3D-bioprinted hydrogels with instructive niches for dental pulp regeneration
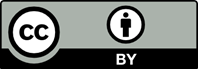
Infections to dental pulp commonly result in pulpitis and pulp necrosis, and surgical removal of the infected tissues is the only therapeutic approach. Dental pulp injury remains a challenging medical issue due to the limited regenerative capability of dental pulp. In this work, a dental pulp guidance construct (DPGC) with the instructive niche was bioprinted to mimic native teeth for dentin and neovascular-like structure reconstruction. GelMA-Dextran aqueous emulsion was used as an ink for in situ printing of porous DPGC to induce predominant nuclear localization of Yes-associated protein (YAP) in the encapsulated dental pulp stem cells (DPSCs) and enhance their stemness properties. Furthermore, the DPSCs encapsulated in DPGC with microporous structures exhibited enhanced viability, migration, and spreading. Meanwhile, we found that DPGC could promote capillary tube formation and induce neurogenesis. In a mouse subcutaneous implant model, the DPGC consisted of porous structures, such as odontoblasts and newly formed vascular structures, that mimic dental pulp characteristics. This study demonstrated a new strategy to design DPGC with instructive niche for dental pulp regeneration, presenting a potential treatment alternative to root canal therapy.
- Zhang W, Yelick PC. Tooth repair and regeneration: potential of dental stem cells. Trends Mol Med. 2021;27(5):501-511. doi: 10.1016/j.molmed.2021.02.005
- Nalliah RP, Allareddy V, Elangovan S, et al. Hospital emergency department visits attributed to pulpal and periapical disease in the United States in 2006. J Endod. 2011;37(1):6-9. doi: 10.1016/j.joen.2010.09.006
- Siddiqui Z, Acevedo-Jake AM, Griffith A, et al. Cells and material-based strategies for regenerative endodontics. Bioact Mater. 2022;14:234-249. doi: 10.1016/j.bioactmat.2021.11.015
- Goldberg F, Cantarini C, Alfie D, Macchi RL, Arias A. Relationship between unintentional canal overfilling and the long-term outcome of primary root canal treatments and nonsurgical retreatments: a retrospective radiographic assessment. Int Endod J. 2020;53(1):19-26. doi: 10.1111/iej.13209
- Wigsten E, Kvist T, Jonasson P, Davidson T. Comparing quality of life of patients undergoing root canal treatment or tooth extraction. J Endod. 2020;46(1):19-28.e1. doi: 10.1016/j.joen.2019.10.012
- Zipser CM, Cragg JJ, Guest JD, et al. Cell-based and stem-cell-based treatments for spinal cord injury: evidence from clinical trials. Lancet Neurol. 2022;21(7):659-670. doi: 10.1016/s1474-4422(21)00464-6
- Chen H, Fu H, Wu X, et al. Regeneration of pulpo-dentinal-like complex by a group of unique multipotent CD24a(+) stem cells. Sci Adv. 2020;6(15):eaay1514. doi: 10.1126/sciadv.aay1514
- Xuan K, Li B, Guo H, et al. Deciduous autologous tooth stem cells regenerate dental pulp after implantation into injured teeth. Sci Transl Med. 2018;10(455). doi: 10.1126/scitranslmed.aaf3227
- Liu S, Sun J, Yuan S, et al. Treated dentin matrix induces odontogenic differentiation of dental pulp stem cells via regulation of Wnt/β-catenin signaling. Bioact Mater. 2022;7:85-97. doi: 10.1016/j.bioactmat.2021.05.026
- Zhang R, Xie L, Wu H, et al. Alginate/laponite hydrogel microspheres co-encapsulating dental pulp stem cells and VEGF for endodontic regeneration. Acta Biomater. 2020;113:305-316.
doi: 10.1016/j.actbio.2020.07.012
- Yang T, Zhang Q, Xie L, et al. hDPSC-laden GelMA microspheres fabricated using electrostatic microdroplet method for endodontic regeneration. Mater Sci Eng C Mater Biol Appl. 2021;121:111850. doi: 10.1016/j.msec.2020.111850
- Kim SW, Im GB, Jeong GJ, et al. Delivery of a spheroids-incorporated human dermal fibroblast sheet increases angiogenesis and M2 polarization for wound healing. Biomaterials. 2021;275:120954. doi: 10.1016/j.biomaterials.2021.120954
- Soman SS, Vijayavenkataraman S. Applications of 3D bioprinted-induced pluripotent stem cells in healthcare. Int J Bioprint. 2020;6(4):280. doi: 10.18063/ijb.v6i4.280
- Yang L, Liu X, Song L, et al. Melatonin restores the pluripotency of long-term-cultured embryonic stem cells through melatonin receptor-dependent m6A RNA regulation. J Pineal Res. 2020;69(2):e12669. doi: 10.1111/jpi.12669
- Kim Y, Jin HJ, Heo J, et al. Small hypoxia-primed mesenchymal stem cells attenuate graft-versus-host disease. Leukemia. 2018;32(12):2672-2684. doi: 10.1038/s41375-018-0151-8
- Huebsch N, Lippens E, Lee K, et al. Matrix elasticity of void-forming hydrogels controls transplanted-stem-cell-mediated bone formation. Nat Mater. 2015;14(12): 1269-1277. doi: 10.1038/nmat4407
- Yuan Z, Yuan X, Zhao Y, et al. Injectable GelMA cryogel microspheres for modularized cell delivery and potential vascularized bone regeneration. Small. 2021;17(11):e2006596. doi: 10.1002/smll.202006596
- Ying G, Jiang N, Parra C, et al. Bioprinted injectable hierarchically porous gelatin methacryloyl hydrogel constructs with shape-memory properties. Adv Funct Mater. 2020;30(46):2003740. doi: 10.1002/adfm.202003740
- Guo Y, Xue Y, Wang P, et al. Muse cell spheroids have therapeutic effect on corneal scarring wound in mice and tree shrews. Sci Transl Med. 2020;12(562):eaaw1120. doi: 10.1126/scitranslmed.aaw1120
- Zhou L, Zeng Z, Liu S, et al. Multifunctional DNA hydrogel enhances stemness of adipose‐derived stem cells to activate immune pathways for guidance burn wound regeneration. Adv Funct Mater. 2022;32(46). doi: 10.1002/adfm.202207466
- Han M, Yang H, Lu X, et al. Three-dimensional-cultured MSC-derived exosome-hydrogel hybrid microneedle array patch for spinal cord repair. Nano Lett. 2022;22(15):6391- 6401. doi: 10.1021/acs.nanolett.2c02259
- Zhang J, Hu Q, Wang S, Tao J, Gou M. Digital light processing based three-dimensional printing for medical applications. Int J Bioprint. 2020;6(1):242. doi: 10.18063/ijb.v6i1.242
- Levato R, Jungst T, Scheuring RG, Blunk T, Groll J, Malda J. From shape to function: the next step in bioprinting. Adv Mater. 2020;32(12):e1906423. doi: 10.1002/adma.201906423
- Tao J, Liu H, Wu W, et al. 3D‐printed nerve conduits with live platelets for effective peripheral nerve repair. Adv Funct Mater. 2020;30(42). doi: 10.1002/adfm.202004272
- Tao J, Zhu S, Zhou N, et al. Nanoparticle-stabilized emulsion bioink for digital light processing based 3D bioprinting of porous tissue constructs. Adv Healthc Mater. 2022;11(12):e2102810. doi: 10.1002/adhm.202102810
- Shi S, Cui M, Sun F, et al. An innovative solvent-responsive coiling-expanding stent. Adv Mater. 2021;33(32):e2101005. doi: 10.1002/adma.202101005
- Lu X, Xu G, Lin Z, et al. Engineered exosomes enriched in netrin-1 modRNA promote axonal growth in spinal cord injury by attenuating inflammation and pyroptosis. Biomater Res. 2023;27(1):3. doi: 10.1186/s40824-023-00339-0
- Zhang X, Wu W, Huang Y, Yang X, Gou M. Antheraea pernyi silk fibroin bioinks for digital light processing 3D printing. Int J Bioprint. 2023;9(5):760. doi: 10.18063/ijb.760
- Zhang M, Yang F, Han D, et al. 3D bioprinting of corneal decellularized extracellular matrix: GelMA composite hydrogel for corneal stroma engineering. Int J Bioprint. 2023;9(5). doi: 10.18063/ijb.774
- Wu Y, Li M, Su H, Chen H, Zhu Y. Up-to-date progress in bioprinting of bone tissue. Int J Bioprint. 2023;9(1):628. doi: 10.18063/ijb.v9i1.628
- Zhang C, Ren Y, Kong W, et al. Photocurable 3D-printed PMBG/TCP biphasic scaffold mimicking vasculature for bone regeneration. Int J Bioprint. 2023;9(5):767. doi: 10.18063/ijb.767
- Tang Z, Zhou Y, Ma L, Li J. Flow performance of porous implants with different geometry: line, surface, and volume structures. Int J Bioprint. 2023;9(3):700. doi: 10.18063/ijb.700
- Yu C, Zhu W, Sun B, Mei D, Gou M, Chen S. Modulating physical, chemical, and biological properties in 3D printing for tissue engineering applications. Appl Phys Rev. 2018;5(4). doi: 10.1063/1.5050245
- Seetharaman S, Vianay B, Roca V, et al. Microtubules tune mechanosensitive cell responses. Nat Mater. 2022;21(3): 366-377. doi: 10.1038/s41563-021-01108-x
- Li C, Jin Y, Wei S, et al. Hippo signaling controls NLR family pyrin domain containing 3 activation and governs immunoregulation of mesenchymal stem cells in mouse liver injury. Hepatology. 2019;70(5):1714-1731. doi: 10.1002/hep.30700
- Moya IM, Halder G. Hippo-YAP/TAZ signalling in organ regeneration and regenerative medicine. Nat Rev Mol Cell Biol. 2019;20(4):211-226. doi: 10.1038/s41580-018-0086-y
- Lian M, Sun B, Han Y, et al. A low-temperature-printed hierarchical porous sponge-like scaffold that promotes cell-material interaction and modulates paracrine activity of MSCs for vascularized bone regeneration. Biomaterials. 2021;274:120841. doi: 10.1016/j.biomaterials.2021.120841
- Liu S, Tang L, Zhao X, et al. Yap promotes noncanonical Wnt signals from cardiomyocytes for heart regeneration. Circ Res. 2021;129(8):782-797. doi: 10.1161/CIRCRESAHA.121.318966
- Dey A, Varelas X, Guan KL. Targeting the Hippo pathway in cancer, fibrosis, wound healing and regenerative medicine. Nat Rev Drug Discov. 2020;19(7):480-494. doi: 10.1038/s41573-020-0070-z
- Jeanette H, Marziali LN, Bhatia U, et al. YAP and TAZ regulate Schwann cell proliferation and differentiation during peripheral nerve regeneration. Glia. 2021;69(4): 1061-1074. doi: 10.1002/glia.23949
- Liang H, Wang Y, Chen S, Liu Y, Liu Z, Bai J. Nano-hydroxyapatite bone scaffolds with different porous structures processed by digital light processing 3D printing. Int J Bioprint. 2022;8(1):502. doi: 10.18063/ijb.v8i1.502
- Hasturk O, Kaplan DL. Cell armor for protection against environmental stress: advances, challenges and applications in micro- and nanoencapsulation of mammalian cells. Acta Biomater. 2019;95:3-31. doi: 10.1016/j.actbio.2018.11.040
- Brusatin G, Panciera T, Gandin A, Citron A, Piccolo S. Biomaterials and engineered microenvironments to control YAP/TAZ-dependent cell behaviour. Nat Mater. 2018;17(12):1063-1075. doi: 10.1038/s41563-018-0180-8
- Chaudhuri O, Gu L, Darnell M, et al. Substrate stress relaxation regulates cell spreading. Nat Commun. 2015;6:6364. doi: 10.1038/ncomms7365
- Tang S, Ma H, Tu HC, Wang HR, Lin PC, Anseth KS. Adaptable fast relaxing boronate-based hydrogels for probing cell-matrix interactions. Adv Sci. 2018;5(9): 1800638. doi: 10.1002/advs.201800638
- Qian Y, Gong J, Lu K, et al. DLP printed hDPSC-loaded GelMA microsphere regenerates dental pulp and repairs spinal cord. Biomaterials. 2023;299. doi: 10.1016/j.biomaterials.2023.122137
- Guo S, Redenski I, Landau S, Szklanny A, Merdler U, Levenberg S. Prevascularized scaffolds bearing human dental pulp stem cells for treating complete spinal cord injury. Adv Healthc Mater. 2020;9(20). doi: 10.1002/adhm.202000974
- Sultan N, Amin LE, Zaher AR, Grawish ME, Scheven BA. Dental pulp stem cells stimulate neuronal differentiation of PC12 cells. Neural Regen Res. 2021;16(9):1821-1828. doi: 10.4103/1673-5374.306089
- Liu C, Fan L, Tian Z, et al. Self-curling electroconductive nerve dressing for enhancing peripheral nerve regeneration in diabetic rats. Bioact Mater. 2021;6(11):3892-3903. doi: 10.1016/j.bioactmat.2021.03.034
- Fang W, Yang M, Wang L, et al. Hydrogels for 3D bioprinting in tissue engineering and regenerative medicine: current progress and challenges. Int J Bioprint. 2023;9(5):759. doi: 10.18063/ijb.759
- Rodenhizer D, Gaude E, Cojocari D, et al. A three-dimensional engineered tumour for spatial snapshot analysis of cell metabolism and phenotype in hypoxic gradients. Nat Mater. 2016;15(2):227-234. doi: 10.1038/nmat4482
- Liu Y, Graves DT, Wang S. Development and clinical application of human mesenchymal stem cell drugs. Sci Bull. 2023;68(9):860-863. doi: 10.1016/j.scib.2023.03.050
- Rodriguez-Salvador M, Ruiz-Cantu L. Revealing emerging science and technology research for dentistry applications of 3D bioprinting. Int J Bioprint. 2019;5(1):170. doi: 10.18063/ijb.v5i1.170
- Chang PH, Chao HM, Chern E, Hsu SH. Chitosan 3D cell culture system promotes naïve-like features of human induced pluripotent stem cells: A novel tool to sustain pluripotency and facilitate differentiation. Biomaterials. 2021;268:120575. doi: 10.1016/j.biomaterials.2020.120575
- Lu X, Sun C, Chen L, et al. Stemness maintenance and massproduction of neural stem cells on poly L-lactic acid nanofibrous membrane based on piezoelectric effect. Small. 2022;18(13):e2107236. doi: 10.1002/smll.202107236
- Gao J, Wang H, Li M, et al. DLP-printed GelMA-PMAA scaffold for bone regeneration through endochondral ossification. Int J Bioprint. 2023;9(5):754. doi: 10.18063/ijb.754
- Salvador T, Oliveira MB, Mano JF. Leachable-free fabrication of hydrogel foams enabling homogeneous viability of encapsulated cells in large-volume constructs. Adv Healthc Mater. 2020;9(20):e2000543. doi: 10.1002/adhm.202000543
- Silvestro I, Sergi R, Scotto d’Abusco A, et al. Chitosan scaffolds with enhanced mechanical strength and elastic response by combination of freeze gelation, photo-crosslinking and freeze-drying. Carbohydr Polym. 2021;267:118156. doi: 10.1016/j.carbpol.2021.118156
- Yang XY, Chen LH, Li Y, Rooke JC, Sanchez C, Su BL. Hierarchically porous materials: synthesis strategies and structure design. Chem Soc Rev. 2017;46(2):481-558. doi: 10.1039/c6cs00829a
- Esquena J. Water-in-water (W/W) emulsions. Curr Opin Colloid Interface Sci. 2016;25:109-119. doi: 10.1016/j.cocis.2016.09.010
- Hu Q, Lu Y, Luo Y. Recent advances in dextran-based drug delivery systems: from fabrication strategies to applications. Carbohydr Polym. 2021;264:117999. doi: 10.1016/j.carbpol.2021.117999
- Wu X, Zhu H, Che J, Xu Y, Tan Q, Zhao Y. Stem cell niche-inspired microcarriers with ADSCs encapsulation for diabetic wound treatment. Bioact Mater. 2023;26:159-168. doi: 10.1016/j.bioactmat.2023.02.031
- Wilson KL, Pérez SCL, Naffaa MM, Kelly SH, Segura T. Stoichiometric post-modification of hydrogel microparticles dictates neural stem cell fate in microporous annealed particle scaffolds. Adv Mater. 2022;34(33):e2201921. doi: 10.1002/adma.202201921
- Zhao Y, Shi Y, Yang H, et al. Stem cell microencapsulation maintains stemness in inflammatory microenvironment. Int J Oral Sci. 2022;14(1):48. doi: 10.1038/s41368-022-00198-w
- Kong Y, Ma B, Liu F, et al. Cellular stemness maintenance of human adipose-derived stem cells on ZnO nanorod arrays. Small. 2019;15(51):e1904099. doi: 10.1002/smll.201904099
- Kim J, Kim YM, Song SC. One-step preparation of an injectable hydrogel scaffold system capable of sequential dual-growth factor release to maximize bone regeneration. Adv Healthc Mater. 2023;12(4):e2202401. doi: 10.1002/adhm.202202401
- Madl CM, LeSavage BL, Khariton M, Heilshorn SC. Neural progenitor cells alter chromatin organization and neurotrophin expression in response to 3D matrix degradability. Adv Healthc Mater. 2020;9(18):e2000754. doi: 10.1002/adhm.202000754
- Bhattacharya S, Mukherjee A, Pisano S, et al. The biophysical property of the limbal niche maintains stemness through YAP. Cell Death Differ. 2023;30(6):1601-1614. doi: 10.1038/s41418-023-01156-7
- Gjorevski N, Sachs N, Manfrin A, et al. Designer matrices for intestinal stem cell and organoid culture. Nature. 2016;539(7630):560-564. doi: 10.1038/nature20168
- Atlas Y, Gorin C, Novais A, et al. Microvascular maturation by mesenchymal stem cells in vitro improves blood perfusion in implanted tissue constructs. Biomaterials. 2021;268:120594. doi: 10.1016/j.biomaterials.2020.120594
- Gorin C, Rochefort GY, Bascetin R, et al. Priming dental pulp stem cells with fibroblast growth factor-2 increases angiogenesis of implanted tissue-engineered constructs through hepatocyte growth factor and vascular endothelial growth factor secretion. Stem Cells Transl Med. 2016;5(3):392-404. doi: 10.5966/sctm.2015-0166
- Xu D, Wu D, Qin M, et al. Efficient delivery of nerve growth factors to the central nervous system for neural regeneration. Adv Mater. 2019;31(33):e1900727. doi: 10.1002/adma.201900727
- Jiang S, Lyu C, Zhao P, et al. Cryoprotectant enables structural control of porous scaffolds for exploration of cellular mechano-responsiveness in 3D. Nat Commun. 2019;10(1). doi: 10.1038/s41467-019-11397-1
- Zhang J, Chen Y, Huang Y, et al. A 3D-printed self-adhesive bandage with drug release for peripheral nerve repair. Adv Sci. 2020;7(23):2002601. doi: 10.1002/advs.202002601
- Khayat A, Monteiro N, Smith EE, et al. GelMA-encapsulated hDPSCs and HUVECs for dental pulp regeneration. J Dent Res. 2017;96(2):192-199. doi: 10.1177/0022034516682005