printing and stimuli-responsive materials in biomedical aspects. Acta Biomater. 2019;92:19-36. doi: 10.1016/j.actbio.2019.05.005
- Ghosh S, Chaudhuri S, Roy P, Lahiri D. 4D printing in biomedical engineering: a state-of-the-art review of technologies, biomaterials, and application. Regen Eng Transl Med. 2023;9(3):339-365. doi: 10.1007/s40883-022-00288-5
- Martinez-De-Anda AA, Rodriguez-Salvador M, Castillo- Valdez PF. The attractiveness of 4D printing in the medical field: revealing scientific and technological advances in design factors and applications. Int J Bioprint. 2023;9(6): 187-199. doi: 10.36922/IJB.1112
- Han S, Wu J. Three-dimensional (3D) scaffolds as powerful weapons for tumor immunotherapy. Bioact Mater. 2022;17:300-319. doi: 10.1016/j.bioactmat.2022.01.020
- Chen X, Han S, Wu W, et al. Harnessing 4D printing bioscaffolds for advanced orthopedics. Small. 2022;18:2106824. doi: 10.1002/smll.202106824
- Chen A, Wang W, Mao Z, et al. Multimaterial 3D and 4D bioprinting of heterogenous constructs for tissue engineering. Adv Mater. 2023;2307686. doi: 10.1002/adma.202307686
- Yang Q, Gao B, Xu F. Recent advances in 4D bioprinting. Biotechnol J. 2020;15(1):1-10. doi: 10.1002/biot.201900086
- Zhang C, Cai D, Liao P, et al. 4D printing of shape-memory polymeric scaffolds for adaptive biomedical implantation. Acta Biomater. 2021;122:101-110. doi: 10.1016/j.actbio.2020.12.042
- Xu J, Song J. Polylactic acid (PLA)-based shape-memory materials for biomedical applications. Shape Mem Polym Biomed Appl. 2015:197-217. doi: 10.1016/B978-0-85709-698-2.00010-6
- Qu G, Huang J, Gu G, Li Z, Wu X, Ren J. Smart implants: 4D-printed shape-morphing scaffolds for medical implantation. Int J Bioprint. 2023;9(5). doi: 10.18063/ijb.764
- Guo Y, Huang J, Fang Y, Huang H, Wu J. 1D, 2D, and 3D scaffolds promoting angiogenesis for enhanced wound healing. Chem Eng J. 2022;437:134690. doi: 10.1016/j.cej.2022.134690
- Saska S, Pilatti L, Blay A, Shibli JA. Bioresorbable polymers: advanced materials and 4D printing for tissue engineering. Polymers (Basel). 2021;13(4):1-24. doi: 10.3390/polym13040563
- Khalid MY, Arif ZU, Noroozi R, Zolfagharian A, Bodaghi M. 4D printing of shape memory polymer composites: a review on fabrication techniques, applications, and future perspectives. J Manuf Process. 2022;81:759-797. doi: 10.1016/j.jmapro.2022.07.035
- Sydney Gladman A, Matsumoto EA, Nuzzo RG, Mahadevan L, Lewis JA. Biomimetic 4D printing. Nat Mater. 2016;15(4):413-418. doi: 10.1038/nmat4544
- Patil AN, Sarje SH. Additive manufacturing with shape changing/memory materials: a review on 4D printing technology. Mater Today Proc. 2021;44:1744-1749. doi: 10.1016/j.matpr.2020.11.907
- Costa PDC, Costa DCS, Correia TR, Gaspar VM, Mano JF. Natural origin biomaterials for 4D bioprinting tissue-like constructs. Adv Mater Technol. 2021;6(10):1-21. doi: 10.1002/admt.202100168
- Miao S, Castro N, Nowicki M, et al. 4D printing of polymeric materials for tissue and organ regeneration. Mater Today. 2017;20(10):577-591. doi: 10.1016/j.mattod.2017.06.005
- Zhao W, Liu L, Zhang F, Leng J, Liu Y. Shape memory polymers and their composites in biomedical applications. Mater Sci Eng C. 2019;97:864-883. doi: 10.1016/j.msec.2018.12.054
- Jin Z, Hu G, Zhang Z, Yu SY, Gu GX. Modeling and analysis of post-processing conditions on 4D-bioprinting of deformable hydrogel-based biomaterial inks. Bioprinting. 2023;33:e00286. doi: 10.1016/j.bprint.2023.e00286
- Feng YC, Bodaghi M, Liao WH. Numerical/experimental assessment of 3D-printed shape-memory polymeric beams. J Appl Polym Sci. 2019;136(16):1-12. doi: 10.1002/app.47422
- Yu K, Xie T, Leng J, Ding Y, Qi HJ. Mechanisms of multi-shape memory effects and associated energy release in shape memory polymers. Soft Matter. 2012;8(20):5687-5695. doi: 10.1039/c2sm25292a
- Dogan SK, Boyacioglu S, Kodal M, Gokce O, Ozkoc G. Thermally induced shape memory behavior, enzymatic degradation and biocompatibility of PLA/TPU blends: “effects of compatibilization.” J Mech Behav Biomed Mater. 2017;71:349-361. doi: 10.1016/j.jmbbm.2017.04.001
- Yang B, Huang WM, Li C, Chor JH. Effects of moisture on the glass transition temperature of polyurethane shape memory polymer filled with nano-carbon powder. Eur Polym J. 2005;41(5):1123-1128. doi: 10.1016/j.eurpolymj.2004.11.029
- Zhang H, Wang H, Zhong W, Du Q. A novel type of shape memory polymer blend and the shape memory mechanism. Polymer (Guildf). 2009;50(6):1596-1601. doi: 10.1016/j.polymer.2009.01.011
- Huskić M, Kusić D, Pulko I, Nardin B. Determination of residual stresses in amorphous thermoplastic polymers by DMA. J Appl Polym Sci. 2022;139(48):1-8. doi: 10.1002/app.53210
- Ziegler W, Guttmann P, Kopeinig S, et al. Influence of different polyol segments on the crystallisation behavior of polyurethane elastomers measured with DSC and DMA experiments. Polym Test. 2018;71:18-26. doi: 10.1016/j.polymertesting.2018.08.021
- De Nardo L, Farè S. Dynamico-mechanical characterization of polymer biomaterials. Charact Polym Biomater. 2017: 203-232. doi: 10.1016/B978-0-08-100737-2.00009-1
- Göpferich A. Mechanisms of polymer degradation and erosion1. Biomater Silver Jubil Compend. 1996;17(2): 117-128. doi: 10.1016/B978-008045154-1.50016-2
- Vasanthan N, Ly O. Effect of microstructure on hydrolytic degradation studies of poly (l-lactic acid) by FTIR spectroscopy and differential scanning calorimetry. Polym Degrad Stab. 2009;94(9):1364-1372. doi: 10.1016/j.polymdegradstab.2009.05.015
- Araque-Monrós MC, Vidaurre A, Gil-Santos L, Gironés Bernabé S, Monleón-Pradas M, Más-Estellés J. Study of the degradation of a new PLA braided biomaterial in buffer phosphate saline, basic and acid media, intended for the regeneration of tendons and ligaments. Polym Degrad Stab. 2013;98(9):1563-1570. doi: 10.1016/j.polymdegradstab.2013.06.031
- Kim D, Lee J, Min Seok J, et al. Three-dimensional bioprinting of bioactive scaffolds with thermally embedded abalone shell particles for bone tissue engineering. Mater Des. 2021;212. doi: 10.1016/j.matdes.2021.110228
- Kohn DH, Sarmadi M, Helman JI, Krebsbach PH. Effects of pH on human bone marrow stromal cells in vitro: implications for tissue engineering of bone. J Biomed Mater Res. 2002;60(2):292-299. doi: 10.1002/jbm.10050
- Mao H, Yang L, Zhu H, et al. Recent advances and challenges in materials for 3D bioprinting. Prog Nat Sci Mater Int. 2020;30(5):618-634. doi: 10.1016/j.pnsc.2020.09.015
- Kim D, Lee SJ, Lee D, et al. Biomimetic mineralization of 3D-printed polyhydroxyalkanoate-based microbial scaffolds for bone tissue engineering. Int J Bioprint. 2024;10(2):1806. doi: 10.36922/ijb.1806
- Yin GB, Zhang YZ, Wang SD, Shi DB, Dong ZH, Fu WG. Study of the electrospun PLA/silk fibroin-gelatin composite nanofibrous scaffold for tissue engineering. J Biomed Mater Res - Part A. 2010;93(1):158-163. doi: 10.1002/jbm.a.32496
- Wang S, Wang Y, Chen B, et al. Preparation and performance study of multichannel PLA artificial nerve conduits. Biomed Mater. 2023;18(6). doi: 10.1088/1748-605X/acf0ae
- Li Y, Liu Z. A novel constitutive model of shape memory polymers combining phase transition and viscoelasticity. Polymer (Guildf). 2018;143:298-308. doi: 10.1016/j.polymer.2018.04.026
- Noroozi R, Bodaghi M, Jafari H, Zolfagharian A, Fotouhi M. Shape-adaptive metastructures with variable bandgap regions by 4D printing. Polymers (Basel). 2020;12(3). doi: 10.3390/polym12030519