Roles of pore architecture of artificial bone grafts in invasion competition between bone and fibrous tissue and orientation of regenerated bone
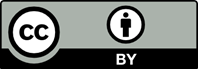
In the reconstruction of bone defects close to soft tissue, preventing the invasion of fibrous tissue into the bone defect is key to successful bone reconstruction. In this study, we clarified the effects of the pore architecture of artificial bone grafts on the penetration of bone and fibrous tissue, and the orientation of regenerated bone. Carbonate apatite grafts with uniaxial pores along the long- (L-graft) or short-axis (S-graft) direction of the graft and biaxial pores along the long- and short-axes (LS-graft) were used. These grafts were implanted in bone defects created by rabbit ulnae amputation. The pores of the L-, S-, and LS-grafts opened into the bone stumps, muscles, and bone stumps and muscles together, respectively. In the L-graft, the graft pores developed bone from the bone stump to the graft center, while preventing excessive invasion of fibrous tissue. In S- and LS-grafts, the graft pores along the short axis allowed the invasion of fibrous tissue into the grafts. Consequently, although the bone grew to the edge regions in these grafts, further bone ingrowth was inhibited by the fibrous tissue. Furthermore, the pore architecture of the grafts affected the orientation of the regenerated bone. The degree of orientation of the bone formed in the L- and S-grafts was 1.6-fold higher than that formed in the LS-grafts. Thus, controlling the pore architecture allowed the growth of bone to predominate over that of fibrous tissue and induced the formation of bone with an ordered orientation
- GBD 2019 Fracture Collaborators. Global, regional, and national burden of bone fractures in 204 countries and territories, 1990–2019: a systematic analysis from the Global Burden of Disease Study 2019. Lancet Healthy Longev. 2021;2:e580-e592. doi: 10.1016/S2666-7568(21)00172-0
- Shen Y, Huang X, Wu J, et al. The global burden of osteoporosis, low bone mass, and its related fracture in 204 countries and territories, 1990-2019. Front Endocrinol. 2022;13. doi: 10.3389/fendo.2022.882241
- Stewart SK. Fracture non-union: a review of clinical challenges and future research needs. Malays Orthop J. 2019;13:1-10. doi: 10.5704/MOJ.1907.001
- Wildemann B, Ignatius A, Leung F, et al. Non-union bone fractures. Nat Rev Dis Primers. 2021;7:57. doi: 10.1038/s41572-021-00289-8
- Leng Y, Yang F, Wang Q, et al. Material-based therapy for bone nonunion. Mater Des. 2019;183:108161. doi: 10.1016/j.matdes.2019.108161
- Dahl MT, Morrison S. Segmental bone defects and the history of bone transport. J Orthop Trauma. 2021;35:S1-7. doi: 10.1097/BOT.0000000000002124
- Sparks DS, Saifzadeh S, Savi FM, et al. A preclinical large-animal model for the assessment of critical-size load-bearing bone defect reconstruction. Nat Protoc. 2020;15:877-924. doi: 10.1038/s41596-019-0271-2
- Schenk RK, Buser D, Hardwick WR, Dahlin C. Healing pattern of bone regeneration in membrane-protected defects: a histologic study in the canine mandible. Int J Oral Maxillofac. 1994;9:13-29. https://www.researchgate.net/publication/15047765
- Reichert JC, Wullschleger ME, Cipitria A, et al. Custom-made composite scaffolds for segmental defect repair in long bones. Int Ortho. 2011;35:1229-1236. doi: 10.1007/s00264-010-1146-x
- Cipitria A, Lange C, Schell H, et al. Porous scaffold architecture guides tissue formation. J Bone Miner Res. 2012;27:1275-1288. doi: 10.1002/jbmr.1589
- Berner A, Reichert JC, Woodruff MA, et al. Autologous vs. allogenic mesenchymal progenitor cells for the reconstruction of critical sized segmental tibial bone defects in aged sheep. Acta Biomater. 2013;9:7874-7884. doi: 10.1016/j.actbio.2013.04.035
- Akagi H, Ochi H, Soeta S, et al. A comparison of the process of remodeling of hydroxyapatite/Poly-D/L-lactide and beta-tricalcium phosphate in a loading site. Biomed Res Int. 2015;2015:730105. doi: 10.1155/2015/730105
- Song J, Kim J, Woo HM, et al. Repair of rabbit radial bone defects using bone morphogenetic protein-2 combined with 3D porous silk fibroin/β-tricalcium phosphate hybrid scaffolds. J Biomater Sci Polym Ed. 2018;29:716-729. doi: 10.1080/09205063.2018.1438126
- Abtahi S, Chen X, Shahabi S, Nasiri N. Resorbable membranes for guided bone regeneration: critical features, potentials, and limitations. ACS Mater Au. 2023;3:394-417. doi: 10.1021/acsmaterialsau.3c00013
- Lee FH, Shen PC, Jou IM, Li C-Y, Hsieh J-L. A population-based 16-year study on the risk factors of surgical site infection in patients after bone grafting. Medicine. 2015;94:e2034. doi: 10.1097/MD.0000000000002034
- Rodham PL, Giannoudis VP, Kanakaris NK, Giannoudis PV. Biological aspects to enhance fracture healing. EFORT Open Rev. 2023;8:264-282. doi: 10.1530/EOR-23-0047
- Barba A, Diez-Escudero A, Maazouz Y, et al. Osteoinduction by foamed and 3D-printed calcium phosphate scaffolds: effect of nanostructure and pore architecture. ACS Appl Mater Interfaces. 2017;9:41722-41736. doi: 10.1021/acsami.7b14175
- Nakamura T, Matsumine A, Asanuma K, Matsubara T, Sudo A. Treatment of bone defect with calcium phosphate cement subsequent to tumor curettage in pediatric patients. Oncol Lett. 2016;11:247-252. doi: 10.3892/ol.2015.3855
- Hayashi K, Kishida R, Tsuchiya A, Ishikawa K. Honeycomb blocks composed of carbonate apatite, β-tricalcium phosphate, and hydroxyapatite for bone regeneration: effects of composition on biological responses. Mater Today Bio. 2019;4:100031. doi: 10.1016/j.mtbio.2019.100031
- Hayashi K, Kishida R, Tsuchiya A, Ishikawa K. Granular honeycombs composed of carbonate apatite, hydroxyapatite, and β-tricalcium phosphate as bone graft substitutes: effects of composition on bone formation and maturation. ACS Appl Bio Mater. 2020;3:1787-1795. doi: 10.1021/acsabm.0c00060
- Hayashi K, Shimabukuro M, Kishida R, Tsuchiya A, Ishikawa K. Honeycomb scaffolds capable of achieving barrier membrane-free guided bone regeneration. Mater Adv. 2021;2:7638-7649. doi: 10.1039/d1ma00698c
- Hayashi K, Shimabukuro M, Kishida R, Tsuchiya A, Ishikawa K. Structurally optimized honeycomb scaffolds with outstanding ability for vertical bone augmentation. J Adv Res. 2022;41:101-112. doi: 10.1016/j.jare.2021.12.010
- Hayashi K, Kishida R, Tsuchiya A, Ishikawa K. Channel aperture characteristics of carbonate apatite honeycomb scaffolds affect ingrowths of bone and fibrous tissues in vertical bone augmentation. Bioengineering. 2022;9(11):627. doi: 10.3390/bioengineering9110627
- Feng YF, Wang L, Li X, et al. Influence of architecture of β-tricalcium phosphate scaffolds on biological performance in repairing segmental bone defects. PLoS One. 2012;7:e49955. doi: 10.1371/journal.pone.0049955
- Petersen A, Princ A, Korus G, et al. A biomaterial with a channel-like pore architecture induces endochondral healing of bone defects. Nat Commun. 2018;9:4430. doi: 10.1038/s41467-018-06504-7
- Hayashi K, Kishida R, Tsuchiya A, Ishikawa K. Supporting information superiority of triply periodic minimal surface gyroid structure to strut-based grid structure in both strength and bone regeneration. ACS Appl Mater Interfaces. 2023;15:34570-34577. doi: 10.1021/acsami.3c06263
- Shibahara K, Hayashi K, Nakashima Y, Ishikawa K. Honeycomb scaffold-guided bone reconstruction of critical-sized defects in rabbit ulnar shafts. ACS Appl Bio Mater. 2021;4:6821-6831. doi: 10.1021/acsabm.1c00533
- Julien A, Kanagalingam A, Martínez-Sarrà E, et al. Direct contribution of skeletal muscle mesenchymal progenitors to bone repair. Nat Commun. 2021;12:2860. doi: 10.1038/s41467-021-22842-5
- Hayashi K, Yanagisawa T, Kishida R, Ishikawa K. Effects of scaffold shape on bone regeneration: tiny shape differences affect the entire system. ACS Nano. 2022;16:11755-11768. doi: 10.1021/acsnano.2c03776
- Boudaoud A, Burian A, Borowska-Wykrȩt D, et al. FibrilTool, an ImageJ plug-in to quantify fibrillar structures in raw microscopy images. Nat Protoc. 2014;9:457-463. doi: 10.1038/nprot.2014.024
- Gurcan MN, Boucheron LE, Can A, Madabhushi A, Rajpoot NM, Yener B. Histopathological image analysis: a review. IEEE Rev Biomed Eng. 2009;2:147-171. https://doi.org /10.1109/RBME.2009.2034865
- Kanda Y. Investigation of the freely available easy-to-use software “EZR” for medical statistics. Bone Marrow Transplant. 2013;48:452-458. doi: 10.1038/bmt.2012.244
- Ren F, Ding Y, Leng Y. Infrared spectroscopic characterization of carbonated apatite: a combined experimental and computational study. J Biomed Mater Res A. 2014; 102:496-505. doi: 10.1002/jbm.a.34720
- Madupalli H, Pavan B, Tecklenburg MMJ. Carbonate substitution in the mineral component of bone: discriminating the structural changes, simultaneously imposed by carbonate in A and B sites of apatite. J Solid State Chem. 2017;255:27-35. doi: 10.1016/j.jssc.2017.07.025
- Jodati H, Yılmaz B, Evis Z. A review of bioceramic porous scaffolds for hard tissue applications: effects of structural features. Ceram Int. 2020;46:15725-15739. doi: 10.1016/j.ceramint.2020.03.192
- Hara K, Hellem E, Yamada S, et al. Efficacy of treating segmental bone defects through endochondral ossification: 3D printed designs and bone metabolic activities. Mater Today Bio. 2022;14:100237. doi: 10.1016/j.mtbio.2022.100237
- Pan Q, Li Y, Xu J, et al. The effects of tubular structure on biomaterial aided bone regeneration in distraction osteogenesis. J Orthop Transl. 2020;25:80-86. doi: 10.1016/j.jot.2020.09.009
- Al-Maharma AY, Patil SP, Markert B. Effects of porosity on the mechanical properties of additively manufactured components: a critical review. Mater Res Express. 2020;7:122001. doi: 10.1088/2053-1591/abcc5d
- Hannink G, Arts JJC. Bioresorbability, porosity and mechanical strength of bone substitutes: what is optimal for bone regeneration? Injury. 2011;42:S22-S25. doi: 10.1016/j.injury.2011.06.008
- Shibahara K, Hayashi K, Nakashima Y, Ishikawa K. Reconstruction of load-bearing segmental bone defects using carbonate apatite honeycomb blocks. ACS Mater Au. 2023;3:321-336. doi: 10.1021/acsmaterialsau.3c00008
- Lu T, Feng S, He F, Ye J. Enhanced osteogenesis of honeycomb β-tricalcium phosphate scaffold by construction of interconnected pore structure: an in vivo study. J Biomed Mater Res A. 2020;108:645-653. doi: 10.1002/jbm.a.36844
- He F, Lu T, Fang X, et al. Novel extrusion-microdrilling approach to fabricate calcium phosphate-based bioceramic scaffolds enabling fast bone regeneration. ACS Appl Mater Interfaces. 2020;12:32340-32351. doi: 10.1021/acsami.0c07304
- Zhao YN, Fan JJ, Li ZQ, Liu YW, Wu YP, Liu J. Effects of pore size on the osteoconductivity and mechanical properties of calcium phosphate cement in a rabbit model. Artif Organs. 2017;41:199-204. doi: 10.1111/aor.12742
- Van Bael S, Chai YC, Truscello S, et al. The effect of pore geometry on the in vitro biological behavior of human periosteum-derived cells seeded on selective laser-melted Ti6Al4V bone scaffolds. Acta Biomater. 2012;8:2824-2834. doi: 10.1016/j.actbio.2012.04.001
- Hoerth RM, Seidt BM, Shah M, et al. Mechanical and structural properties of bone in non-critical and critical healing in rat. Acta Biomater. 2014;10:4009-4019. doi: 10.1016/j.actbio.2014.06.003