Man vs. machine: Automated bioink mixing device improves reliability and reproducibility of bioprinting results compared to human operators
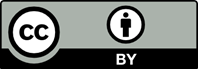
The bioink mixing process is highly relevant to the bioink quality, which is the basis for reproducible extrusion-based three-dimensional (3D) bioprinting (EBB). Currently, most bioinks mixed by skilled human operators show variations in terms of cell homogeneity and biological properties as well as other properties. For preparation of many types of bioinks, striking the balance between homogeneity and cell viability remains a major challenge. This study investigates the relationship between bioink homogeneity and mixing parameters, particularly mixing speed and number of exchanges, utilizing a customized automated device. We found that up to a certain point, increasing the rate of mixing led to a better distribution of cells within the bioink, but beyond that point, there was a detrimental effect on cell viability. In contrast, the mixing number had less impact on the physiological properties of the cells in the bioink. Furthermore, a comparison between skilled human and machine bioink mixing revealed that the machine consistently provided better outcomes in terms of bioink homogeneity, cell distribution, and cell viability, highlighting the advantages and importance of standardizing the bioink mixing process. The methodology and approaches in this study can improve the reproducibility and reliability of EBB bioink and may thereby advance the field of 3D bioprinting in various applications.
- Assad H, Assad A, Kumar A. Recent developments in 3D bio-printing and its biomedical applications. Pharmaceutics. 2023;15(1):255. doi: 10.3390/pharmaceutics15010255
- Hagenbuchner J, Nothdurfter D, Ausserlechner MJ. 3D bioprinting: novel approaches for engineering complex human tissue equivalents and drug testing. Essays Biochem. 2021;65(3):417-427. doi: 10.1042/ebc20200153
- Berg J, Kurreck J. Clean bioprinting - fabrication of 3D organ models devoid of animal components. ALTEX. 2021;38(2):269-288. doi: 10.14573/altex.2009151
- Bhattacharyya A, Janarthanan G, Tran HN, Ham HJ, Yoon J, Noh I. Bioink homogeneity control during 3D bioprinting of multicomponent micro/nanocomposite hydrogel for even tissue regeneration using novel twin screw extrusion system. Chem Eng J. 2021;415:128971. doi: 10.1016/j.cej.2021.128971
- Wang Y, Yuan X, Yao B, Zhu S, Zhu P, Huang S. Tailoring bioinks of extrusion-based bioprinting for cutaneous wound healing. Bioact. Mater. 2022;17:178-194. doi: 10.1016/j.bioactmat.2022.01.024
- Ainsworth MJ, Chirico N, de Ruijter M, et al. Convergence of melt electrowriting and extrusion-based bioprinting for vascular patterning of a myocardial construct. Biofabrication. 2023;15(3):035025. doi: 10.1088/1758-5090/ace07f
- Ozbolat IT, Hospodiuk M. Current advances and future perspectives in extrusion-based bioprinting. Biomaterials. 2016;76:321-343. doi: 10.1016/j.biomaterials.2015.10.076
- Kessel B, Lee M, Bonato A, Tinguely Y, Tosoratti E, Zenobi- Wong M. 3D bioprinting of macroporous materials based on entangled hydrogel microstrands. Adv Sci. 2016;7(18):2001419. doi: 10.1002/advs.202001419
- Karvinen J, Kellomäki M. Design aspects and characterization of hydrogel-based bioinks for extrusion-based bioprinting. Bioprinting. 2023;32:e00274. doi: 10.1016/j.bprint.2023.e00274
- Vu M, Pramanik A, Basak AK, Prakash C, Shankar S. Progress and challenges on extrusion based three dimensional (3D) printing of biomaterials. Bioprinting. 2022; 27:e00223. doi: 10.1016/j.bprint.2022.e00223
- Jia R, Zhang M, Yang T, Ma M, Sun Q, Li M. Evolution of the morphological, structural, and molecular properties of gluten protein in dough with different hydration levels during mixing. Food Chem: X. 2022;15:100448. doi: 10.1016/j.fochx.2022.100448
- Thayer PS, Orrhult LS, Martínez H. Bioprinting of cartilage and skin tissue analogs utilizing a novel passive mixing unit technique for bioink precellularization. JoVE. 2018;131(131):e56372. doi: 10.3791/56372
- Li G, Huang K, Deng J, et al. Highly conducting and stretchable double-network hydrogel for soft bioelectronics. Adv Mater. 2022;34(15):2200261. doi: 10.1002/adma.202200261
- Raja N, Park H, Gal CW, Sung A, Choi Y-J, Yun H-s. Support-less ceramic 3D printing of bioceramic structures using a hydrogel bath. Biofabrication. 2023; 15(3):035006. doi: 10.1088/1758-5090/acc903
- Zheng Z, Wu J, Liu M, et al. 3D bioprinting of self-standing silk-based bioink. Adv Healthc Mater. 2018;7(6):1701026. doi: 10.1002/adhm.201701026
- Guagliano G, Volpini C, Sardelli L, et al. Hep3Gel: a shape-shifting extracellular matrix- based, three-dimensional liver model adaptable to different culture systems. ACS Biomater Sci Eng. 2023;9(1):211-229. doi: 10.1021/acsbiomaterials.2c01226
- Dou Z, Tang H, Chen K, et al. Highly elastic and self-healing nanostructured gelatin/clay colloidal gels with osteogenic capacity for minimally invasive and customized bone regeneration. Biofabrication. 2023;15(2):025001. doi: 10.1088/1758-5090/acab36
- Berg J, Hiller T, Kissner MS, et al. Optimization of cell-laden bioinks for 3D bioprinting and efficient infection with influenza A virus. Sci Rep. 2018;8(1):13877. doi: 10.1038/s41598-018-31880-x
- Wu D, Berg J, Arlt B, et al. Bioprinted cancer model of neuroblastoma in a renal microenvironment as an efficiently applicable drug testing platform. Int J Mol Sci. 2022;23(1):122. doi: 10.3390/ijms23010122
- Dani S, Ahlfeld T, Albrecht F, et al. Homogeneous and reproducible mixing of highly viscous biomaterial inks and cell suspensions to create bioinks. Gels. 72021;(4):227. doi: 10.3390/gels7040227
- Cavallo A, Al Kayal T, Mero A, et al. Marine collagen-based bioink for 3D bioprinting of a bilayered skin model. Pharmaceutics. 2023;15(5):1331. doi: 10.3390/pharmaceutics15051331
- Gillispie G, Prim P, Copus J, et al. Assessment methodologies for extrusion-based bioink printability. Biofabrication. 2020;12(2):022003. doi: 10.1088/1758-5090/ab6f0d
- Kang D, Liu Z, Qian C, et al. 3D bioprinting of a gelatin-alginate hydrogel for tissue-engineered hair follicle regeneration. Acta Biomater. 2023;165:19-30. doi: 10.1016/j.actbio.2022.03.011
- Isaacson A, Swioklo S, Connon CJ. 3D bioprinting of a corneal stroma equivalent. Exp Eye Res. 2018;173:188-193. doi: 10.1016/j.exer.2018.05.010
- Cadena M, Ning L, King A, et al. 3D bioprinting of neural tissues. Adv Healthc Mater. 2021;10(15):2001600. doi: 10.1002/adhm.202001600
- Graham AD, Olof SN, Burke MJ, et al. High-resolution patterned cellular constructs by droplet-based 3D printing. Sci Rep. 2017;7(1):7004. doi: 10.1038/s41598-017-06358-x
- Pretorius V, Smuts TW. Turbulent flow chromatography. A new approach to faster analysis. Anal Chem. 1966;38(2):274-281. doi: 10.1021/ac60234a030
- Dimotakis PE. Turbulent mixing. Annu Rev Fluid Mech. 2005;37(1):329-356. doi: 10.1146/annurev.fluid.36.050802.122015
- Hiller T, Berg J, Elomaa L, et al. Generation of a 3D liver model comprising human extracellular matrix in an alginate/ gelatin-based bioink by extrusion bioprinting for infection and transduction studies. Int J Mol Sci. 2018;19(10):3129. doi: 10.3390/ijms19103129
- Berg J, Weber Z, Fechler-Bitteti M, et al. Bioprinted multi-cell type lung model for the study of viral inhibitors. Viruses. 2021;13(8):1590. doi: 10.3390/v13081590
- Al-Zeer MA, Prehn F, Fiedler S, et al. Evaluating the suitability of 3D bioprinted samples for experimental radiotherapy: a pilot study. Int J Mol Sci. 2022;23(17):9951. doi: 10.3390/ijms23179951
- Senturk E, Bilici C, Afghah F, et al. 3D bioprinting of tyramine modified hydrogels under visible light for osteochondral interface. Biofabrication. 2023;15(3):034102. doi: 10.1088/1758-5090/acd6bf
- Xin S, Deo KA, Dai J, et al. Generalizing hydrogel microparticles into a new class of bioinks for extrusion bioprinting. Sci Adv. 2021;7(42):eabk3087. doi: 10.1126/sciadv.abk3087
- Tibbitt MW, Anseth KS. Hydrogels as extracellular matrix mimics for 3D cell culture. Biotechnol Bioeng. 2009;103(4):655-663. doi: 10.1002/bit.22361
- Nam S, Stowers R, Lou J, Xia Y, Chaudhuri O. Varying PEG density to control stress relaxation in alginate-PEG hydrogels for 3D cell culture studies. Biomaterials. 2019;200:15-24. doi: 10.1016/j.biomaterials.2019.02.004
- Pasturel A, Strale P-O, Studer V. Tailoring common hydrogels into 3D cell culture templates. Adv Healthc Mater. 2020;9(18):2000519. doi: 10.1002/adhm.202000519
- Cui X, Li J, Hartanto Y, et al. Advances in extrusion 3D bioprinting: a focus on multicomponent hydrogel-based bioinks. Adv Healthc Mater. 2020;9(15):1901648. doi: 10.1002/adhm.201901648
- Unagolla JM, Jayasuriya AC. Hydrogel-based 3D bioprinting: a comprehensive review on cell-laden hydrogels, bioink formulations, and future perspectives. Appl Mater Today. 2020;18:100479. doi: 10.1016/j.apmt.2019.100479
- Ho T-C, Chang C-C, Chan H-P, et al. Hydrogels: properties and applications in biomedicine. Molecules. 2022;27(9):2902. doi: 10.3390/molecules27092902
- Puertas-Bartolomé M, Włodarczyk-Biegun MK, del Campo A, Vázquez-Lasa B, San Román J. 3D printing of a reactive hydrogel bio-ink using a static mixing tool. Polymers. 2020;12(9):1986. doi: 10.3390/polym12091986
- Jeon O, Lee YB, Lee SJ, Guliyeva N, Lee J, Alsberg E. Stem cell-laden hydrogel bioink for generation of high resolution and fidelity engineered tissues with complex geometries. Bioact Mater. 2022;15:185-193. doi: 10.1016/j.bioactmat.2021.11.025
- Semba JA, Mieloch AA, Tomaszewska E, Cywoniuk P, Rybka JD. Formulation and evaluation of a bioink composed of alginate, gelatin, and nanocellulose for meniscal tissue engineering. Int J Bioprint. 2023;9(1):621. doi: 10.18063/ijb.v9i1.621
- Pagan E, Stefanek E, Seyfoori A, et al. A handheld bioprinter for multi-material printing of complex constructs. Biofabrication. 2023;15(3):035012. doi: 10.1088/1758-5090/acc42c
- Mörö A, Samanta S, Honkamäki L, et al. Hyaluronic acid based next generation bioink for 3D bioprinting of human stem cell derived corneal stromal model with innervation. Biofabrication. 2023;15(1):015020. doi: 10.1088/1758-5090/acab34
- Hunsberger J, Simon C, Zylberberg C, et al. Improving patient outcomes with regenerative medicine: how the Regenerative Medicine Manufacturing Society plans to move the needle forward in cell manufacturing, standards, 3D bioprinting, artificial intelligence-enabled automation, education, and training. Stem Cells Transl Med. 2020;9(7):728-733. doi: 10.1002/sctm.19-0389
- Brown A. 3D printing in instructional settings: identifying a curricular hierarchy of activities. TechTrends. 2015; 59(5):16-24. doi: 10.1007/s11528-015-0887-1
- Boogert NJ, Madden JR, Morand-Ferron J, Thornton A. Measuring and understanding individual differences in cognition. Philos Trans R Soc Lond B Biol Sci. 2018;373(1756):20170280. doi: 10.1098/rstb.2017.0280
- Spurk JH, Aksel N, eds. Fluid Mechanics. Cham: Springer International Publishing; 2020: 223-249. doi: 10.1007/978-3-030-30259-7_7
- Li J, Shelby T, Alizadeh HV, Shelby H, Yang YP. Development and characterization of an automated active mixing platform for hydrogel bioink preparation. Int J Bioprint. 2023;9(4):4. doi: 10.18063/ijb.705
- Samokhin AS. Syringe pump created using 3D printing technology and arduino platform. J Anal Chem. 2020;75(3):416-421. doi: 10.1134/S1061934820030156
- Campbell T, Jones JFX. Design and implementation of a low cost, modular, adaptable and open-source XYZ positioning system for neurophysiology. HardwareX. 2020; 7:e00098. doi: 10.1016/j.ohx.2020.e00098
- Hadisujoto B, Wijaya R. Development and accuracy test of a fused deposition modeling (FDM) 3D printing using H-Bot mechanism. IJoCED. 2021;3(1):46-53. doi: 10.35806/ijoced.v3i1.148
- Manolescu VD, Secco EL. Design of an assistive low-cost 6 d.o.f. robotic arm with gripper. In: Yang XS, Sherratt S, Dey N, Joshi A, eds. Proceedings of Seventh International Congress on Information and Communication Technology. Singapore: Springer Nature Singapore; 2023: 39-55.
- Othman SA, Soon CF, Ma NL, et al. Alginate-gelatin bioink for bioprinting of hela spheroids in alginate-gelatin hexagon shaped scaffolds. Polym Bull. 2021;78(11):6115-6135. doi: 10.1007/s00289-020-03421-y
- Freeman FE, Pitacco P, van Dommelen LHA, et al. 3D bioprinting spatiotemporally defined patterns of growth factors to tightly control tissue regeneration. Sci Adv. 2020;6(33):eabb5093. doi: 10.1126/sciadv.abb5093
- Mirek A, Belaid H, Bartkowiak A, et al. Gelatin methacrylate hydrogel with drug-loaded polymer microspheres as a new bioink for 3D bioprinting. Biomater Adv. 2023;150:213436. doi: 10.1016/j.bioadv.2023.213436
- Seo JW, Kim GM, Choi Y, Cha JM, Bae H. Improving printability of digital-light-processing 3D bioprinting via photoabsorber pigment adjustment. Int J Mol Sci. 2022;23(10):5428. doi: 10.3390/ijms23105428
- Ding Y-W, Zhang X-W, Mi C-H, Qi X-Y, Zhou J, Wei D-X. Recent advances in hyaluronic acid-based hydrogels for 3D bioprinting in tissue engineering applications. Smart Mater Med. 2023;4:59-68. doi: 10.1016/j.smaim.2022.07.003
- Serafin A, Culebras M, Oliveira JM, Koffler J, Collins MN. 3D printable electroconductive gelatin-hyaluronic acid materials containing polypyrrole nanoparticles for electroactive tissue engineering. Adv Compos Hybrid Mater. 2023;6(3):109. doi: 10.1007/s42114-023-00665-w