3D bioprinting in otorhinolaryngology: from bench to clinical application
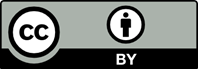
Three-dimensional (3D) bioprinting is a promising additive manufacturing technology that uses imaging data and computer-assisted deposition of biological materials or cells to reconstruct complex 3D structures accurately. This technology has progressed rapidly, in part because of its integration across multiple disciplines and combination with other technologies for clinical applications. Advances in experimental research and clinical applications related to otorhinolaryngology have led to the development of diagnostic and treatment methods based on 3D bioprinting, including the development of tissue engineering scaffolds, biosensors, organ chips, and organoids, surgical planning, graft construction, and medical education. Additionally, otorhinolaryngologists will be better equipped to treat tissue function defects with personalized printed graft implants. It is also expected that 3D printing can be used to build ideal in vitro models in the future to help solve existing research challenges. This article briefly introduces the relevant 3D bioprinting technologies and bioinks that can be used by otorhinolaryngologists and discusses their potential applications in otorhinolaryngology.
- Lott DG, Janus JR. Tissue engineering for otorhinolaryngology–head and neck surgery. Mayo Clin Proc. 2014;89(12):1722-1733. doi: 10.1016/j.mayocp.2014.09.007
- McMillan A, McMillan N, Gupta N, Kanotra SP, Salem AK. 3D bioprinting in otolaryngology: a review. Adv Healthc Mater. 2023;12(19):e2203268. doi: 10.1002/adhm.202203268
- Sun Y, Wang EH, Yu JT, et al. A novel surgery classification for endoscopic approaches to middle ear cholesteatoma. Curr Med Sci. 2020;40(1):9-17. doi: 10.1007/s11596-020-2141-0
- Kuru I, Maier H, Müller M, Lenarz T, Lueth TC. A 3D-printed functioning anatomical human middle ear model. Hear Res. 2016;340:204-213. doi: 10.1016/j.heares.2015.12.025
- MacDonald E, Wicker R. Multiprocess 3D printing for increasing component functionality. Science. 2016;353(6307):aaf2093. doi: 10.1126/science.aaf2093
- Zhang Y, Dong Z, Li C, et al. Continuous 3D printing from one single droplet. Nat Commun. 2020;11(1):4685. doi: 10.1038/s41467-020-18518-1
- Kang HW, Lee SJ, Ko IK, Kengla C, Yoo JJ, Atala A. A 3D bioprinting system to produce human-scale tissue constructs with structural integrity. Nat Biotechnol. 2016;34(3): 312-319. doi: 10.1038/nbt.3413
- Jia MS, Rao RR, Elsaadany M. Early introduction of 3D modeling modules promotes the development of simulation skills in downstream biomedical engineering curricula. J Biol Eng. 2023;17(1):26. doi: 10.1186/s13036-023-00339-7
- Truby RL, Lewis JA. Printing soft matter in three dimensions. Nature. 2016;540(7633):371-378. doi: 10.1038/nature21003
- Wüst S, Müller R, Hofmann S. Controlled positioning of cells in biomaterials-approaches towards 3D tissue printing. J Funct Biomater. 2011;2(3):119-154. doi: 10.3390/jfb2030119
- Miller JS, Stevens KR, Yang MT, et al. Rapid casting of patterned vascular networks for perfusable engineered three-dimensional tissues. Nat Mater. 2012;11(9):768-774. doi: 10.1038/nmat3357
- Whitaker M. The history of 3D printing in healthcare. Bulletin. 2014;96(7):228-229. doi: 10.1308/147363514X13990346756481
- Thayer P, Martinez H, Gatenholm E. History and trends of 3D bioprinting. Methods Mol Biol. 2020;2140:3-18. doi: 10.1007/978-1-0716-0520-2_1
- Bhatia SN, Ingber DE. Microfluidic organs-on-chips. Nat Biotechnol. 2014;32(8):760-772. doi: 10.1038/nbt.2989
- Cohen J, Reyes SA. Creation of a 3D printed temporal bone model from clinical CT data. Am J Otolaryngol. 2015;36(5):619-624. doi: 10.1016/j.amjoto.2015.02.012
- Kozin ED, Remenschneider AK, Cheng S, Nakajima HH, Lee DJ. Three-dimensional printed prosthesis for repair of superior canal dehiscence. Otolaryngol Head Neck Surg. 2015;153(4):616-619. doi: 10.1177/0194599815592602
- AlReefi MA, Nguyen LHP, Mongeau LG, et al. Development and validation of a septoplasty training model using 3-dimensional printing technology. Int Forum Allergy Rhinol. 2017;7(4):399-404. doi: 10.1002/alr.21887
- Nuseir A, Hatamleh M, Watson J, Al-Wahadni AM, Alzoubi F, Murad M. Improved construction of auricular prosthesis by digital technologies. J Craniofac Surg. 2015;26(6):e502-e505. doi: 10.1097/SCS.0000000000002012
- Chan HHL, Siewerdsen JH, Vescan A, Daly MJ, Prisman E, Irish JC. 3D rapid prototyping for otolaryngology—head and neck surgery: applications in image-guidance, surgical simulation and patient-specific modeling. PLoS One. 2015;10(9):e0136370. doi: 10.1371/journal.pone.0136370
- Mocanu H, Mocanu AI, Dascălu I, Schipor MA, Rădulescu M. Materials for ossicular chain reconstruction: history and evolution. Med Int. 2023;3(2):13. doi: 10.3892/mi.2023.73
- Ferris CJ, Gilmore KG, Wallace GG, In het Panhuis M. Biofabrication: an overview of the approaches used for printing of living cells. Appl Microbiol Biotechnol. 2013;97(10):4243-4258. doi: 10.1007/s00253-013-4853-6
- Miller JS, Burdick JA. Editorial: special issue on 3D printing of biomaterials. ACS Biomater Sci Eng. 2016;2(10): 1658-1661. doi: 10.1021/acsbiomaterials.6b00566
- Ning L, Chen X. A brief review of extrusion-based tissue scaffold bio-printing. Biotechnol J. 2017;12(8):1600671. doi: 10.1002/biot.201600671
- Chen DXB. Extrusion bioprinting of scaffolds. In: Extrusion Bioprinting of Scaffolds for Tissue Engineering Applications. Cham: Springer International Publishing; 2019:117-145. doi: 10.1007/978-3-030-03460-3_6
- Knowlton S, Onal S, Yu CH, Zhao JJ, Tasoglu S. Bioprinting for cancer research. Trends Biotechnol. 2015;33(9):504-513. doi: 10.1016/j.tibtech.2015.06.007
- Pati F, Jang J, Ha DH, et al. Printing three-dimensional tissue analogues with decellularized extracellular matrix bioink. Nat Commun. 2014;5:3935. doi: 10.1038/ncomms4935
- Nakamura M, Kobayashi A, Takagi F, et al. Biocompatible inkjet printing technique for designed seeding of individual living cells. Tissue Eng. 2005;11(11-12):1658-1666. doi: 10.1089/ten.2005.11.1658
- Dey M, Ozbolat IT. 3D bioprinting of cells, tissues and organs. Sci Rep. 2020;10(1). doi: 10.1038/s41598-020-70086-y
- Ovsianikov A, Gruene M, Pflaum M, et al. Laser printing of cells into 3D scaffolds. Biofabrication. 2010;2(1):014104. doi: 10.1088/1758-5082/2/1/014104
- Wang J, Xu C, Yang S, Wang L, Xu M. Continuous and highly accurate multi-material extrusion-based bioprinting with optical coherence tomography imaging. Int J Bioprint. 2023;9(3):707. doi: 10.18063/ijb.707
- Brion DAJ, Pattinson SW. Generalisable 3D printing error detection and correction via multi-head neural networks. Nat Commun. 2022;13(1):4654. doi: 10.1038/s41467-022-31985-y
- Bagnol R, Sprecher C, Peroglio M, et al. Coaxial micro-extrusion of a calcium phosphate ink with aqueous solvents improves printing stability, structure fidelity and mechanical properties. Acta Biomater. 2021;125:322-332. doi: 10.1016/j.actbio.2021.02.022
- Gudapati H, Dey M, Ozbolat I. A comprehensive review on droplet-based bioprinting: past, present and future. Biomaterials. 2016;102:20-42. doi: 10.1016/j.biomaterials.2016.06.012
- Demirci U. Acoustic picoliter droplets for emerging applications in semiconductor industry and biotechnology. J Microelectromech Syst. 2006;15(4):957-966. doi: 10.1109/JMEMS.2006.878879
- Chen H, Wu Z, Gong Z, et al. Acoustic bioprinting of patient-derived organoids for predicting cancer therapy responses. Adv Healthc Mater. 2022;11(13). doi: 10.1002/adhm.202102784
- Li X, Liu B, Pei B, et al. Inkjet bioprinting of biomaterials. Chem Rev. 2020;120(19):10793-10833. doi: 10.1021/acs.chemrev.0c00008
- Ji Y, Yang Q, Huang G, et al. Improved resolution and fidelity of droplet-based bioprinting by upward ejection. ACS Biomater Sci Eng. 2019;5(8):4112-4121. doi: 10.1021/acsbiomaterials.9b00400
- Roth EA, Xu T, Das M, Gregory C, Hickman JJ, Boland T. Inkjet printing for high-throughput cell patterning. Biomaterials. 2004;25(17):3707-3715. doi: 10.1016/j.biomaterials.2003.10.052
- Graham AD, Olof SN, Burke MJ, et al. High-resolution patterned cellular constructs by droplet-based 3D printing. Sci Rep. 2017;7(1):7004. doi: 10.1038/s41598-017-06358-x
- Moon S, Hasan SK, Song YS, et al. Layer by layer three-dimensional tissue epitaxy by cell-laden hydrogel droplets. Tissue Eng Part C: Methods. 2010;16(1):157-166. doi: 10.1089/ten.tec.2009.0179
- Ozbolat IT, Hospodiuk M. Current advances and future perspectives in extrusion-based bioprinting. Biomaterials. 2016;76:321-343. doi: 10.1016/j.biomaterials.2015.10.076
- Lee W, Pinckney J, Lee V, et al. Three-dimensional bioprinting of rat embryonic neural cells. Neuroreport. 2009;20(8): 798-803. doi: 10.1097/WNR.0b013e32832b8be4
- Zhang H, Hong E, Chen X, Liu Z. Machine learning enables process optimization of aerosol jet 3D printing based on the droplet morphology. ACS Appl Mater Interfaces. 2023. doi: 10.1021/acsami.2c21476
- Xu T, Binder KW, Albanna MZ, et al. Hybrid printing of mechanically and biologically improved constructs for cartilage tissue engineering applications. Biofabrication. 2012;5(1):015001. doi: 10.1088/1758-5082/5/1/015001
- Ebrahimi Orimi H, Hosseini Kolkooh SS, Hooker E, Narayanswamy S, Larrivée B, Boutopoulos C. Drop-on-demand cell bioprinting via laser induced side transfer (LIST). Sci Rep. 2020;10(1):9730. doi: 10.1038/s41598-020-66565-x
- Roversi K, Ebrahimi Orimi H, Erfanian M, Talbot S, Boutopoulos C. LIST: a newly developed laser-assisted cell bioprinting technology. Bio Protoc. 2022;12(19). doi: 10.21769/BioProtoc.4527
- Guillotin B, Souquet A, Catros S, et al. Laser assisted bioprinting of engineered tissue with high cell density and microscale organization. Biomaterials. 2010;31(28):7250-7256. doi: 10.1016/j.biomaterials.2010.05.055
- Kim SH, Yeon YK, Lee JM, et al. Precisely printable and biocompatible silk fibroin bioink for digital light processing 3D printing. Nat Commun. 2018;9(1):1620. doi: 10.1038/s41467-018-03759-y
- Zhang J, Wehrle E, Rubert M, Müller R. 3D bioprinting of human tissues: biofabrication, bioinks, and bioreactors. IJMS. 2021;22(8):3971. doi: 10.3390/ijms22083971
- Mandrycky C, Wang Z, Kim K, Kim DH. 3D bioprinting for engineering complex tissues. Biotechnol Adv. 2016;34(4): 422-434. doi: 10.1016/j.biotechadv.2015.12.011
- Xiong R, Zhang Z, Chai W, Huang Y, Chrisey DB. Freeform drop-on-demand laser printing of 3D alginate and cellular constructs. Biofabrication. 2015;7(4):045011. doi: 10.1088/1758-5090/7/4/045011
- Serien D, Sugioka K. Three-dimensional printing of pure proteinaceous microstructures by femtosecond laser multiphoton cross-linking. ACS Biomater Sci Eng. 2020;6(2):1279-1287. doi: 10.1021/acsbiomaterials.9b01619
- Kingsley DM, Roberge CL, Rudkouskaya A, et al. Laser-based 3D bioprinting for spatial and size control of tumor spheroids and embryoid bodies. Acta Biomater. 2019;95: 357-370. doi: 10.1016/j.actbio.2019.02.014
- Gugulothu SB, Asthana S, Homer-Vanniasinkam S, Chatterjee K. Trends in photopolymerizable bioinks for 3D bioprinting of tumor models. JACS Au. 2023;3(8): 2086-2106. doi: 10.1021/jacsau.3c00281 Eng C. 2021;130:112423. doi: 10.1016/j.msec.2021.112423
- Murphy SV, Atala A. 3D bioprinting of tissues and organs. Nat Biotechnol. 2014;32(8):773-785. doi: 10.1038/nbt.2958
- Mandrycky C, Wang Z, Kim K, Kim DH. 3D bioprinting for engineering complex tissues. Biotechnol Adv. 2016;34(4): 422-434. doi: 10.1016/j.biotechadv.2015.12.011
- Alhnan MA, Okwuosa TC, Sadia M, Wan KW, Ahmed W, Arafat B. Emergence of 3D printed dosage forms: opportunities and challenges. Pharm Res. 2016;33(8): 1817-1832. doi: 10.1007/s11095-016-1933-1
- Groll J, Burdick JA, Cho DW, et al. A definition of bioinks and their distinction from biomaterial inks. Biofabrication. 2018;11(1):013001. doi: 10.1088/1758-5090/aaec52
- Heid S, Boccaccini AR. Advancing bioinks for 3D bioprinting using reactive fillers: a review. Acta Biomater. 2020;113:1-22. doi: 10.1016/j.actbio.2020.06.040
- DeForest CA, Anseth KS. Advances in bioactive hydrogels to probe and direct cell fate. Annu Rev Chem Biomol Eng. 2012;3(1):421-444. doi: 10.1146/annurev-chembioeng-062011-080945
- Bertassoni LE, Cardoso JC, Manoharan V, et al. Direct-write bioprinting of cell-laden methacrylated gelatin hydrogels. Biofabrication. 2014;6(2):024105. doi: 10.1088/1758-5082/6/2/024105
- Li W, Mille LS, Robledo JA, Uribe T, Huerta V, Zhang YS. Recent advances in formulating and processing biomaterial inks for vat polymerization‐based 3D printing. Adv Healthc Mater. 2020;9(15):2000156. doi: 10.1002/adhm.202000156
- Seliktar D. Designing cell-compatible hydrogels for biomedical applications. Science. 2012;336(6085): 1124-1128. doi: 10.1126/science.1214804
- Guvendiren M, Burdick JA. Engineering synthetic hydrogel microenvironments to instruct stem cells. Curr Opin Biotechnol. 2013;24(5):841-846. doi: 10.1016/j.copbio.2013.03.009
- Lee V, Singh G, Trasatti JP, et al. Design and fabrication of human skin by three-dimensional bioprinting. Tissue Eng Part C: Methods. 2014;20(6):473-484. doi: 10.1089/ten.tec.2013.0335
- Xu T, Gregory C, Molnar P, et al. Viability and electrophysiology of neural cell structures generated by the inkjet printing method. Biomaterials. 2006;27(19): 3580-3588. doi: 10.1016/j.biomaterials.2006.01.048
- Ilkhanizadeh S, Teixeira A, Hermanson O. Inkjet printing of macromolecules on hydrogels to steer neural stem cell differentiation. Biomaterials. 2007;28(27):3936-3943. doi: 10.1016/j.biomaterials.2007.05.018
- Beketov EE, Isaeva VE, Yakovleva ND, et al. Bioprinting of cartilage with bioink based on high-concentration collagen and chondrocytes. Int J Mol Sci. 2021;22(21). doi: 10.3390/ijms222111351
- Zhu J, Marchant RE. Design properties of hydrogel tissue-engineering scaffolds. Expert Rev Med Devices. 2011;8(5):607-626. doi: 10.1586/erd.11.27
- Schuurman W, Levett PA, Pot MW, et al. Gelatin-methacrylamide hydrogels as potential biomaterials for fabrication of tissue-engineered cartilage constructs: gelatin-methacrylamide hydrogels as potential biomaterials for fabrication. Macromol Biosci. 2013;13(5):551-561. doi: 10.1002/mabi.201200471
- Bedell ML, Torres AL, Hogan KJ, et al. Human gelatin-based composite hydrogels for osteochondral tissue engineering and their adaptation into bioinks for extrusion, inkjet, and digital light processing bioprinting. Biofabrication. 2022;14(4). doi: 10.1088/1758-5090/ac8768
- Tang P, Song P, Peng Z, et al. Chondrocyte-laden GelMA hydrogel combined with 3D printed PLA scaffolds for auricle regeneration. Mater Sci Eng C. 2021;130:112423. doi: 10.1016/j.msec.2021.112423
- Sun T, Feng Z, He W, et al. Novel 3D-printing bilayer GelMA-based hydrogel containing BP,β-TCP and exosomes for cartilage-bone integrated repair. Biofabrication. 2023;16(1). doi: 10.1088/1758-5090/ad04fe
- Humenik M, Winkler A, Scheibel T. Patterning of protein‐based materials. Biopolymers. 2021;112(2). doi: 10.1002/bip.23412
- Singh YP, Bandyopadhyay A, Mandal BB. 3D bioprinting using cross-linker-free silk–gelatin bioink for cartilage tissue engineering. ACS Appl Mater Interfaces. 2019;11(37): 33684-33696. doi: 10.1021/acsami.9b11644
- Hong H, Seo YB, Kim DY, et al. Digital light processing 3D printed silk fibroin hydrogel for cartilage tissue engineering. Biomaterials. 2020;232:119679. doi: 10.1016/j.biomaterials.2019.119679
- Gao Q, Kim BS, Gao G. Advanced strategies for 3D bioprinting of tissue and organ analogs using alginate hydrogel bioinks. Marine Drugs. 2021;19(12):708. doi: 10.3390/md19120708
- Drury JL, Mooney DJ. Hydrogels for tissue engineering: scaffold design variables and applications. Biomaterials. 2003;24(24):4337-4351. doi: 10.1016/S0142-9612(03)00340-5
- Sarker B, Zehnder T, Rath SN, et al. Oxidized alginate-gelatin hydrogel: a favorable matrix for growth and osteogenic differentiation of adipose-derived stem cells in 3D. ACS Biomater Sci Eng. 2017;3(8):1730-1737. doi: 10.1021/acsbiomaterials.7b00188
- Ilhan E, Ulag S, Sahin A, et al. Fabrication of tissue-engineered tympanic membrane patches using 3D-Printing technology. J Mech Behav Biomed Mater. 2021;114:104219. doi: 10.1016/j.jmbbm.2020.104219
- Schwarz S, Kuth S, Distler T, et al. 3D printing and characterization of human nasoseptal chondrocytes laden dual crosslinked oxidized alginate-gelatin hydrogels for cartilage repair approaches. Mater Sci Eng C. 2020;116:111189. doi: 10.1016/j.msec.2020.111189
- Olate-Moya F, Arens L, Wilhelm M, Mateos-Timoneda MA, Engel E, Palza H. Chondroinductive alginate-based hydrogels having graphene oxide for 3D printed scaffold fabrication. ACS Appl Mater Interfaces. 2020;12(4):4343-4357. doi: 10.1021/acsami.9b22062
- Moon D, Lee M, Sun J, Song KH, Doh J. Jammed microgel‐based inks for 3D printing of complex structures transformable via pH/temperature variations. Macromol Rapid Commun. 2022;43(19):2200271. doi: 10.1002/marc.202200271
- Hinton TJ, Jallerat Q, Palchesko RN, et al. Three-dimensional printing of complex biological structures by freeform reversible embedding of suspended hydrogels. Sci Adv. 2015;1(9):e1500758. doi: 10.1126/sciadv.1500758
- Gungor-Ozkerim PS, Inci I, Zhang YS, Khademhosseini A, Dokmeci MR. Bioinks for 3D bioprinting: an overview. Biomater Sci. 2018;6(5):915. doi: 10.1039/c7bm00765e
- Guilak F, Cohen DM, Estes BT, Gimble JM, Liedtke W, Chen CS. Control of stem cell fate by physical interactions with the extracellular matrix. Cell Stem Cell. 2009;5(1):17-26. doi: 10.1016/j.stem.2009.06.016
- Derby B. Printing and prototyping of tissues and scaffolds. Science. 2012;338(6109):921-926. doi: 10.1126/science.1226340
- Shanto PC, Park S, Park M, Lee BT. Physico-biological evaluation of 3D printed dECM/TOCN/alginate hydrogel based scaffolds for cartilage tissue regeneration. Biomater Adv. 2023;145:213239. doi: 10.1016/j.bioadv.2022.213239
- Yeleswarapu S, Chameettachal S, Pati F. Integrated 3D printing-based framework—a strategy to fabricate tubular structures with mechanocompromised hydrogels. ACS Appl Biomater. 2021;4(9):6982-6992. doi: 10.1021/acsabm.1c00644
- Brown M, Zhu S, Taylor L, Tabrizian M, Li-Jessen NYK. Unraveling the relevance of tissue‐specific decellularized extracellular matrix hydrogels for vocal fold regenerative biomaterials: a comprehensive proteomic and in vitro study. Adv Nanobiomed Res. 2023;3(4):2200095. doi: 10.1002/anbr.202200095
- Bichara DA, O’Sullivan NA, Pomerantseva I, et al. The tissue-engineered auricle: past, present, and future. Tissue Eng Part B Rev. 2012;18(1):51-61. doi: 10.1089/ten.teb.2011.0326
- Jang CH, Koo Y, Kim G. ASC/chondrocyte-laden alginate hydrogel/PCL hybrid scaffold fabricated using 3D printing for auricle regeneration. Carbohydr Polym. 2020;248:116776. doi: 10.1016/j.carbpol.2020.116776
- Cooke ME, Ramirez-GarciaLuna JL, Rangel-Berridi K, et al. 3D printed polyurethane scaffolds for the repair of bone defects. Front Bioeng Biotechnol. 2020;8:557215. doi: 10.3389/fbioe.2020.557215
- Wen YT, Dai NT, Hsu S hui. Biodegradable water-based polyurethane scaffolds with a sequential release function for cell-free cartilage tissue engineering. Acta Biomater. 2019;88:301-313. doi: 10.1016/j.actbio.2019.02.044
- Li S, Tallia F, Mohammed AA, Stevens MM, Jones JR. Scaffold channel size influences stem cell differentiation pathway in 3-D printed silica hybrid scaffolds for cartilage regeneration. Biomater Sci. 2020;8(16):4458-4466. doi: 10.1039/C9BM01829H
- Park JH, Ahn M, Park SH, et al. 3D bioprinting of a trachea-mimetic cellular construct of a clinically relevant size. Biomaterials. 2021;279:121246. doi: 10.1016/j.biomaterials.2021.121246
- Heuer RA, Nella KT, Chang HT, et al. Three-dimensional otic neuronal progenitor spheroids derived from human embryonic stem cells. Tissue Eng Part A. 2021;27(3-4): 256-269. doi: 10.1089/ten.tea.2020.0078
- Kurihara S, Fujioka M, Hirabayashi M, et al. Otic organoids containing spiral ganglion neuron-like cells derived from human-induced pluripotent stem cells as a model of drug-induced neuropathy. Stem Cells Transl Med. 2022;11(3): 282-296. doi: 10.1093/stcltm/szab023
- Das S, Pati F, Choi YJ, et al. Bioprintable, cell-laden silk fibroin-gelatin hydrogel supporting multilineage differentiation of stem cells for fabrication of three-dimensional tissue constructs. Acta Biomater. 2015;11: 233-246. doi: 10.1016/j.actbio.2014.09.023
- Zhang C, Wang G, Lin H, et al. Cartilage 3D bioprinting for rhinoplasty using adipose-derived stem cells as seed cells: review and recent advances. Cell Prolif. 2023;56(4):e13417. doi: 10.1111/cpr.13417
- Lee J, Hong J, Kim W, Kim GH. Bone-derived dECM/ alginate bioink for fabricating a 3D cell-laden mesh structure for bone tissue engineering. Carbohydr Polym. 2020;250:116914. doi: 10.1016/j.carbpol.2020.116914
- Bae SW, Lee KW, Park JH, et al. 3D bioprinted artificial trachea with epithelial cells and chondrogenic-differentiated bone marrow-derived mesenchymal stem cells. IJMS. 2018;19(6):1624. doi: 10.3390/ijms19061624
- Csobonyeiova M, Polak S, Zamborsky R, Danisovic L. iPS cell technologies and their prospect for bone regeneration and disease modeling: a mini review. J Adv Res. 2017;8(4):321-327. doi: 10.1016/j.jare.2017.02.004
- Liang L, Li Z, Yao B, et al. Extrusion bioprinting of cellular aggregates improves mesenchymal stem cell proliferation and differentiation. Biomater Adv. 2023;149:213369. doi: 10.1016/j.bioadv.2023.213369
- Gantumur E, Nakahata M, Kojima M, Sakai S. Extrusion-based bioprinting through glucose-mediated enzymatic hydrogelation. Int J Bioprint. 2020;6(1):250. doi: 10.18063/ijb.v6i1.250
- Lee HJ, Kim YB, Ahn SH, et al. A new approach for fabricating collagen/ECM-based bioinks using preosteoblasts and human adipose stem cells. Adv Healthc Mater. 2015;4(9):1359-1368. doi: 10.1002/adhm.201500193
- Faramarzi N, Yazdi IK, Nabavinia M, et al. Patient-specific bioinks for 3D bioprinting of tissue engineering scaffolds. Adv Healthc Mater. 2018;7(11):1701347. doi: 10.1002/adhm.201701347
- Yu K, Zhang X, Sun Y, et al. Printability during projection-based 3D bioprinting. Bioact Mater. 2022;11:254-267. doi: 10.1016/j.bioactmat.2021.09.021
- He Y, Yang F, Zhao H, Gao Q, Xia B, Fu J. Research on the printability of hydrogels in 3D bioprinting. Sci Rep. 2016;6(1):29977. doi: 10.1038/srep29977
- Kryou C, Theodorakos I, Karakaidos P, Klinakis A, Hatziapostolou A, Zergioti I. Parametric study of jet/droplet formation process during LIFT printing of living cell-laden bioink. Micromachines. 2021;12(11). doi: 10.3390/mi12111408
- Gopinathan J, Noh I. Recent trends in bioinks for 3D printing. Biomater Res. 2018;22(1):11. doi: 10.1186/s40824-018-0122-1
- Hölzl K, Lin S, Tytgat L, Van Vlierberghe S, Gu L, Ovsianikov A. Bioink properties before, during and after 3D bioprinting. Biofabrication. 2016;8(3):032002. doi: 10.1088/1758-5090/8/3/032002
- Ozbolat IT, Peng W, Ozbolat V. Application areas of 3D bioprinting. Drug Discov Today. 2016;21(8):1257-1271. doi: 10.1016/j.drudis.2016.04.006
- Lee JS, Kim BS, Seo D, Park JH, Cho DW. Three-dimensional cell printing of large-volume tissues: application to ear regeneration. Tissue Eng Part C Methods. 2017;23(3):136-145. doi: 10.1089/ten.tec.2016.0362
- Ouyang L, Yao R, Zhao Y, Sun W. Effect of bioink properties on printability and cell viability for 3D bioplotting of embryonic stem cells. Biofabrication. 2016;8(3):035020. doi: 10.1088/1758-5090/8/3/035020
- Chanlalit C, Shukla DR, Fitzsimmons JS, An KN, O’Driscoll SW. Stress shielding around radial head prostheses. J Hand Surg Am. 2012;37(10):2118-2125. doi: 10.1016/j.jhsa.2012.06.020
- Kim UJ, Park J, Joo Kim H, Wada M, Kaplan DL. Three-dimensional aqueous-derived biomaterial scaffolds from silk fibroin. Biomaterials. 2005;26(15):2775-2785. doi: 10.1016/j.biomaterials.2004.07.044
- Wenk E, Merkle HP, Meinel L. Silk fibroin as a vehicle for drug delivery applications. J Control Release. 2011;150(2): 128-141. doi: 10.1016/j.jconrel.2010.11.007
- Bradner SA, Galaiya D, Raol N, Kaplan DL, Hartnick CJ. Silk protein bioresorbable, drug-eluting ear tubes: proof-of-concept. Adv Healthc Mater. 2019;8(3):1801409. doi: 10.1002/adhm.201801409
- Williams DF. The language of biomaterials-based technologies. Regen Eng Transl Med. 2019;5(1):53-60. doi: 10.1007/s40883-018-0088-5
- Winkler S, Meyer KV, Heuer C, Kortmann C, Dehne M, Bahnemann J. In vitro biocompatibility evaluation of a heat‐resistant 3D printing material for use in customized cell culture devices. Eng Life Sci. 2022;22(11):699-708. doi: 10.1002/elsc.202100104
- Bernard M, Jubeli E, Pungente MD, Yagoubi N. Biocompatibility of polymer-based biomaterials and medical devices – regulations, in vitro screening and risk-management. Biomater Sci. 2018;6(8):2025-2053. doi: 10.1039/C8BM00518D
- Yan Y, Chen H, Zhang H, et al. Vascularized 3D printed scaffolds for promoting bone regeneration. Biomaterials. 2019;190-191:97-110. doi: 10.1016/j.biomaterials.2018.10.033
- Heikkinen AK, Lähde S, Rissanen V, et al. Feasibility of 3D-printed middle ear prostheses in partial ossicular chain reconstruction. Int J Bioprint. 2023;9(4):727. doi: 10.18063/ijb.727
- Ueda Y, Nakamura T, Nie J, et al. Defining developmental trajectories of prosensory cells in human inner ear organoids at single-cell resolution. Development. 2023;150(12):dev201071. doi: 10.1242/dev.201071
- Osaki D, Ouji Y, Sakagami M, et al. Culture of organoids with vestibular cell-derived factors promotes differentiation of embryonic stem cells into inner ear vestibular hair cells. J Biosci Bioeng. 2023;135(2):143-150. doi: 10.1016/j.jbiosc.2022.11.005
- Dong Q, Su X, Li X, et al. In vitro construction of lung cancer organoids by 3D bioprinting for drug evaluation. Colloids Surf A. 2023;666. doi: 10.1016/j.colsurfa.2023.131288
- Salmon I, Grebenyuk S, Abdel Fattah AR, et al. Engineering neurovascular organoids with 3D printed microfluidic chips. Lab Chip. 2022;22(8):1615-1629. doi: 10.1039/d1lc00535a
- Ungar OJ. Letter to the Editor regarding “Single-sided deafness after sudden hearing loss: late effect on cochlear nerve size” by Islamoglu et al. European Archives of Oto- Rhino-Laryngology (2020) 277:2423-2426. Eur Arch Otorhinolaryngol. 2020;277(11). doi: 10.1007/s00405-020-06304-0
- Kim T, Yi Q, Hoang E, Esfandyarpour R. A 3D printed wearable bioelectronic patch for multi-sensing and in situ sweat electrolyte monitoring. Adv Mater Technol. 2021;6(4). doi: 10.1002/admt.202001021
- Sandstrom CG. The non-disruptive emergence of an ecosystem for 3D printing - insights from the hearing aid industry’s transition 1989-2008. Technol Forecast Soc Change. 2016;102:160-168. doi: 10.1016/j.techfore.2015.09.006
- Fu F, Luximon Y. Fit and comfort perception on hearing aids: a pilot study. In: Ahram T, Karwowski W, Pickl S, Taiar R, eds. Human Systems Engineering and Design II. Cham: Springer International Publishing; 2020:360-364. doi: 10.1007/978-3-030-27928-8_55
- Vivero-Lopez M, Xu X, Muras A, et al. Anti-biofilm multi drug-loaded 3D printed hearing aids. Mater Sci Eng C Mater Biol Appl. 2021;119:111606. doi: 10.1016/j.msec.2020.111606
- Famm K, Litt B, Tracey KJ, Boyden ES, Slaoui M. Drug discovery: a jump-start for electroceuticals. Nature. 2013;496(7444):159-161. doi: 10.1038/496159a
- Birmingham K, Gradinaru V, Anikeeva P, et al. Bioelectronic medicines: a research roadmap. Nat Rev Drug Discov. 2014;13(6):399-400. doi: 10.1038/nrd4351
- Sarreal RR, Bhatti P. Characterization and miniaturization of silver-nanoparticle microcoil via aerosol jet printing techniques for micromagnetic cochlear stimulation. Sensors (Basel). 2020;20(21):6087. doi: 10.3390/s20216087
- Lei IM, Jiang C, Lei CL, et al. 3D printed biomimetic cochleae and machine learning co-modelling provides clinical informatics for cochlear implant patients. Nat Commun. 2021;12(1):6260. doi: 10.1038/s41467-021-26491-6
- Lan X, Liang Y, Vyhlidal M, et al. In vitro maturation and in vivo stability of bioprinted human nasal cartilage. J Tissue Eng. 2022;13:20417314221086368. doi: 10.1177/20417314221086368
- Nuseir A, Hatamleh MM, Alnazzawi A, Al-Rabab’ah M, Kamel B, Jaradat E. Direct 3D printing of flexible nasal prosthesis: optimized digital workflow from scan to fit. J Prosthodont. 2019;28(1):10-14. doi: 10.1111/jopr.13001
- Chiesa-Estomba CM, González-García J, Sistiaga-Suarez JA, González Fernández I. A novel computer-aided design/computer-aided manufacturing (CAD/CAM) 3D printing method for nasal framework reconstruction using microvascular free flaps. Cureus. 2022;14(9): e28971. doi: 10.7759/cureus.28971
- Heikkinen AK, Lähde S, Rissanen V, et al. Feasibility of 3D-printed middle ear prostheses in partial ossicular chain reconstruction. Int J Bioprint. 2023;9(4):727. doi: 10.18063/ijb.727
- Ueda Y, Nakamura T, Nie J, et al. Defining developmental trajectories of prosensory cells in human inner ear organoids at single-cell resolution. Development. 2023;150(12):dev201071. doi: 10.1242/dev.201071
- Osaki D, Ouji Y, Sakagami M, et al. Culture of organoids with vestibular cell-derived factors promotes differentiation of embryonic stem cells into inner ear vestibular hair cells. J Biosci Bioeng. 2023;135(2):143-150. doi: 10.1016/j.jbiosc.2022.11.005
- Dong Q, Su X, Li X, et al. In vitro construction of lung cancer organoids by 3D bioprinting for drug evaluation. Colloids Surf A. 2023;666. doi: 10.1016/j.colsurfa.2023.131288
- Salmon I, Grebenyuk S, Abdel Fattah AR, et al. Engineering neurovascular organoids with 3D printed microfluidic chips. Lab Chip. 2022;22(8):1615-1629. doi: 10.1039/d1lc00535a
- Ungar OJ. Letter to the Editor regarding “Single-sided deafness after sudden hearing loss: late effect on cochlear nerve size” by Islamoglu et al. European Archives of Oto- Rhino-Laryngology (2020) 277:2423-2426. Eur Arch Otorhinolaryngol. 2020;277(11). doi: 10.1007/s00405-020-06304-0
- Kim T, Yi Q, Hoang E, Esfandyarpour R. A 3D printed wearable bioelectronic patch for multi-sensing and in situ sweat electrolyte monitoring. Adv Mater Technol. 2021;6(4). doi: 10.1002/admt.202001021
- Sandstrom CG. The non-disruptive emergence of an ecosystem for 3D printing - insights from the hearing aid industry’s transition 1989-2008. Technol Forecast Soc Change. 2016;102:160-168. doi: 10.1016/j.techfore.2015.09.006
- Fu F, Luximon Y. Fit and comfort perception on hearing aids: a pilot study. In: Ahram T, Karwowski W, Pickl S, Taiar R, eds. Human Systems Engineering and Design II. Cham: Springer International Publishing; 2020:360-364. doi: 10.1007/978-3-030-27928-8_55
- Vivero-Lopez M, Xu X, Muras A, et al. Anti-biofilm multi drug-loaded 3D printed hearing aids. Mater Sci Eng C Mater Biol Appl. 2021;119:111606. doi: 10.1016/j.msec.2020.111606
- Famm K, Litt B, Tracey KJ, Boyden ES, Slaoui M. Drug discovery: a jump-start for electroceuticals. Nature. 2013;496(7444):159-161. doi: 10.1038/496159a
- Birmingham K, Gradinaru V, Anikeeva P, et al. Bioelectronic medicines: a research roadmap. Nat Rev Drug Discov. 2014;13(6):399-400. doi: 10.1038/nrd4351
- Sarreal RR, Bhatti P. Characterization and miniaturization of silver-nanoparticle microcoil via aerosol jet printing techniques for micromagnetic cochlear stimulation. Sensors (Basel). 2020;20(21):6087. doi: 10.3390/s20216087
- Lei IM, Jiang C, Lei CL, et al. 3D printed biomimetic cochleae and machine learning co-modelling provides clinical informatics for cochlear implant patients. Nat Commun. 2021;12(1):6260. doi: 10.1038/s41467-021-26491-6
- Lan X, Liang Y, Vyhlidal M, et al. In vitro maturation and in vivo stability of bioprinted human nasal cartilage. J Tissue Eng. 2022;13:20417314221086368. doi: 10.1177/20417314221086368
- Nuseir A, Hatamleh MM, Alnazzawi A, Al-Rabab’ah M, Kamel B, Jaradat E. Direct 3D printing of flexible nasal prosthesis: optimized digital workflow from scan to fit. J Prosthodont. 2019;28(1):10-14. doi: 10.1111/jopr.13001
- Chiesa-Estomba CM, González-García J, Sistiaga-Suarez JA, González Fernández I. A novel computer-aided design/computer-aided manufacturing (CAD/CAM) 3D printing method for nasal framework reconstruction using microvascular free flaps. Cureus. 2022;14(9): e28971. doi: 10.7759/cureus.28971
- Yi HG, Choi YJ, Jung JW, et al. Three-dimensional printing of a patient-specific engineered nasal cartilage for augmentative rhinoplasty. J Tissue Eng. 2019;10:2041731418824797. doi: 10.1177/2041731418824797
- Luo D, Li T, Wang H, Chen Y. Three-dimensional printing of personalized nasal stents for patients with cleft lip. Cleft Palate Craniofac J. 2019;56(4):521-524. doi: 10.1177/1055665618782804
- Jung JW, Ha DH, Kim BY, et al. Nasal reconstruction using a customized three-dimensional-printed stent for congenital arhinia: three-year follow-up: nasal reconstruction using a 3D-printed stent. Laryngoscope. 2019;129(3):582-585. doi: 10.1002/lary.27335
- Lee AWM, Ma BBY, Ng WT, Chan ATC. Management of nasopharyngeal carcinoma: current practice and future perspective. J Clin Oncol. 2015;33(29):3356-3364. doi: 10.1200/JCO.2015.60.9347
- Ding RB, Chen P, Rajendran BK, et al. Molecular landscape and subtype-specific therapeutic response of nasopharyngeal carcinoma revealed by integrative pharmacogenomics. Nat Commun. 2021;12(1):3046. doi: 10.1038/s41467-021-23379-3
- Lucky SS, Law M, Lui MH, et al. Patient-derived nasopharyngeal cancer organoids for disease modeling and radiation dose optimization. Front Oncol. 2021;11:622244. doi: 10.3389/fonc.2021.622244
- Wang XW, Xia TL, Tang HC, et al. Establishment of a patient-derived organoid model and living biobank for nasopharyngeal carcinoma. Ann Transl Med. 2022;10(9):526. doi: 10.21037/atm-22-1076
- Park W, Bae M, Hwang M, Jang J, Cho DW, Yi HG. 3D cell-printed hypoxic cancer-on-a-chip for recapitulating pathologic progression of solid cancer. J Vis Exp. 2021;(167). doi: 10.3791/61945
- Kankala RK, Wang SB, Chen AZ. Microengineered organ-on-a-chip platforms towards personalized medicine. Curr Pharm Des. 2018;24(45):5354-5366. doi: 10.2174/1381612825666190222143542
- Liu Z, Zhang W, Pang SW. Migration of immortalized nasopharyngeal epithelia and carcinoma cells through porous membrane in 3D platforms. Biosci Rep. 2020;40(6):BSR20194113. doi: 10.1042/BSR20194113
- Zhang WG, Liu ZY, Pang SW. Separation of nasopharyngeal epithelial cells from carcinoma cells on 3D scaffold platforms. Biotechnol Bioeng. 2021;118(4):1444-1455. doi: 10.1002/bit.27640
- Shen Z, Xie Y, Shang X, et al. The manufacturing procedure of 3D printed models for endoscopic endonasal transsphenoidal pituitary surgery. Technol Health Care. 2020;28(S1):131-150. doi: 10.3233/THC-209014
- Huang X, Fan N, Wang HJ, Zhou Y, Li X, Jiang XB. Application of 3D printed model for planning the endoscopic endonasal transsphenoidal surgery. Sci Rep. 2021;11(1):5333. doi: 10.1038/s41598-021-84779-5
- Moon JH, Kim EH, Kim SH. Various modifications of a vascularized nasoseptal flap for repair of extensive skull base dural defects. J Neurosurg. 2019;132(2):371-379. doi: 10.3171/2018.10.JNS181556
- Kayastha D, Wiznia D, Manes RP, Omay SB, Khoury T, Rimmer R. 3D printing for virtual surgical planning of nasoseptal flap skull-base reconstruction: a proof-of-concept study. Int Forum Allergy Rhinol. 2023;13(11):2073-2075. doi: 10.1002/alr.23165
- Deruyver L, Rigaut C, Lambert P, Haut B, Goole J. The importance of pre-formulation studies and of 3D-printed nasal casts in the success of a pharmaceutical product intended for nose-to-brain delivery. Adv Drug Deliv Rev. 2021;175:113826. doi: 10.1016/j.addr.2021.113826 16/j.celrep.2018.12.090
- Goyanes A, Det-Amornrat U, Wang J, Basit AW, Gaisford S. 3D scanning and 3D printing as innovative technologies for fabricating personalized topical drug delivery systems. J Control Release. 2016;234:41-48. doi: 10.1016/j.jconrel.2016.05.034
- Djupesland PG, Messina JC, Palmer JN. Deposition of drugs in the nose and sinuses with an exhalation delivery system vs conventional nasal spray or high-volume irrigation in Draf II/ III post-surgical anatomy. Rhinology. 2020;58(2):175-183. doi: 10.4193/Rhin18.304
- Ciavarella D, Campobasso A, Conte E, et al. Correlation between dental arch form and OSA severity in adult patients: an observational study. Prog Orthod. 2023;24(1):19. doi: 10.1186/s40510-023-00464-5
- Kecik D. Three-dimensional analyses of palatal morphology and its relation to upper airway area in obstructive sleep apnea. Angle Orthod. 2017;87(2):300-306. doi: 10.2319/051116-377.1
- Chi AC, Day TA, Neville BW. Oral cavity and oropharyngeal squamous cell carcinoma--an update. CA Cancer J Clin. 2015;65(5):401-421. doi: 10.3322/caac.21293
- Xu J, Lai F, Liu Y, et al. Novel computer-aided reconstruction of soft tissue defects following resection of oral and oropharyngeal squamous cell carcinoma. World J Surg Oncol. 2022;20(1):196. doi: 10.1186/s12957-022-02654-7
- Bhattacharyya A, Janarthanan G, Kim T, et al. Modulation of bioactive calcium phosphate micro/nanoparticle size and shape during in situ synthesis of photo-crosslinkable gelatin methacryloyl based nanocomposite hydrogels for 3D bioprinting and tissue engineering. Biomater Res. 2022;26(1):54. doi: 10.1186/s40824-022-00301-6
- Macielak RJ, Ziebarth MT, Price DL. 3D printed fistula plug: a novel bridge to definitive reconstruction. Laryngoscope. 2021;131(1):111-114. doi: 10.1002/lary.28563
- Hu Q, Cui J, Zhang H, Liu S, Ramalingam M. A 5 + 1-axis 3D printing platform for producing customized intestinal fistula stents. 3D Print Addit Manuf. 2023;10(5):955-970. doi: 10.1089/3dp.2021.0044
- Nyirjesy SC, Judd RT, Alfayez Y, et al. Use of 3-dimensional printing at the point-of-care to manage a complex wound in hemifacial necrotizing fasciitis: a case report. 3D Print Med. 2023;9(1):4. doi: 10.1186/s41205-022-00166-4
- Arens C, Schwemmle C, Voigt-Zimmermann S. Surgical reconstruction in laryngeal carcinoma. HNO. 2020;68(9):666-677. doi: 10.1007/s00106-020-00916-y
- Kahmke R, Sajisevi M. Larynx cancer: reconstructive options. Otolaryngol Clin North Am. 2023;56(2):333-343. doi: 10.1016/j.otc.2022.11.002
- Tian H, Gao S, Yu J, et al. Application of digital modeling and three-dimensional printing of titanium mesh for reconstruction of thyroid cartilage in partial laryngectomy. Acta Otolaryngol. 2022;142(3-4):363-368. doi: 10.1080/00016489.2022.2055138
- Celik H, Krug E, Zhang CR, et al. A humanized animal model predicts clonal evolution and therapeutic vulnerabilities in myeloproliferative neoplasms. Cancer Discov. 2021;11(12):3126-3141. doi: 10.1158/2159-8290.CD-20-1652
- Fan H, Demirci U, Chen P. Emerging organoid models: leaping forward in cancer research. J Hematol Oncol. 2019;12(1):142. doi: 10.1186/s13045-019-0832-4
- Almela T, Tayebi L, Moharamzadeh K. 3D bioprinting for in vitro models of oral cancer: toward development and validation. Bioprinting. 2021;22:e00132. doi: 10.1016/j.bprint.2021.e00132
- Langer EM, Allen-Petersen BL, King SM, et al. Modeling tumor phenotypes in vitro with three-dimensional bioprinting. Cell Rep. 2019;26(3):608-623.e6. doi: 10.1016/j.celrep.2018.12.090
- Vimawala S, Gao T, Goldfarb J, et al. Initial experience using 3-dimensional printed models for head and neck reconstruction in Haiti. Ear Nose Throat J. 2022;101(3): NP89-NP91. doi: 10.1177/0145561320938920
- Richard Z, Jackson E, Jung JP, Kanotra SP. Feasibility and potential of three-dimensional printing in laryngotracheal stenosis. J Laryngol Otol. 2019;133(6):530-534. doi: 10.1017/S0022215119001208
- Furlow PW, Mathisen DJ. Surgical anatomy of the trachea. Ann Cardiothorac Surg. 2018;7(2):255-260. doi: 10.21037/acs.2018.03.01
- Sun Y, Huo Y, Ran X, et al. Instant trachea reconstruction using 3D-bioprinted C-shape biomimetic trachea based on tissue-specific matrix hydrogels. Bioact Mater. 2024;32: 52-65. doi: 10.1016/j.bioactmat.2023.09.011
- Huo Y, Xu Y, Wu X, et al. Functional trachea reconstruction using 3D-bioprinted native-like tissue architecture based on designable tissue-specific bioinks. Adv Sci (Weinh). 2022;9(29):e2202181. doi: 10.1002/advs.202202181
- Gao B, Jing H, Gao M, et al. Long-segmental tracheal reconstruction in rabbits with pedicled tissue-engineered trachea based on a 3D-printed scaffold. Acta Biomater. 2019;97:177-186. doi: 10.1016/j.actbio.2019.07.043
- Weber JF, Rehmani SS, Baig MZ, et al. Novel composite trachea grafts using 3-dimensional printing. JTCVS Open. 2021;5:152-160. doi: 10.1016/j.xjon.2020.11.001
- Tsai AY, Moroi MK, Les AS, et al. Tracheal agenesis: esophageal airway support with a 3-dimensional-printed bioresorbable splint. JTCVS Tech. 2021;10:563-568. doi: 10.1016/j.xjtc.2021.08.037
- Yu D, Peng W, Mo X, Zhang Y, Zhang X, He J. Personalized 3D-printed bioresorbable airway external splint for tracheomalacia combined with congenital heart disease. Front Bioeng Biotechnol. 2022;10:859777. doi: 10.3389/fbioe.2022.859777
- Greenwood TE, Thomson SL. Embedded 3D printing of multi-layer, self-oscillating vocal fold models. J Biomech. 2021;121:110388. doi: 10.1016/j.jbiomech.2021.110388
- Romero RGT, Colton MB, Thomson SL. 3D-printed synthetic vocal fold models. J Voice. 2021;35(5):685-694. doi: 10.1016/j.jvoice.2020.01.030
- Tiwari D, Vobilisetty RK, Heer B. Current application and future prospects of 3D printing in otorhinolaryngology-a narrative review. Indian J Otolaryngol Head Neck Surg. 2022;74(1):123-126. doi: 10.1007/s12070-021-02634-5
- Gao Q, Niu X, Shao L, et al. 3D printing of complex GelMA-based scaffolds with nanoclay. Biofabrication. 2019;11(3):035006. doi: 10.1088/1758-5090/ab0cf6
- Mihankhah P, Azdast T, Mohammadzadeh H, Hasanzadeh R, Aghaiee S. Fused filament fabrication of biodegradable polylactic acid reinforced by nanoclay as a potential biomedical material. J Thermoplast Compos Mater. 2023;36(3):961-983. doi: 10.1177/08927057211044185
- Zheng F, Xiao Y, Liu H, Fan Y, Dao M. Patient-specific organoid and organ-on-a-chip: 3D cell-culture meets 3D printing and numerical simulation. Adv Biol (Weinh). 2021;5(6):e2000024. doi: 10.1002/adbi.202000024
- Velasco V, Shariati SA, Esfandyarpour R. Microtechnology-based methods for organoid models. Microsyst Nanoeng. 2020;6:76. doi: 10.1038/s41378-020-00185-3
- Morrison RJ, Sengupta S, Flanangan CL, Ohye RG, Hollister SJ, Green GE. Treatment of severe acquired tracheomalacia with a patient-specific, 3D-printed, permanent tracheal splint. JAMA Otolaryngol Head Neck Surg. 2017;143(5):523-525. doi: 10.1001/jamaoto.2016.3932
- Goyanes A, Robles Martinez P, Buanz A, Basit AW, Gaisford S. Effect of geometry on drug release from 3D printed tablets. Int J Pharm. 2015;494(2):657-663. doi: 10.1016/j.ijpharm.2015.04.069
- Norman J, Madurawe RD, Moore CMV, Khan MA, Khairuzzaman A. A new chapter in pharmaceutical manufacturing: 3D-printed drug products. Adv Drug Deliv Rev. 2017;108:39-50. doi: 10.1016/j.addr.2016.03.001