Melt-electrowriting of 3D anatomically relevant scaffolds to recreate a pancreatic acinar unit in vitro
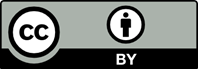
Melt-electrowriting (MEW) belongs to the group of advanced additive manufacturing techniques and consists of computer-aided design (CAD)-assisted polymer extrusion combined with a high-voltage supply to achieve deposition of polymeric fibers with diameters in the micrometric range (1 to 20 μm) similar to the size of natural extracellular matrix fibers. In this work, we exploit MEW to design and fabricate a three-dimensional (3D) model that resembles the morphology of the exocrine pancreatic functional unit without the need of supports, mandrels, or sacrificial materials. Optimized process parameters resulted in a MEW scaffold having regular fibers (19 ± 5 μm size) and an acinar cavity showing high shape fidelity. Then, human foreskin fibroblasts (HFF1) and human pancreatic ductal epithelial cells (HPDE), wild-type HPDE, and HPDE overexpressing KRAS oncogene were allowed to colonize the entire 3D structure and the acinar cavity. Thus, a physiologically relevant 3D model was created in vitro after 24 days using a co-culture protocol (14 days of HFF1 alone plus 10 days of HPDE and HFF1 co-culture). The effect of cell crosstalk within the MEW scaffolds was also assessed by monitoring HFF1 secretion of interleukin (IL)-6, a pro-inflammatory cytokine responsible for the inflammatory cascade occurring in pancreatic cancer. High levels of IL-6 were detected only when fibroblasts were co-cultured with the HPDE overexpressing KRAS. These findings confirmed that the MEW 3D in vitro model is able to recreate the characteristic hallmark of the pathological condition where cancer oncogenes mediate fibroblast activities.
- Hegyi P, Petersen OH. The exocrine pancreas: the acinar-ductal tango in physiology and pathophysiology. Rev Physiol Biochem Pharmacol. 2013;165:1-30. doi: 10.1007/112_2013_14
- Shih HP, Wang A, Sander M. Pancreas organogenesis: from lineage determination to morphogenesis. Annu Rev Cell Dev Biol. 2013;29:81-105. doi: 10.1146/annurev-cellbio-101512-122405
- Weniger M, Honselmann KC, Liss AS. The extracellular matrix and pancreatic cancer: a complex relationship. Cancers. 2018;10(9):316. doi: 10.3390/cancers10090316
- Fu Y, Liu S, Zeng S, Shen H. The critical roles of activated stellate cells-mediated paracrine signaling, metabolism and onco-immunology in pancreatic ductal adenocarcinoma. Mol Cancer. 2018;17:62. doi: 10.1186/s12943-018-0815-z
- Pothula SP, Pirola RC, Wilson JS, Apte MV. Pancreatic stellate cells: aiding and abetting pancreatic cancer progression. Pancreatology. 2020;20:409-418. doi: 10.1016/j.pan.2020.01.003
- Wang S, Li Y, Xing C, et al. Tumor microenvironment in chemoresistance, metastasis and immunotherapy of pancreatic cancer. Am J Cancer Res. 2020;10:1937-1953.
- Bynigeri RR, Jakkampudi A, Jangala R, et al. Pancreatic stellate cell: Pandora’s box for pancreatic disease biology. World J Gastroenterol. 2017;23:382. doi: 10.3748/wjg.v23.i3.382
- Apte MV, Wilson JS, Lugea A, Pandol SJ. A starring role for stellate cells in the pancreatic cancer microenvironment. Gastroenterology. 2013;144:1210-1219. doi: 10.1053/j.gastro.2012.11.037
- Wu Y, Zhang C, Jiang K, Werner J, Bazhin AV, D’Haese JG. The role of stellate cells in pancreatic ductal adenocarcinoma: targeting perspectives. Front Oncol. 2021;10. doi: 10.3389/fonc.2020.621937
- Zhan HX, Zhou B, Cheng Y, et al. Crosstalk between stromal cells and cancer cells in pancreatic cancer: new insights into stromal biology. Cancer Lett. 2017;392:83-93. doi: 10.1016/j.canlet.2017.01.041
- Cui SJ, Tang TY, Zou XW, Su Q-M, Feng L, Gong X-Y. Role of imaging biomarkers for prognostic prediction in patients with pancreatic ductal adenocarcinoma. Clin Radiol. 2020;75:478.e1-478.e11. doi: 10.1016/j.crad.2019.12.023
- Orth M, Metzger P, Gerum S, et al. Pancreatic ductal adenocarcinoma: Biological hallmarks, current status, and future perspectives of combined modality treatment approaches. Radiat Oncol. 2019;14(1):141. doi: 10.1186/s13014-019-1345-6
- Porciuncula A, Hajdu C, David G. The dual role of senescence in pancreatic ductal adenocarcinoma. Adv Cancer Res. 2016;131:1-20. doi: 10.1016/bs.acr.2016.05.006
- Brunet LR, Hagemann T, Andrew G, Mudan S, Marabelle A. Have lessons from past failures brought us closer to the success of immunotherapy in metastatic pancreatic cancer? Oncoimmunology. 2016;5(4). doi: 10.1080/2162402X.2015.1112942
- Cave DD, Rizzo R, Sainz B, Gigli G, Del Mercato LL, Lonardo E. The revolutionary roads to study cell–cell interactions in 3d in vitro pancreatic cancer models. Cancers (Basel). 2021;13(4):930. doi: 10.3390/cancers13040930
- Osuna de la Peña D, Trabulo S, Collin E, et al. Bioengineered 3D models of human pancreatic cancer recapitulate in vivo tumour biology. Nat Commun. 2021;12:5623. doi: 10.1038/s41467-021-25921-9
- Haque MR, Rempert TH, Al-Hilal TA, Wang C, Bhushan A, Bishehsari F. Organ-chip models: opportunities for precision medicine in pancreatic cancer. Cancers (Basel). 2021;13(17):4487. doi: 10.3390/cancers13174487
- Mollica H, Teo YJ, Tan DZ, et al. A 3D pancreatic tumor model to study T cell infiltration. Biomater Sci. 2021;9:7420-7431. doi: 10.1039/D1BM00210D
- Schuster B, Junkin M, Kashaf SS, et al. Automated microfluidic platform for dynamic and combinatorial drug screening of tumor organoids. Nat Commun. 2020;11:1-12. doi: 10.1038/s41467-020-19058-4
- Nguyen, DHT, Lee E, Alimperti S, et al. A biomimetic pancreatic cancer on-chip reveals endothelial ablation via ALK7 signaling. Sci Adv. 2019;5(8):eaav6789. doi: 10.1126/sciadv.aav6789
- Sgarminato V, Marasso SL, Cocuzza M, et al. PDAC-on-chip for in vitro modeling of stromal and pancreatic cancer cell crosstalk. Biomater Sci. 2022;11:208-224. doi: 10.1039/D2BM00881E
- Kota J, Hancock J, Kwon J, Korc M. Pancreatic cancer: stroma and its current and emerging targeted therapies. Cancer Lett. 2017;391:38-49. doi: 10.1016/j.canlet.2016.12.035
- Hajiabbas M, D’Agostino C, Simińska-Stanny J, Tran SD, Shavandi A, Delporte C. Bioengineering in salivary gland regeneration. J Biomed Sci. 2022;291(29):1-24. doi: 10.1186/s12929-022-00819-w
- Gupta P, Pérez-Mancera PA, Kocher H, Nisbet A, Schettino G, Velliou EG. A novel scaffold-based hybrid multicellular model for pancreatic ductal adenocarcinoma—toward a better mimicry of the in vivo tumor microenvironment. Front Bioeng Biotechnol. 2020;8:290. doi: 10.3389/fbioe.2020.00290
- Venis SM, Moon HR, Yang Y, Utturkar SM, Konieczny SF, Han B. Engineering of a functional pancreatic acinus with reprogrammed cancer cells by induced: PTF1a expression. Lab Chip. 2021;21:3675-3685. doi: 10.1039/d1lc00350j
- Charbonneau AM, Kinsella JM, Tran SD. 3D cultures of salivary gland cells in native or gelled egg yolk plasma, combined with egg white and 3D-printing of gelled egg yolk plasma. Materials (Basel). 2019;12(21):3480. doi: 10.3390/ma12213480
- Jain P, Kathuria H, Dubey N. Advances in 3D bioprinting of tissues/organs for regenerative medicine and in-vitro models. Biomaterials. 2022;287:121639. doi: 10.1016/j.biomaterials.2022.121639
- Loewner S, Heene S, Baroth T, et al. Recent advances in melt electro writing for tissue engineering for 3D printing of microporous scaffolds for tissue engineering. Front Bioeng Biotechnol. 2022;10. doi: 10.3389/fbioe.2022.896719
- Hutmacher DW, Dalton PD. Melt electrospinning. Chem Asian J. 2011;6:44-56. doi: 10.1002/asia.201000436
- Kade JC, Dalton PD. Polymers for melt electrowriting. Adv Healthc Mater. 2021;10(1):2001232. doi: 10.1002/adhm.202001232
- Cao K, Zhang F, Zaeri A, et al. Advances in design and quality of melt electrowritten scaffolds. Mater Des. 2023;1;226. doi: 10.1016/j.matdes.2023.111618
- Moroni L, Boland T, Burdick JA, et al. Biofabrication: a guide to technology and terminology. Trends Biotechnol. 2018;36:384-402. doi: 10.1016/j.tibtech.2017.10.015
- Fu Z, Naghieh S, Xu C, Wang C, Sun W, Chen X. Printability in extrusion bioprinting. Biofabrication. 2021;13(3): 033001. doi: 10.1088/1758-5090/abe7ab
- Licciardello M, Ciardelli G, Tonda-Turo C. Biocompatible electrospun polycaprolactone-polyaniline scaffold treated with atmospheric plasma to improve hydrophilicity. Bioengineering. 2021;8(2):24. doi: 10.3390/bioengineering8020024
- Mancuso E, Tonda-Turo C, Ceresa C, et al. Potential of Manuka honey as a natural polyelectrolyte to develop biomimetic nanostructured meshes with antimicrobial properties. Front Bioeng Biotechnol. 2019;7:344. doi: 10.3389/fbioe.2019.00344
- Giuntoli G, Muzio G, Actis C, et al. In-vitro characterization of a hernia mesh featuring a nanostructured coating. Front Bioeng Biotechnol. 2021;8:589223. doi: 10.3389/fbioe.2020.589223
- Backes, EH, Harb SV, Beatrice CA, et al. Polycaprolactone usage in additive manufacturing strategies for tissue engineering applications: a review. J Biomed Mater Res B Appl Biomater. 2022;110:1479-1503. doi: 10.1002/jbm.b.34997
- Großhaus C, Bakirci E, Berthel M, et al. Melt electrospinning of nanofibers from medical-grade poly(ε-caprolactone) with a modified nozzle. Small. 2020;16:2003471. doi: 10.1002/smll.202003471
- Kumar N, Joisher H, Ganguly A. Polymeric scaffolds for pancreatic tissue engineering: a review. Rev Diabet Stud. 2017;14:334-353. doi: 10.1900/RDS.2017.14.334
- Yang X, Wang Y, Zhou Y, Chen J, Wan Q. The application of polycaprolactone in three-dimensional printing scaffolds for bone tissue engineering. Polymers (Basel). 2021;13:2754. doi: 10.3390/polym13162754
- Abràmoff MD, Magalhães PJ, Ram SJ. Image processing with ImageJ. Biophotonics Int. 2004;11:36-42.
- Lee JH, Kim SK, Khawar IA, Jeong S-Y, Chung S, Kuh H-J. Microfluidic co-culture of pancreatic tumor spheroids with stellate cells as a novel 3D model for investigation of stroma-mediated cell motility and drug resistance. J Exp Clin Cancer Res. 2018;37:1-12. doi: 10.1186/s13046-017-0654-6
- Jeong SY, Lee JH, Shin Y, Chung S, Kuh H-J. Co-culture of tumor spheroids and fibroblasts in a collagen matrix-incorporated microfluidic chip mimics reciprocal activation in solid tumor microenvironment. PLoS One. 2016;11:e0159013. doi: 10.1371/journal.pone.0159013
- Fujiwara M, Kanayama K, Hirokawa YS, Shiraishi T. ASF- 4-1 fibroblast-rich culture increases chemoresistance and mTOR expression of pancreatic cancer BxPC-3 cells at the invasive front in vitro, and promotes tumor growth and invasion in vivo. Oncol Lett. 2016;11:2773. doi: 10.3892/ol.2016.4289
- Weeber F, Ooft SN, Dijkstra KK, Voest EE. Tumor organoids as a pre-clinical cancer model for drug discovery. Cell Chem Biol. 2017;24:1092-1100. doi: 10.1016/j.chembiol.2017.06.012
- Kapałczyńska M, Kolenda T, Przybyła W, et al. 2D and 3D cell cultures - a comparison of different types of cancer cell cultures. Arch Med Sci. 2018;14:910-919. doi: 10.5114/aoms.2016.63743
- Desoize B, Jardillier J. Multicellular resistance: a paradigm for clinical resistance? Crit Rev Oncol Hematol. 2000;36:193-207. doi: 10.1016/s1040-8428(00)00086-x
- Brancato V, Oliveira JM, Correlo VM, Reis RL, Kundu SC. Could 3D models of cancer enhance drug screening? Biomaterials. 2020;232:119744. doi: 10.1016/j.biomaterials.2019.119744
- Shichi Y, Norihiko S, Masaki M, et al. Enhanced morphological and functional differences of pancreatic cancer with epithelial or mesenchymal characteristics in 3D culture. Sci Rep. 2019;9:10871. doi: 10.1038/s41598-019-47416-w
- Fang Y, Eglen RM. Three-dimensional cell cultures in drug discovery and development. SLAS Discov. 2017;22:456-472. doi: 10.1177/1087057117696795
- Laschke MW, Menger MD. Life is 3D: boosting spheroid function for tissue engineering. Trends Biotechnol. 2017;35:133-144. doi: 10.1016/j.tibtech.2016.08.004
- Tomás-Bort E, Kieler M, Sharma S, Candido JB, Loessner D. 3D approaches to model the tumor microenvironment of pancreatic cancer. Theranostics. 2020;10:5074-5089. doi: 10.7150/thno.42441
- Monteiro MV, Ferreira LP, Rocha M, Gaspar VM, Mano JF. Advances in bioengineering pancreatic tumor-stroma physiomimetic biomodels. Biomaterials. 2022;287:121653. doi: 10.1016/j.biomaterials.2022.121653
- Bradney MJ, Venis SM, Yang Y, Konieczny SF, Han B. A biomimetic tumor model of heterogeneous invasion in pancreatic ductal adenocarcinoma. Small. 2020;16(10):e1905500. doi: 10.1002/smll.201905500
- Sung JH, Shuler ML. Microtechnology for mimicking in vivo tissue environment. Ann Biomed Eng. 2012;40:1289-1300. doi: 10.1007/s10439-011-0491-2
- Randriamanantsoa S, Papargyriou A, Maurer HC, et al. Spatiotemporal dynamics of self-organized branching in pancreas-derived organoids. Nat Commun. 2022;13:1-15. doi: 10.1038/s41467-022-32806-y
- Ushiki T. Collagen fibers, reticular fibers and elastic fibers. A comprehensive understanding from a morphological viewpoint. Arch Histol Cytol. 2002;65:109-126. doi: 10.1679/aohc.65.109
- Rajan K, Samykano M, Kadirgama K, Harun WSW, Rahman MM. Fused deposition modeling: process, materials, parameters, properties, and applications. Int J Adv Manuf Technol. 2022;120:1531-1570. doi: 10.1007/s00170-022-08860-7
- Bachs-Herrera A, Yousefzade O, Del Valle LJ, Puiggali J. Melt electrospinning of polymers: blends, nanocomposites, additives and applications. Appl Sci. 2021;11(4):1808. doi: 10.3390/app11041808
- Saidy NT, Shabab T, Bas O, et al. Melt electrowriting of complex 3D anatomically relevant scaffolds. Front Bioeng Biotechnol. 2020;8:793. doi: 10.3389/fbioe.2020.00793
- Peiffer QC, de Ruijter M, van Duijn J, et al. Melt electrowriting onto anatomically relevant biodegradable substrates: resurfacing a diarthrodial joint. Mater Des. 2020;195:109025. doi: 10.1016/j.matdes.2020.109025
- Brooks-Richards TL, Paxton NC, Allenby MC, Woodruff MA. Dissolvable 3D printed PVA moulds for melt electrowriting tubular scaffolds with patient-specific geometry. Mater Des. 2022;215:110466. doi: 10.1016/j.matdes.2022.110466
- Saha U, Nairn R, Keenan O, Monaghan MG. A deeper insight into the influence of the electric field strength when melt-electrowriting on non-planar surfaces. Macromol Mater Eng. 2021;306:2100496. doi: 10.1002/mame.202100496
- Constante, G, Apsite I, Alkhamis H, et al. 4D biofabrication using a combination of 3D printing and melt-electrowriting of shape-morphing polymers. ACS Appl Mater Interfaces. 2021;13:12767-12776. doi: 10.1021/acsami.0c18608
- Van Genderen AM, Jansen K, Kristen M, et al. Topographic guidance in melt-electrowritten tubular scaffolds enhances engineered kidney tubule performance. Front Bioeng Biotechnol. 2021;8:617364. doi: 10.3389/fbioe.2020.617364
- Liashenko I, Hrynevich A, Dalton PD. Designing outside the box: unlocking the geometric freedom of melt electrowriting using microscale layer shifting. Adv Mater. 2020;32:2001874. doi: 10.1002/adma.202001874
- Bakirci E, Schaefer N, Dahri O, et al. Melt electrowritten in vitro radial device to study cell growth and migration. Adv Biosyst. 2020;4:2000077. doi: 10.1002/adbi.202000077
- Korpershoek, JV, Korpershoek JV, Ruijter M, et al. Potential of melt electrowritten scaffolds seeded with meniscus cells and mesenchymal stromal cells. Int J Mol Sci. 2021;22:11200. doi: 10.3390/ijms222011200
- Neves SC, Moreira Teixeira LS, Moroni L, et al. Chitosan/ poly(ɛ-caprolactone) blend scaffolds for cartilage repair. Biomaterials. 2011;32:1068-1079. doi: 10.1016/j.biomaterials.2010.09.073
- Malakpour Permlid A, Roci P, Fredlund E, et al. Unique animal friendly 3D culturing of human cancer and normal cells. Toxicol In Vitro. 2019;60:51-60. doi: 10.1016/j.tiv.2019.04.022
- Kuen J, Darowski D, Kluge T, Majety M. Pancreatic cancer cell/fibroblast co-culture induces M2 like macrophages that influence therapeutic response in a 3D model. PLoS One. 2017;12:e0182039. doi: 10.1371/journal.pone.0182039
- van Duijneveldt G, Griffin MDW, Putoczki TL. Emerging roles for the IL-6 family of cytokines in pancreatic cancer. Clin Sci. 2020;134:2091-2115. doi: 10.1042/CS20191211
- Öhlund D, Handly-Santana A, Biffi G, et al. Distinct populations of inflammatory fibroblasts and myofibroblasts in pancreatic cancer. J Exp Med. 2017;214:579-596. doi: 10.1084/jem.20162024
- Feurino LW, Zhang Y, Bharadwaj U, et al. IL-6 stimulates Th2 type cytokine secretion and upregulates VEGF and NRP-1 expression in pancreatic cancer cells. Cancer Biol Ther. 2007;6:1096-1100. doi: 10.4161/cbt.6.7.4328
- Ancrile B, Lim KH, Counter CM. Oncogenic Ras-induced secretion of IL6 is required for tumorigenesis. Genes Dev. 2007;21:1714-1719. doi: 10.1101/gad.1549407
- Nagasaki T, Hara M, Nakanishi H, Takahashi H, Sato M, Takeyama H. Interleukin-6 released by colon cancer-associated fibroblasts is critical for tumour angiogenesis: anti-interleukin-6 receptor antibody suppressed angiogenesis and inhibited tumour–stroma interaction. Br J Cancer. 2014;110:469-478. doi: 10.1038/bjc.2013.748
- Ebrahimi B, Tucker SL, Li D, Abbruzzese JL, Kurzrock R. Cytokines in pancreatic carcinoma. Cancer. 2004;101: 2727-2736. doi: 10.1002/cncr.20672
- Wigmore SJ, Fearon KC, Sangster K, Maingay JP, Garden OJ, Ross JA. Cytokine regulation of constitutive production of interleukin-8 and -6 by human pancreatic cancer cell lines and serum cytokine concentrations in patients with pancreatic cancer. Int J Oncol. 2002;21:881-886. doi: 10.3892/ijo.21.4.881
- Talar-Wojnarowska, R, Gasiorowska A, Smolarz B, Romanowicz-Makowska H, Kulig A, Malecka-Panas E. Clinical significance of interleukin-6 (Il-6) gene polymorphism and Il-6 serum level in pancreatic adenocarcinoma and chronic pancreatitis. Dig Dis Sci. 2009;54:683-689. doi: 10.1007/s10620-008-0390-z
- Barber MD, Fearon KCH, Ross JA. Relationship of serum levels of interleukin-6, soluble interleukin-6 receptor and tumour necrosis factor receptors to the acute-phase protein response in advanced pancreatic cancer. Clin Sci. 1999;96:83-87.
- Okada S, Okusaka T, Ishii H, et al. Elevated serum interleukin-6 levels in patients with pancreatic cancer. Jpn J Clin Oncol. 1998;28:12-15. doi: 10.1093/jjco/28.1.12
- Błogowski W, Deskur A, Budkowska M, et al. Selected cytokines in patients with pancreatic cancer: a preliminary report. PLoS One. 2014;9:e97613. doi: 10.1371/journal.pone.0097613
- Mroczko B, Groblewska M, Gryko M, Kędra B, Szmitkowski M. Diagnostic usefulness of serum interleukin 6 (IL-6) and C-reactive protein (CRP) in the differentiation between pancreatic cancer and chronic pancreatitis. J Clin Lab Anal. 2010;24:256-261. doi: 10.1002/jcla.20395
- Chen K, Wang Q, Li M, et al. Single-cell RNA-seq reveals dynamic change in tumor microenvironment during pancreatic ductal adenocarcinoma malignant progression. EBioMedicine. 2021;66:103315. doi: 10.1016/j.ebiom.2021.103315
- Pothula SP, Pirola RC, Wilson JS, Apte MV. Pancreatic stellate cells: aiding and abetting pancreatic cancer progression. Pancreatology. 2020;20:409-418. doi: 10.1016/j.pan.2020.01.003
- Koikawa K, Ohuchida K, Ando Y, et al. Basement membrane destruction by pancreatic stellate cells leads to local invasion in pancreatic ductal adenocarcinoma. Cancer Lett. 2018;425:65-77. doi: 10.1016/j.canlet.2018.03.031
- Dufour A, Gallostra XB, O’Keeffe C, et al. Integrating melt electrowriting and inkjet bioprinting for engineering structurally organized articular cartilage. Biomaterials. 2022;283:121405. doi: 10.1016/j.biomaterials.2022.121405