Modeling inflammatory response using 3D bioprinting of polarized macrophages
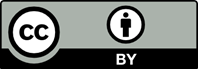
The field of drug discovery has seen the rise of three-dimensional (3D) bioprinting as a promising tool for disease modeling. The focus to date has been on tissue engineering and cancer modeling, although the application of 3D-bioprinted models for the study of inflammation using macrophages is still in its infancy. This study explores the potential of bioprinting technology in the development of a 3D macrophage model and macrophage response to inflammatory stimuli using this platform. To this end, we established a 3D-bioprinted macrophage model and assessed the inflammatory and anti-inflammatory response to bacterial endotoxin (lipopolysaccharide, LPS) and the drug ibuprofen (Ibu), respectively. Optimal conditions for macrophage differentiation of the human monocytic cell line, THP-1, in the 3D environment were studied, as well as the effect of the 3D microenvironment on macrophage polarization. Viability of THP-1 cells following the 3D bioprinting process was demonstrated and maintained, allowing successful macrophage differentiation of the cells. The developed 3D-bioprinted macrophage model exhibited elevated expression of selected pro-inflammatory gene and protein markers following exposure to LPS, consistent with polarization from M0 to M1 phenotype. Additionally, the model was responsive to the anti-inflammatory properties of Ibu, demonstrating its potential in drug screening and discovery. The current study highlights the potential of bioprinting in the investigation of inflammatory cell response and behavior in a 3D environment ex vivo, opening new avenues for research in modeling inflammatory responses to various stimuli.
- Merien F. A journey with Elie Metchnikoff: from innate cell mechanisms in infectious diseases to quantum biology. Front Public Health. 2016;4:125. doi: 10.3389/fpubh.2016.00125
- Lokaj J, John C. Ilya Ilich Metchnikov and Paul Ehrlich: 1908 Nobel Prize winners for their research on immunity. Epidemiol Mikrobiol Imunol. 2008;57(4):119-124.
- Wrzesinski K, Fey SJ. From 2D to 3D--a new dimension for modelling the effect of natural products on human tissue. Curr Pharm Des. 2015;21(38):5605-5616. doi: 10.2174/1381612821666151002114227
- Kapalczynska M, Kolenda T, Przybyla W, et al. 2D and 3D cell cultures - a comparison of different types of cancer cell cultures. Arch Med Sci. 2018;14(4):910-919. doi: 10.5114/aoms.2016.63743
- Jensen C, Teng Y. Is it time to start transitioning from 2D to 3D cell culture? Front Mol Biosci. 2020;7:33. doi: 10.3389/fmolb.2020.00033
- Malindisa S, Josephs JS, Ntwasa M. Two-dimensional (2D) and three-dimensional (3D) cell culturing in drug discovery. In: Radwa Ali M, ed. Cell Culture. IntechOpen; 2018.
- Gunti S, Hoke ATK, Vu KP, London NR, Jr. Organoid and spheroid tumor models: techniques and applications. Cancers (Basel). 2021;13(4). doi: 10.3390/cancers13040874
- Dini S, Binder BJ, Fischer SC, et al. Identifying the necrotic zone boundary in tumour spheroids with pair-correlation functions. J R Soc Interface. 2016;13(123). doi: 10.1098/rsif.2016.0649
- Germain N, Dhayer M, Dekiouk S, Marchetti P. Current advances in 3D bioprinting for cancer modeling and personalized medicine. Int J Mol Sci. 2022;23(7). doi: 10.3390/ijms23073432
- Agarwal S, Saha S, Balla VK, Pal A, Barui A, Bodhak S. Current developments in 3D bioprinting for tissue and organ regeneration–a review. Front Mech Eng. 2020;6. doi: 10.3389/fmech.2020.589171
- Bose S, Roy M, Bandyopadhyay A. Recent advances in bone tissue engineering scaffolds. Trends Biotechnol. 2012;30(10):546-554. doi: 10.1016/j.tibtech.2012.07.005
- Satpathy A, Datta P, Wu Y, Ayan B, Bayram E, Ozbolat IT. Developments with 3D bioprinting for novel drug discovery. Expert Opin Drug Discov. 2018;13(12):1115-1129. doi: 10.1080/17460441.2018.1542427
- Sun T, Jackson S, Haycock JW, MacNeil S. Culture of skin cells in 3D rather than 2D improves their ability to survive exposure to cytotoxic agents. J Biotechnol. 2006;122(3):372-381. doi: 10.1016/j.jbiotec.2005.12.021
- Li Y, Kilian KA. Bridging the gap: from 2D cell culture to 3D microengineered extracellular matrices. Adv Healthc Mater. 2015;4(18):2780-2796. doi: 10.1002/adhm.201500427
- Langer R, Vacanti J. Advances in tissue engineering. J Pediatr Surg. 2016;51(1):8-12. doi: 10.1016/j.jpedsurg.2015.10.022
- Knight E, Przyborski S. Advances in 3D cell culture technologies enabling tissue-like structures to be created in vitro. J Anat. 2015;227(6):746-756. doi: 10.1111/joa.12257
- Potyondy T, Uquillas JA, Tebon PJ, et al. Recent advances in 3D bioprinting of musculoskeletal tissues. Biofabrication. 2021;13(2). doi: 10.1088/1758-5090/abc8de
- Barreiro Carpio M, Dabaghi M, Ungureanu J, Kolb MR, Hirota JA, Moran-Mirabal JM. 3D bioprinting strategies, challenges, and opportunities to model the lung tissue microenvironment and its function. Front Bioeng Biotechnol. 2021;9:773511. doi: 10.3389/fbioe.2021.773511
- Zhong Z, Wang J, Tian J, et al. Rapid 3D bioprinting of a multicellular model recapitulating pterygium microenvironment. Biomaterials. 2022;282:121391. doi: 10.1016/j.biomaterials.2022.121391
- Sun X, Ma Z, Zhao X, et al. Three-dimensional bioprinting of multicell-laden scaffolds containing bone morphogenic protein-4 for promoting M2 macrophage polarization and accelerating bone defect repair in diabetes mellitus. Bioact Mater. 2021;6(3):757-769. doi: 10.1016/j.bioactmat.2020.08.030
- Berg J, Weber Z, Fechler-Bitteti M, et al. Bioprinted multi-cell type lung model for the study of viral inhibitors. Viruses. 2021;13(8). doi: 10.3390/v13081590
- Ouyang L, Yao R, Zhao Y, Sun W. Effect of bioink properties on printability and cell viability for 3D bioplotting of embryonic stem cells. Biofabrication. 2016;8(3):035020. doi: 10.1088/1758-5090/8/3/035020
- Thattaruparambil Raveendran N, Vaquette C, Meinert C, Samuel Ipe D, Ivanovski S. Optimization of 3D bioprinting of periodontal ligament cells. Dent Mater. 2019;35(12):1683-1694. doi: 10.1016/j.dental.2019.08.114
- Haloul M, Oliveira ERA, Kader M, et al. mTORC1-mediated polarization of M1 macrophages and their accumulation in the liver correlate with immunopathology in fatal ehrlichiosis. Sci Rep. 2019;9(1):14050. doi: 10.1038/s41598-019-50320-y
- Starr T, Bauler TJ, Malik-Kale P, Steele-Mortimer O. The phorbol 12-myristate-13-acetate differentiation protocol is critical to the interaction of THP-1 macrophages with Salmonella Typhimurium. PLOS ONE. 2018;13(3): e0193601. doi: 10.1371/journal.pone.0193601
- Surdziel E, Clay I, Nigsch F, et al. Multidimensional pooled shRNA screens in human THP-1 cells identify candidate modulators of macrophage polarization. PLOS ONE. 2017;12(8):e0183679. doi: 10.1371/journal.pone.0183679
- Schmittgen TD, Livak KJ. Analyzing real-time PCR data by the comparative CT method. Nat Protoc. 2008;3(6):1101-1108. doi: 10.1038/nprot.2008.73
- Kale P. Pharmacokinetics and bioavailability of single dose ibuprofen and pseudoephedrine alone or in combination: a randomized three-period, cross-over trial in healthy Indian volunteers. Front Pharmacol. 2014;5:98. doi: 10.3389/fphar.2014.00098
- Dey M, Ozbolat IT. 3D bioprinting of cells, tissues and organs. Sci Rep. 2020;10(1):14023. doi: 10.1038/s41598-020-70086-y
- Almela T, Tayebi L, Moharamzadeh K. 3D bioprinting for in vitro models of oral cancer: toward development and validation. Bioprinting. 2021;22:e00132. doi: 10.1016/j.bprint.2021.e00132
- Neufeld L, Yeini E, Pozzi S, Satchi-Fainaro R. 3D bioprinted cancer models: from basic biology to drug development. Nat Rev Cancer. 2022;22(12):679-692. doi: 10.1038/s41568-022-00514-w
- Aldrich A, Kuss MA, Duan B, Kielian T. 3D bioprinted scaffolds containing viable macrophages and antibiotics promote clearance of Staphylococcus aureus craniotomy-associated biofilm infection. ACS Appl Mater Interfaces. 2019;11(13):12298-12307. doi: 10.1021/acsami.9b00264
- Raveendran N, Ivanovski S, Vaquette C. The effect of culture conditions on the bone regeneration potential of osteoblast-laden 3D bioprinted constructs. Acta Biomater. 2023;156:190-201. doi: 10.1016/j.actbio.2022.09.042
- Auwerx J, Staels B, Van Vaeck F, Ceuppens JL. Changes in IgG Fc receptor expression induced by phorbol 12-myristate 13-acetate treatment of THP-1 monocytic leukemia cells. Leuk Res. 1992;16(3):317-327. doi: 10.1016/0145-2126(92)90070-n
- Schwende H, Fitzke E, Ambs P, Dieter P. Differences in the state of differentiation of THP-1 cells induced by phorbol ester and 1,25-dihydroxyvitamin D3. J Leukoc Biol. 1996;59(4):555-561.
- Qin Z. The use of THP-1 cells as a model for mimicking the function and regulation of monocytes and macrophages in the vasculature. Atherosclerosis. 2012;221(1):2-11. doi: 10.1016/j.atherosclerosis.2011.09.003
- Aldo PB, Craveiro V, Guller S, Mor G. Effect of culture conditions on the phenotype of THP-1 monocyte cell line. Am J Reprod Immunol. 2013;70(1):80-86. doi: 10.1111/aji.12129
- Park EK, Jung HS, Yang HI, Yoo MC, Kim C, Kim KS. Optimized THP-1 differentiation is required for the detection of responses to weak stimuli. Inflamm Res. 2007;56(1):45-50. doi: 10.1007/s00011-007-6115-5
- Maeß MB, Wittig B, Cignarella A, Lorkowski S. Reduced PMA enhances the responsiveness of transfected THP-1 macrophages to polarizing stimuli. J Immunol Methods. 2014;402(1-2):76-81. doi: 10.1016/j.jim.2013.11.006
- Wang L, Zhu L, Duan C, Li L, Chen G. Total saponin of Dioscorea collettii attenuates MSU crystal‑induced inflammation via inhibiting the activation of the NALP3 inflammasome and caspase‑1 in THP‑1 macrophages. Mol Med Rep. 2020;21(6):2466-2474 doi: 10.3892/mmr.2020.11035
- Sapudom J, Karaman S, Mohamed WKE, Garcia-Sabaté A, Quartey BC, Teo JCM. 3D in vitro M2 macrophage model to mimic modulation of tissue repair. NPJ Regen Med. 2021;6(1):83. doi: 10.1038/s41536-021-00193-5
- Garcia-Sabaté A, Mohamed WKE, Sapudom J, Alatoom A, Al Safadi L, Teo JCM. Biomimetic 3D models for investigating the role of monocytes and macrophages in atherosclerosis. Bioengineering. 2020;7(3). doi: 10.3390/bioengineering7030113
- Sapudom J, Mohamed WKE, Garcia-Sabaté A, et al. Collagen fibril density modulates macrophage activation and cellular functions during tissue repair. Bioengineering (Basel). 2020;7(2). doi: 10.3390/bioengineering7020033
- Bystroňová J, Ščigalková I, Wolfová L, Pravda M, Vrana NE, Velebný V. Creating a 3D microenvironment for monocyte cultivation: ECM-mimicking hydrogels based on gelatine and hyaluronic acid derivatives. RSC Adv. 2018;8(14):7606-7614. doi: 10.1039/c7ra13739g
- Barthes J, Dollinger C, Muller CB, et al. Immune assisted tissue engineering via incorporation of macrophages in cell-laden hydrogels under cytokine stimulation. Front Bioeng Biotechnol. 2018;6. doi: 10.3389/fbioe.2018.00108
- Wodzanowski KA, Caplan JL, Kloxin AM, Grimes CL. Multiscale invasion assay for probing macrophage response to gram-negative bacteria. Front Chem. 2022;10. doi: 10.3389/fchem.2022.842602
- Mantovani A, Sica A, Sozzani S, Allavena P, Vecchi A, Locati M. The chemokine system in diverse forms of macrophage activation and polarization. Trends Immunol. 2004;25(12):677-686. doi: 10.1016/j.it.2004.09.015
- Rostam HM, Singh S, Vrana NE, Alexander MR, Ghaemmaghami AM. Impact of surface chemistry and topography on the function of antigen presenting cells. Biomater Sci. 2015;3(3):424-441. doi: 10.1039/c4bm00375f
- Wong VW, Rustad KC, Akaishi S, et al. Focal adhesion kinase links mechanical force to skin fibrosis via inflammatory signaling. Nat Med. 2011;18(1):148-152. doi: 10.1038/nm.2574
- Madden LR, Mortisen DJ, Sussman EM, et al. Proangiogenic scaffolds as functional templates for cardiac tissue engineering. Proc Natl Acad Sci USA. 2010;107(34):15211-15216. doi: 10.1073/pnas.1006442107
- Zhou J, Bai W, Liu Q, Cui J, Zhang W. Intermittent hypoxia enhances THP-1 monocyte adhesion and chemotaxis and promotes M1 macrophage polarization via RAGE. Biomed Res Int. 2018;2018:1650456. doi: 10.1155/2018/1650456
- Sicari BM, Dziki JL, Siu BF, Medberry CJ, Dearth CL, Badylak SF. The promotion of a constructive macrophage phenotype by solubilized extracellular matrix. Biomaterials. 2014;35(30):8605-8612. doi: 10.1016/j.biomaterials.2014.06.060
- Shackelford RE, Alford PB, Xue Y, Thai S-F, Adams DO, Pizzo S. Aspirin inhibits tumor necrosis factorα gene expression in murine tissue macrophages. Mol Pharmacol. 1997;52(3):421-429. doi: 10.1124/mol.52.3.421
- Lee YJ, Chuang YC. Ibuprofen augments pro-inflammatory cytokine release in a mouse model of Vibrio vulnificus infection. Microbiol Immunol. 2010;54(9):542-550. doi: 10.1111/j.1348-0421.2010.00249.x
- Uematsu S, Matsumoto M, Takeda K, Akira S. Lipopolysaccharide-dependent prostaglandin E(2) production is regulated by the glutathione-dependent prostaglandin E(2) synthase gene induced by the Toll-like receptor 4/MyD88/NF-IL6 pathway. J Immunol. 2002;168(11):5811-5816. doi: 10.4049/jimmunol.168.11.5811
- Rafiei M, Kiani F, Sayehmiri F, Sayehmiri K, Sheikhi A, Zamanian Azodi M. Study of Porphyromonas gingivalis in periodontal diseases: a systematic review and meta-analysis. Med J Islam Repub Iran. 2017;31:62. doi: 10.18869/mjiri.31.62
- Ko Y-J, Kwon K-Y, Kum K-Y, et al. The anti-inflammatory effect of human telomerase-derived peptide on P. gingivalis lipopolysaccharide-induced inflammatory cytokine production and its mechanism in human dental pulp cells. Mediators Inflammation. 2015;2015:385127. doi: 10.1155/2015/385127
- Darveau RP, Pham TT, Lemley K, et al. Porphyromonas gingivalis lipopolysaccharide contains multiple lipid A species that functionally interact with both toll-like receptors 2 and 4. Infect Immun. 2004;72(9):5041-5051. doi: 10.1128/iai.72.9.5041-5051.2004