Current advances of 3D-bioprinted microfluidic models with hydrogel bioinks and their applications in drug screening
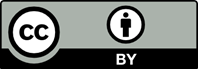
Microenvironments of tumor and organ models dictate the accuracy of drug screening results. The advancement of technologies and hydrogel bioinks has significantly increased the representation of tumor and organs models in the armada of drug testing tools. Hydrogel bioinks, characterized by their high water content and efficient substance transport, facilitate the reconstruction of human tissues by acting as functional carriers for cells. The molding and cell culture function of hydrogels are preserved and optimized through rational engineering techniques. Furthermore, previous studies have often focused on fabrication of supporting constructs by means of three-dimensional (3D) bioprinting or microfluidic technology for dynamic cultures. Nevertheless, the combination of bioprinting and microfluidic technologies offers advantages in terms of dynamic response and automation, which enable the creation of artificial tumor or organ models to represent actual microenvironments. In this review, we discuss the components and physical features of tumor microenvironments (TMEs), most of which have been reproduced in artificial models widely by different researchers. We also classify bioink-simulating extracellular matrix (ECM) in TMEs, explain their crosslinking principles, and introduce their manifestations, including artificial disease or organ models in tissue engineering application. Technologies, such as 3D bioprinting and microfluidic technology, used to create these models are also outlined. At last, we summarize disease models and organ microarchitectures fabricated by these two technologies and offer application prospects of these models in the realm of precision medicine.
- Dawood AA, Yousif WI. The main reasons why cancer is so difficult to treatment. AJOAIMS. Philadelphia: Elsevier; 2020;2(2):20-22.
- DeBerardinis RJ. Tumor microenvironment, metabolism, and immunotherapy. N Engl J Med. 2020;382(9):869-871. doi: 10.1056/NEJMcibr1914890
- Advancing cancer therapy. Nat Cancer. 2021;2(3):245-246. doi: 10.1038/s43018-021-00192-x
- Bains W. Failure rate in drug discovery and development: will we ever get any better? Drug Discov World. 2004;5.
- Imamura Y, Mukohara T, Shimono Y, et al. Comparison of 2D- and 3D-culture models as drug-testing platforms in breast cancer. Oncol Rep. 2015;33(4):1837-1843. doi: 10.3892/or.2015.3767
- Jensen C, Teng Y. Is it time to start transitioning from 2D to 3D cell culture? Front Mol Biosci. 2020;7:33. doi: 10.3389/fmolb.2020.00033
- de Jong M, Maina T. Of mice and humans: are they the same?--Implications in cancer translational research. J Nucl Med. 2010;51(4):501-504. doi: 10.2967/jnumed.109.065706
- Mak IW, Evaniew N, Ghert M. Lost in translation: animal models and clinical trials in cancer treatment. Am J Transl Res. 2014;6(2):114-118.
- Dey M, Ozbolat IT. 3D bioprinting of cells, tissues and organs. Sci Rep. 2020;10:14023. doi: 10.1038/s41598-020-70086-y
- Staros R, Michalak A, Rusinek K, Mucha K, Pojda Z, Zagożdżon R. Perspectives for 3D-bioprinting in modeling of tumor immune evasion. Cancers (Basel). 2022;14(13):3126. doi: 10.3390/cancers14133126
- Xie F, Sun L, Pang Y, et al. Three-dimensional bio-printing of primary human hepatocellular carcinoma for personalized medicine. Biomaterials. 2021;265:120416. doi: 10.1016/j.biomaterials.2020.120416
- Jia X, Yang X, Luo G, Liang Q. Recent progress of microfluidic technology for pharmaceutical analysis. J Pharm Biomed Anal. 2022;209:114534. doi: 10.1016/j.jpba.2021.114534
- Yu F, Choudhury D. Microfluidic bioprinting for organ-on-a-chip models. Drug Discov Today. 2019;24(6):1248-1257. doi: 10.1016/j.drudis.2019.03.025
- Tiwari AP, Thorat ND, Pricl S, Patil RM, Rohiwal S, Townley H. Bioink: a 3D-bioprinting tool for anticancer drug discovery and cancer management. Drug Discov Today. 2021;26(7):1574-1590. doi: 10.1016/j.drudis.2021.03.010
- Ji S, Guvendiren M. Recent advances in bioink design for 3D bioprinting of tissues and organs. Front Bioeng Biotechnol. 2017;5:23. doi: 10.3389/fbioe.2017.00023
- Nejman D, Livyatan I, Fuks G, et al. The human tumor microbiome is composed of tumor type-specific intracellular bacteria. Science. 2020;368(6494):973-980. doi: 10.1126/science.aay9189
- Galeano Niño JL, Wu H, LaCourse KD, et al. Effect of the intratumoral microbiota on spatial and cellular heterogeneity in cancer. Nature. 2022;611(7937):810-817. doi: 10.1038/s41586-022-05435-0
- Bejarano L, Jordāo MJC, Joyce JA. Therapeutic targeting of the tumor microenvironment. Cancer Discov. 2021;11(4):933-959. doi: 10.1158/2159-8290
- Marconi GD, Fonticoli L, Rajan TS, et al. Epithelial-mesenchymal transition (EMT): the type-2 EMT in wound healing, tissue regeneration and organ fibrosis. Cells. 2021;10(7):1587. doi: 10.3390/cells10071587
- Huang H, Wang Z, Zhang Y, et al. Mesothelial cell-derived antigen-presenting cancer-associated fibroblasts induce expansion of regulatory T cells in pancreatic cancer. Cancer Cell. 2022;40(6):656-673. doi: 10.1016/j.ccell.2022.04.011
- Kalluri R, Zeisberg M. Fibroblasts in cancer. Nat Rev Cancer. 2006;6(5):392-401. doi: 10.1038/nrc1877
- Kobayashi H, Enomoto A, Woods SL, Burt AD, Takahashi M, Worthley DL. Cancer-associated fibroblasts in gastrointestinal cancer. Nat Rev Gastroenterol Hepatol. 2019;16(5):282-295. doi: 10.1038/s41575-019-0115-0
- Nurmik M, Ullmann P, Rodriguez F, Haan S, Letellier E. In search of definitions: cancer-associated fibroblasts and their markers. Int J Cancer. 2020;146(4):895-905. doi: 10.1002/ijc.32193
- Chen X, Song. Turning foes to friends: targeting cancer-associated fibroblasts. Nat Rev Drug Discov. 2019;18(2):99-115. doi: 10.1038/s41573-018-0004-1
- Wu K, Lin K, Li X, et al. Redefining tumor-associated macrophage subpopulations and functions in the tumor microenvironment. Front Immunol. 2020;11:1731. doi: 10.3389/fimmu.2020.01731
- Pan Y, Yu Y, Wang X, Zhang T. Tumor-associated macrophages in tumor immunity. Front Immunol. 2020;11:583084. doi: 10.3389/fimmu.2020.583084
- Fukuda K, Kobayashi A, Watabe K. The role of tumor-associated macrophage in tumor progression. Front Biosci. 2012;4(2):787-798. doi: 10.2741/s299
- Qian BZ, Pollard JW. Macrophage diversity enhances tumor progression and metastasis. Cell. 2010;141(1):39-51. doi: 10.1016/j.cell.2010.03.014
- Melaiu O, Lucarini V, Cifaldi L, Fruci D. Influence of the tumor microenvironment on NK cell function in solid tumors. Front Immunol. 2020;10:3038. doi: 10.3389/fimmu.2019.03038
- Xia L, Oyang L, Lin J, et al. The cancer metabolic reprogramming and immune response. Mol Cancer. 2021;20(1):28. doi: 10.1186/s12943-021-01316-8
- Greer SN, Metcalf JL, Wang Y, Ohh M. The updated biology of hypoxia-inducible factor. EMBO J. 2012;31(11):2448-2460. doi: 10.1038/emboj.2012.125
- Jiang Z, Zhou J, Li L, et al. Pericytes in the tumor microenvironment. Cancer Lett. 2023;216074. doi: 10.1016/j.canlet.2023.216074
- Li Y, Zhao L, Li XF. Hypoxia and the tumor microenvironment. Technol Cancer Res Treat. 2021;20:15330338211036304. doi: 10.1177/15330338211036304
- Hanahan D, Weinberg RA. Hallmarks of cancer: the next generation. Cell. 2011;144(5):646-674. doi: 10.1016/j.cell.2011.02.013
- Meirson T, Gil-Henn H, Samson AO. Invasion and metastasis: the elusive hallmark of cancer. Oncogene. 2020;39(9):2024-2026. doi: 10.1038/s41388-019-1110-1
- Majidpoor J, Mortezaee K. Steps in metastasis: an updated review. Med Oncol. 2021;38(1):3. doi: 10.1007/s12032-020-01447-w
- Duffy MJ, McGowan PM, Gallagher WM. Cancer invasion and metastasis: changing views. J Pathol. 2008;214(3):283-293. doi: 10.1002/path.2282
- Jain RK, Martin JD, Chauhan VP, Duda DG. Tumor microenvironment: vascular and extravascular compartment. In: Niederhuber JE, Armitage JO, Kastan MB, Doroshow JH, Tepper JE, eds. Abeloff’s Clinical Oncology. 6th ed. Elsevier; 2020:108-126. doi: 10.1016/B978-0-323-47674-4.00008-6
- Ribatti D, Pezzella F. Overview on the different patterns of tumor vascularization. Cells. 2021;10(3):639. doi: 10.3390/cells10030639
- NajafiM, Goradel NH, Farhood B, et al. Tumor microenvironment: interactions and therapy. J Cell Physiol. 2019;234(5):5700-5721. doi: 10.1002/jcp.27425
- Farhood B, Najafi M, Mortezaee K. Cancer-associated fibroblasts: secretions, interactions, and therapy. J Cell Biochem. 2019;120(3):2791-2800. doi: 10.1002/jcb.27703
- Jeong SY, Lee JH, Shin Y, Chung S, Kuh H-J. Co-culture of tumor spheroids and fibroblasts in a collagen matrix-incorporated microfluidic chip mimics reciprocal activation in solid tumor microenvironment. PLoS One. 2016;11(7):e0159013. doi: 10.1371/journal.pone.0159013
- Melcher V, Graf M, Interlandi M, et al. Macrophage-tumor cell interaction promotes ATRT progression and chemoresistance. Acta Neuropathol. 2020;139(5): 913-936. doi: 10.1007/s00401-019-02116-7
- Mortezaee K. Immune escape: a critical hallmark in solid tumors. Life Sci. 2020;258:118110. doi: 10.1016/j.lfs.2020.118110
- Elmusrati A, Wang J, Wang CY. Tumor microenvironment and immune evasion in head and neck squamous cell carcinoma. Int J Oral Sci. 2021;13(1):24. doi: 10.1038/s41368-021-00131-7
- Tang H, Fu YX. Immune evasion in tumor’s own sweet way. Cell Metab. 2018;27(5):945-946. doi: 10.1016/j.cmet.2018.03.013
- Shay JW. Role of telomeres and telomerase in aging and cancer. Cancer Discov. 2016;6(6):584-593. doi: 10.1158/2159-8290.CD-16-0062
- Cabanos HF, Hata AN. Emerging insights into targeted therapy-tolerant persister cells in cancer. Cancers. 2021;13(11):2666. doi: 10.3390/cancers13112666
- Taylor S, Spugnini EP, Assaraf YG, Azzarito T, Rauch C, Fais S. Microenvironment acidity as a major determinant of tumor chemoresistance: proton pump inhibitors (PPIs) as a novel therapeutic approach. Drug Resist Updat. 2015;23:69-78. doi: 10.1016/j.drup.2015.08.004
- Icard P, Simula L, Fournel L, et al. The strategic roles of four enzymes in the interconnection between metabolism and oncogene activation in non-small cell lung cancer: therapeutic implications. Drug Resist Updat. 2022;63:100852. doi: 10.1016/j.drup.2022.100852
- Sun Y. Tumor microenvironment and cancer therapy resistance. Cancer Lett. 2015;380(1):205-215. doi: 10.1016/j.canlet.2015.07.044
- Seebacher NA, Krchniakova M, Stacy AE, Skoda J, Jansson PJ. Tumour microenvironment stress promotes the development of drug resistance. Antioxidants. 2021;10(11):1801. doi: 10.3390/antiox10111801
- Axpe E, Oyen ML. Applications of alginate-based bioinks in 3D bioprinting. Int J Mol Sci. 2016;17(12):1976. doi: 10.3390/ijms17121976
- Zhang J, Wehrle E, Vetsch JR, Paul GR, Rubert M, Müller R. Alginate dependent changes of physical properties in 3D bioprinted cell-laden porous scaffolds affect cell viability and cell morphology. Biomed Mater. 2019;14(6):065009. doi: 10.1088/1748-605X/ab3c74
- Zhang M, Zhao X. Alginate hydrogel dressings for advanced wound management. Int J Biol Macromol. 2020; 162:1414-1428. doi: 10.1016/j.ijbiomac.2020.07.311
- Thakur S, Govender PP, Mamo MA, Tamulevicius S, Thakur VK. Recent progress in gelatin hydrogel nanocomposites for water purification and beyond. Vacuum. 2017;146:396-408. doi: 10.1016/j.vacuum.2017.05.032
- Distler T, Solisito AA, Schneidereit D, Friedrich O, Detsch R, Boccaccini AR. 3D printed oxidized alginate-gelatin bioink provides guidance for C2C12 muscle precursor cell orientation and differentiation via shear stress during bioprinting. Biofabrication. 2020;12(4):045005. doi: 10.1088/1758-5090/ab98e4
- Wang X, Ao Q, Tian X, et al. Gelatin-based hydrogels for organ 3D bioprinting. Polymers. 2017;9(9):401. doi: 10.3390/polym9090401
- Bertlein S, Brown G, Lim KS, et al. Thiol-ene clickable gelatin: a platform bioink for multiple 3D biofabrication technologies. Adv Mater. 2017;29(44):201703404. doi: 10.1002/adma.201703404
- Leu Alexa R, Iovu H, Ghitman J, et al. 3D-printed gelatin methacryloyl-based scaffolds with potential application in tissue engineering. Polymers. 2021;13(5):727. doi: 10.3390/polym13050727
- Kumar H, Sakthivel K, Mohamed MGA, et al. Designing gelatin methacryloyl (GelMA)-based bioinks for visible light stereolithographic 3D biofabrication. Macromol Biosci. 2021;21(1):e2000317. doi: 10.1002/mabi.202000317
- Stepanovska J, Supova M, Hanzalek K, Broz A, Matejka R. Collagen bioinks for bioprinting: a systematic review of hydrogel properties, bioprinting parameters, protocols, and bioprinted structure characteristics. Biomedicines. 2021;9(9):1137. doi: 10.3390/biomedicines9091137
- Osidak EO, Karalkin PA, Osidak MS, et al. Viscoll collagen solution as a novel bioink for direct 3D bioprinting. J Mater Sci Mater Med. 2019;30(3):31. doi: 10.1007/s10856-019-6233-y
- Lee JM, Suen SKQ, Ng WL, Ma WC, Yeong WY. Bioprinting of collagen: considerations, potentials, and applications. Macromol Biosci. 2021;21(1):e2000280. doi: 10.1002/mabi.202000280
- Osidak EO, Kozhukhov VI, Osidak MS, Domogatsky SP. Collagen as bioink for bioprinting: a comprehensive review. Int J Bioprint. 2020;6(3):270. doi: 10.18063/ijb.v6i3.270
- Wang Z, Yang Y, Gao Y, Xu Z, Yang S, Jin M. Establishing a novel 3D printing bioinks system with recombinant human collagen. Int J Biol Macromol. 2022;211:400-409. doi: 10.1016/j.ijbiomac.2022.05.088
- Zhang W, Du A, Liu S, Lv M, Chen S. Research progress in decellularized extracellular matrix-derived hydrogels. Regen Ther. 2021;18:88-96. doi: 10.1016/j.reth.2021.04.002
- Wang H, Yu H, Zhou X, et al. An overview of extracellular matrix-based bioinks for 3D bioprinting. Front Bioeng Biotechnol. 2022;10:905438. doi: 10.3389/fbioe.2022.905438
- Liu C, Pei M, Li Q, Zhang Y. Decellularized extracellular matrix mediates tissue construction and regeneration. Front Med. 2022;16(1):56-82. doi: 10.1007/s11684-021-0900-3
- Rana D, Kumar TS, Ramalingam M. Cell-laden hydrogels for tissue engineering. J Biomater Tissue Eng. 2014;4. doi: 10.1166/jbt.2014.1206
- Liu H, Gong Y, Zhang K, et al. Recent advances in decellularized matrix-derived materials for bioink and 3D bioprinting. Gels. 2023;9(3):195. doi: 10.3390/gels9030195
- Abaci A, Guvendiren M. Designing decellularized extracellular matrix-based bioinks for 3D bioprinting. Adv Healthc Mater. 2020;9(24):e2000734. doi: 10.1002/adhm.202000734
- Colosi C, Shin SR, Manoharan V, et al. Microfluidic bioprinting of heterogeneous 3D tissue constructs using low-viscosity bioink. Adv Mater. 2016;28(4):677-684. doi: 10.1002/adma.201503310
- Soltan N, Ning L, Mohabatpour F, Papagerakis P, Chen X. Printability and cell viability in bioprinting alginate dialdehyde-gelatin scaffolds. ACS Biomater Sci Eng. 2019;5(6):2976-2987. doi: 10.1021/acsbiomaterials.9b00167
- Jeon O, Lee YB, Lee SJ, Guliyeva N, Lee J, Alsberg E. Stem cell-laden hydrogel bioink for generation of high resolution and fidelity engineered tissues with complex geometries. Bioact Mater. 2021;15:185-193. doi: 10.1016/j.bioactmat.2021.11.025
- Cui R, Li S, Li T, et al. Natural polymer derived hydrogel bioink with enhanced thixotropy improves printability and cellular preservation in 3D bioprinting. J Mater Chem B. 2023;11(17):3907-3918. doi: 10.1039/d2tb02786k
- Wang Q, Karadas Ö, Backman O, et al. Aqueous two-phase emulsion bioresin for facile one-step 3D microgel-based bioprinting. Adv Healthc Mater. 2023;12(19):e2203243. doi: 10.1002/adhm.202203243
- Zhang W, Kuss M, Yan Y, Shi W. Dynamic alginate hydrogel as an antioxidative bioink for bioprinting. Gels. 2023;9(4):312. doi: 10.3390/gels9040312
- Sachdev A 4th, Acharya S, Gadodia T, et al. A review on techniques and biomaterials used in 3D bioprinting. Cureus. 2022;14(8):e28463. doi: 10.7759/cureus.28463
- Zhu J, Li F, Wang X, Yu J, Wu D. Hyaluronic acid and polyethylene glycol hybrid hydrogel encapsulating nanogel with hemostasis and sustainable antibacterial property for wound healing. ACS Appl Mater Interfaces. 2018;10(16):13304-13316. doi: 10.1021/acsami.7b18927
- Shi L, Zhang J, Zhao M, et al. Effects of polyethylene glycol on the surface of nanoparticles for targeted drug delivery. Nanoscale. 2021;13(24):10748-10764. doi: 10.1039/d1nr02065j
- Wang J, Williamson GS, Yang H. Branched polyrotaxane hydrogels consisting of alpha-cyclodextrin and low-molecular-weight four-arm polyethylene glycol and the utility of their thixotropic property for controlled drug release. Colloids Surf B Biointerfaces. 2018;165:144-149. doi: 10.1016/j.colsurfb.2018.02.032
- Liu P, Chen W, Liu C, Tian M, Liu P. A novel poly (vinyl alcohol)/poly (ethylene glycol) scaffold for tissue engineering with a unique bimodal open-celled structure fabricated using supercritical fluid foaming. Sci Rep. 2019;9(1):9534. doi: 10.1038/s41598-019-46061-7
- Labet M, Thielemans W. Synthesis of polycaprolactone: a review. Chem Soc Rev. 2009;38(12):3484-504. doi: 10.1039/b820162p
- Liu G, Chen J, Wang X, Liu Y, Ma Y, Tu X. Functionalized 3D-printed ST2/gelatin methacryloyl/polcaprolactone scaffolds for enhancing bone regeneration with vascularization. Int J Mol Sci. 2022;23(15):8347. doi: 10.3390/ijms23158347
- Schmitt PR, Dwyer KD, Coulombe KLK. Current applications of polycaprolactone as a scaffold material for heart regeneration. ACS Appl Bio Mater. 2022;5(6):2461-2480. doi: 10.1021/acsabm.2c00174
- Khati V, Ramachandraiah H, Pati F, Svahn HA, Gaudenzi G, Russom A. 3D bioprinting of multi-material decellularized liver matrix hydrogel at physiological temperatures. Biosensors. 2022;12(7):521. doi: 10.3390/bios12070521
- Chen H, Fei F, Li X, et al. A structure-supporting, self-healing, and high permeating hydrogel bioink for establishment of diverse homogeneous tissue-like constructs. Bioact Mater. 2021;6(10):3580-3595. doi: 10.1016/j.bioactmat.2021.03.019
- Xu T, Jin J, Gregory C, Hickman JJ, Boland T. Inkjet printing of viable mammalian cells. Biomaterials. 2005;26(1):93-99. doi: 10.1016/j.biomaterials.2004.04.011
- Mandrycky CJ, Howard CC, Rayner SG, Shin YJ, Zheng Y. Organ-on-a-chip systems for vascular biology. J Mol Cell Cardiol. 2021;159:1-13. doi: 10.1016/j.yjmcc.2021.06.002
- Kolesky DB, Truby RL, Gladman AS, Busbee TA, Homan KA, Lewis JA. 3D bioprinting of vascularized, heterogeneous cell-laden tissue constructs. Adv Mater. 2014;26(19):3124-3130. doi: 10.1002/adma.201305506
- Cui X, Dean D, Ruggeri ZM, Boland T. Cell damage evaluation of thermal inkjet printed Chinese hamster ovary cells. Biotechnol Bioeng. 2010;106(6):963-969. doi: 10.1002/bit.22762
- Gudapati H, Dey M, Ozbolat I. A comprehensive review on droplet-based bioprinting: past, present and future. Biomaterials. 2016;102:20-42. doi: 10.1016/j.biomaterials.2016.06.012
- Angelopoulos I, Allenby MC, Lim M, Zamorano M. Engineering inkjet bioprinting processes toward translational therapies. Biotechnol Bioeng. 2020;117(1): 272-284. doi: 10.1002/bit.27176
- Stratesteffen H, Köpf M, Kreimendahl F, Blaeser A, Jockenhoevel S, Fischer H. GelMA-collagen blends enable drop-on-demand 3D printablility and promote angiogenesis. Biofabrication. 2017;9(4):045002. doi: 10.1088/1758-5090/aa857c
- Li W, Wang M, Ma H, Chapa-Villarreal FA, Lobo AO, Zhang YS. Stereolithography apparatus and digital light processing-based 3D bioprinting for tissue fabrication. iScience. 2023;26(2):106039. doi: 10.1016/j.isci.2023.106039
- Ozbolat IT, Hospodiuk M. Current advances and future perspectives in extrusion-based bioprinting. Biomaterials. 2016;76:321-343. doi: 10.1016/j.biomaterials.2015.10.076
- Naghieh S, Chen X. Printability-a key issue in extrusion-based bioprinting. J Pharm Anal. 2021;11(5):564-579. doi: 10.1016/j.jpha.2021.02.001
- Benwood C, Chrenek J, Kirsch RL, et al. Natural biomaterials and their use as bioinks for printing tissues. Bioengineering. 2021;8(2):27. doi: 10.3390/bioengineering8020027
- Mohan TS, Datta P, Nesaei S, Ozbolat V, Ozbolat IT. 3D coaxial bioprinting: process mechanisms, bioinks and applications. Prog Biomed Eng. 2022;4(2):022003. doi: 10.1088/2516-1091/ac631c
- Yu Y, Xie R, He Y, et al. Dual-core coaxial bioprinting of double-channel constructs with a potential for perfusion and interaction of cells. Biofabrication. 2022;14(3). doi: 10.1088/1758-5090/ac6e88
- Ning L, Mehta R, Cao C, et al. Embedded 3D bioprinting of gelatin methacryloyl-based constructs with highly tunable structural fidelity. ACS Appl Mater Interfaces. 2020;12(40):44563-44577. doi: 10.1021/acsami.0c15078
- Zhou K, Sun Y, Yang J, Mao H, Gu Z. Hydrogels for 3D embedded bioprinting: a focused review on bioinks and support baths. J Mater Chem B. 2022;10(12): 1897-1907. doi: 10.1039/d1tb02554f
- Zeng X, Meng Z, He J, et al. Embedded bioprinting for designer 3D tissue constructs with complex structural organization. Acta Biomater. 2022;140:1-22. doi: 10.1016/j.actbio.2021.11.048
- Li Q, Ma L, Gao Z, et al. Regulable supporting baths for embedded printing of soft biomaterials with variable stiffness. ACS Appl Mater Interfaces. 2022; 14(37):41695-41711. doi: 10.1021/acsami.2c09221
- Shao L, Gao Q, Xie C, et al. Directly coaxial 3D bioprinting of large-scale vascularized tissue constructs. Biofabrication. 2020;12(3):035014. doi: 10.1088/1758-5090/ab7e76
- Zheng W, Xie R, Liang X, Liang Q. Fabrication of biomaterials and biostructures based on microfluidic manipulation. Small. 2022;18(16):e2105867. doi: 10.1002/smll.202105867
- Fan XY, Deng ZF, Yan YY, et al. Application of microfluidic chips in anticancer drug screening. Bosn J Basic Med Sci. 2022;22(3):302-314. doi: 10.17305/bjbms.2021.6484
- Torino S, Corrado B, Iodice M, Coppola G. PDMS-based microfluidic devices for cell culture. Inventions. 2018;3:65. doi: 10.3390/inventions3030065
- Yu F, Choudhury D. Microfluidic bioprinting for organ-on-a-chip models. Drug Discov Today. 2019;24(6):1248-1257. doi: 10.1016/j.drudis.2019.03.025
- Yi HG, Jeong YH, Kim Y, et al. A bioprinted human-glioblastoma-on-a-chip for the identification of patient-specific responses to chemoradiotherapy. Nat Biomed Eng. 2019;3(7):509-519. doi: 10.1038/s41551-019-0363-x
- Xu F, Wu J, Wang S, Durmus NG, Gurkan UA, Demirci U. Microengineering methods for cell-based microarrays and high-throughput drug-screening applications. Biofabrication. 2011;3(3):034101. doi: 10.1088/1758-5082/3/3/034101
- Liang Y, Pan J, Fang Q. Research advances of high-throughput cell-based drug screening systems based on microfluidic technique. Se Pu. 2021;39(6):567-577. doi: 10.3724/SP.J.1123.2020.07014
- Wu Q, Liu J, Wang X, et al. Organ-on-a-chip: recent breakthroughs and future prospects. Biomed Eng Online. 2020;19(1):9. doi: 10.1186/s12938-020-0752-0
- Knowlton S, Tasoglu S. A bioprinted liver-on-a-chip for drug screening applications.Trends Biotechnol. 2016; 34(9):681-682. doi: 10.1016/j.tibtech.2016.05.014
- Hoofnagle JH, Björnsson ES. Drug-induced liver injury - types and phenotypes. N Engl J Med. 2019;381(3):264-273. doi: 10.1056/NEJMra1816149
- Yin L, Du G, Zhang B, et al. Efficient drug screening and nephrotoxicity assessment on co-culture microfluidic kidney chip. Sci Rep. 2020;10(1):6568. doi: 10.1038/s41598-020-63096-3
- Lee H, Cho DW. One-step fabrication of an organ-on-a-chip with spatial heterogeneity using a 3D bioprinting technology. Lab Chip. 2016;16(14):2618-2625. doi: 10.1039/c6lc00450d
- Lee H, Chae S, Kim JY, et al. Cell-printed 3D liver-on-a-chip possessing a liver microenvironment and biliary system. Biofabrication. 2019;11(2):025001. doi: 10.1088/1758-5090/aaf9fa
- Zhang YS, Arneri A, Bersini S, et al. Bioprinting 3D microfibrous scaffolds for engineering endothelialized myocardium and heart-on-a-chip. Biomaterials. 2016;110:45-59. doi: 10.1016/j.biomaterials.2016.09.003
- Lin NYC, Homan KA, Robinson SS, et al. Renal reabsorption in 3D vascularized proximal tubule models. Proc Natl Acad Sci U S A. 2019;116(12):5399-5404. doi: 10.1073/pnas.1815208116
- Park JY, Ryu H, Lee B, et al. Development of a functional airway-on-a-chip by 3D cell printing. Biofabrication. 2018;11(1):015002. doi: 10.1088/1758-5090/aae545
- Marino A, Tricinci O, Battaglini M, et al. A 3D real-scale, biomimetic, and biohybrid model of the blood-brain barrier fabricated through two-photon lithography. Small. 2018;14(6). doi: 10.1002/smll.201702959
- Mandt D, Gruber P, Markovic M, et al. Fabrication of biomimetic placental barrier structures within a microfluidic device utilizing two-photon polymerization. Int J Bioprint. 2018;4(2):144. doi: 10.18063/IJB.v4i2.144
- Kim W, Lee Y, Kang D, Kwak T, Lee H-R, Jung S. 3D inkjet-bioprinted lung-on-a-chip. ACS Biomater Sci Eng. 2023;9(5):2806-2815. doi: 10.1021/acsbiomaterials.3c00089
- Li W, Zhou Z, Zhou X, et al. 3D biomimetic models to reconstitute tumor microenvironment in vitro: spheroids, organoids, and tumor-on-a-chip. Adv Healthc Mater. 2023;e2202609. doi: 10.1002/adhm.202202609
- Lee H, Kim J, Choi Y, Cho D-W. Application of gelatin bioinks and cell-printing technology to enhance cell delivery capability for 3D liver fibrosis-on-a-chip development. ACS Biomater Sci Eng. 2020;6(4):2469-2477. doi: 10.1021/acsbiomaterials.9b01735
- Nothdurfter D, Ploner C, Coraça-Huber DC, et al. 3D bioprinted, vascularized neuroblastoma tumor environment in fluidic chip devices for precision medicine drug testing. Biofabrication. 2022;14(3). doi: 10.1088/1758-5090/ac5fb7
- Zhang YS, Davoudi F, Walch P, et al. Bioprinted thrombosis-on-a-chip. Lab Chip. 2016;16(21):4097-4105. doi: 10.1039/c6lc00380j
- Huang CBX, Tu TY. Recent advances in vascularized tumor-on-a-chip. Front Oncol. 2023;13:1150332. doi: 10.3389/fonc.2023.1150332
- Mi S, Yang S, Liu T, et al. A novel controllable cell array printing technique on microfluidic chips. IEEE Trans Biomed Eng. 2019;66(9):2512-2520. doi: 10.1109/TBME.2019.2891016
- Jameson JL, Longo DL. Precision medicine-- personalized, problematic, and promising. N Engl J Med. 2015;372(23):2229-2234. doi: 10.1056/NEJMsb1503104
- van Riet S, van Schadewijk A, Khedoe PPSJ, et al. Organoid-based expansion of patient-derived primary alveolar type 2 cells for establishment of alveolus epithelial lung-chip cultures. Am J Physiol Lung Cell Mol Physiol. 2022;322(4):L526-L538. doi: 10.1152/ajplung.00153.2021
- Haque MR, Wessel CR, Leary DD, Wang C, Bhushan A, Bishehsari F. Patient-derived pancreatic cancer-on-a-chip recapitulates the tumor microenvironment. Microsyst Nanoeng. 2022;8:36. doi: 10.1038/s41378-022-00370-6
- Hu Y, Sui X, Song F, et al. Lung cancer organoids analyzed on microwell arrays predict drug responses of patients within a week. Nat Commun. 2021;12(1):2581. doi: 10.1038/s41467-021-22676-1
- Fan H, Demirci U, Chen P. Emerging organoid models: leaping forward in cancer research. J Hematol Oncol. 2019;12(1):142. doi: 10.1186/s13045-019-0832-4
- Lee SH, Sung JH. Organ-on-a-chip technology for reproducing multiorgan physiology. Adv Healthc Mater. 2018;7(2). doi: 10.1002/adhm.201700419
- Shinha K, Nihei W, Ono T, Nakazato R, Kimura H. A pharmacokinetic-pharmacodynamic model based on multi-organ-on-a-chip for drug-drug interaction studies. Biomicrofluidics. 2020;14(4):044108. doi: 10.1063/5.0011545
- Ronaldson-Bouchard K, Teles D, Yeager K, et al. A multi-organ chip with matured tissue niches linked by vascular flow. Nat Biomed Eng. 2022;6(4):351-371. doi: 10.1038/s41551-022-00882-6
- Li ZA, Tuan RS. Towards establishing human body-on-a-chip systems. Stem Cell Res Ther. 2022;13(1):431. doi: 10.1186/s13287-022-03130-5
- Ingber DE. Human organs-on-chips for disease modelling, drug development and personalized medicine. Nat Rev Genet. 2022;23(8):467-491. doi: 10.1038/s41576-022-00466-9
- van Hasselt JGC, Iyengar R. Systems pharmacology: defining the interactions of drug combinations. Annu Rev Pharmacol Toxicol. 2019;59:21-40. doi: 10.1146/annurev-pharmtox-010818-021511
- Wan L, Yin J, Skoko J, et al. 3D collagen vascular tumor-on-a-chip mimetics for dynamic combinatorial drug screening. Mol Cancer Ther. 2021;20(6): 1210-1219. doi: 10.1158/1535-7163.MCT-20-0880