3D bioprinting of anisotropic filler-reinforced polymer nanocomposites: Synthesis, assembly, and multifunctional applications
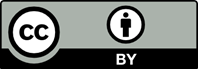
Bioprinting is a novel technique with a wide range of potential uses, including the fabrication of functioning tissue constructs for use in the biomedical sectors. It is a revolutionary method for high-throughput manufacturing that automates fine control over manufactured structures. Bioink refers to the solution of biomaterials usually encapsulating cells used in the bioprinting process; this bioink often encapsulates the appropriate cell types. In order to create the ultimate architecture, this bioink should solidify during or shortly after bioprinting. Bioinks can be developed from either all-natural or all-synthetic biomaterials, or a blend of the two. Cell aggregation can occasionally be used as a bioink without addition of any biomaterials, in bioprinting process. To bioprint functional tissues and organs, an optimal bioink should possess mechanical, rheological, and biological characteristics mimicking those of the target tissues. For attaining physicomechanical properties, anisotropic fillers are commonly added in bioink formulations. In this review, we provide an in-depth discussion of various anisotropic fillers used in bioprinting and their fabrication techniques, and outline their multifunctional applicability in biomedical and environmental areas. Given the steady growth of bioprinting market, we also present the global scenario of the bioprinting market and their techno-commercial orientations.
- Leberfinger AN, Dinda S, Wu Y, et al. Bioprinting functional tissues. Acta Biomater. 2019;95, 32-49.
- Dutton S, Kelly D, Baker A. Composite Materials for Aircraft Structures. Reston: AIAA; 2004. doi: 10.2514/4.861680
- Clyne TW, Hull D. An Introduction to Composite Materials. Cambridge: Cambridge University Press; 2019. doi: 10.1017/9781139050586
- Chang JH, An YU. Nanocomposites of polyurethane with various organoclays: thermomechanical properties, morphology, and gas permeability. J Polym Sci B Polym Phys. 2002;40(7):670-677. doi: 10.1002/polb.10124
- Ramanathan T, Abdala A, Stankovich S, et al. Functionalized graphene sheets for polymer nanocomposites. Nat Nanotechnol. 2008;3(6):327-331. doi: 10.1038/nnano.2008.96
- Potts JR, Dreyer DR, Bielawski CW, Ruoff RS. Graphene-based polymer nanocomposites. Polymer. 2011; 52(1):5-25. doi: 10.1016/j.polymer.2010.11.042
- Lavrador P, Esteves MR, Gaspar VM, Mano JF. Stimuli-responsive nanocomposite hydrogels for biomedical applications. Adv Funct Mater. 2021;31(8):2005941. doi: 10.1002/adfm.202005941
- Guvendiren M, Molde J, Soares RM, Kohn J. Designing biomaterials for 3D printing. ACS Biomater Sci Eng. 2016;2(10):1679-1693. doi: 10.1021/acsbiomaterials.6b00121
- Gungor-Ozkerim PS, Inci I, Zhang YS, Khademhosseini A, Dokmeci MR. Bioinks for 3D bioprinting: an overview. Biomater Sci. 2018;6(5):915-946. doi: 10.1039/C7BM00765E
- Armstrong JP, Burke M, Carter BM, Davis SA, Perriman AW. 3D bioprinting using a templated porous bioink. Adv Healthc Mater. 2016;5(14):1724-1730. doi: 10.1002/adhm.201600022
- Holmström J, Partanen J, Tuomi J, Walter M. Rapid manufacturing in the spare parts supply chain: alternative approaches to capacity deployment. J Manuf Technol Manag. 2010;21(6):687-697. doi: 10.1108/17410381011063996
- Kalsoom U, Nesterenko PN, Paull B. Recent developments in 3D printable composite materials. RSC Adv. 2016;6(65):60355-60371. doi: 10.1039/C6RA11334F
- Mythri G, Kavitha K, Kumar MR, Jagadeesh Singh SD. Novel mucoadhesive polymers – a review. J Appl Pharm Sci. 2011;1(8):37-42.
- Gao G, Kim BS, Jang J, Cho D-W. Recent strategies in extrusion-based three-dimensional cell printing toward organ biofabrication. ACS Biomater Sci Eng. 2019;5(3):1150-1169. doi: 10.1021/acsbiomaterials.8b00691
- Kang H-W, Lee SJ, Ko IK, Kengla C, Yoo JJ, Atala A. A 3D bioprinting system to produce human-scale tissue constructs with structural integrity. Nat Biotechnol. 2016;34(3):312-319. doi: 10.1038/nbt.3413
- Mazzocchi A, Devarasetty M, Huntwork R, Soker S, Skardal A. Optimization of collagen type I-hyaluronan hybrid bioink for 3D bioprinted liver microenvironments. Biofabrication. 2018;11(1):015003. doi: 10.1088/1758-5090/aae543
- Isaacson A, Swioklo S, Connon CJ. 3D bioprinting of a corneal stroma equivalent. Exp Eye Res. 2018;173:188-193. doi: 10.1016/j.exer.2018.05.010
- Liu W, Heinrich MA, Zhou Y, et al. Extrusion bioprinting of shear-thinning gelatin methacryloyl bioinks. Adv Healthc Mater. 2017;6(12):1601451. doi: 10.1002/adhm.201601451
- Scott EA, Stano A, Gillard M, Maio-Liu AC, Swartz MA, Hubbell JA. Dendritic cell activation and T cell priming with adjuvant-and antigen-loaded oxidation-sensitive polymersomes. Biomaterials. 2012;33(26):6211-6219. doi: 10.1016/j.biomaterials.2012.04.060
- Mueller J, Skylar-Scott M, Visser C, Lewis JA. Voxelated soft matter via multimaterial multinozzle 3D printing. Nature. 2019;575(7782):330-335. doi: 10.1038/s41586-019-1736-8
- Krishtul S, Baruch L, Machluf M. Processed tissue-derived extracellular matrices: tailored platforms empowering diverse therapeutic applications. Adv Funct Mater. 2020;30(18):1900386. doi: 10.1002/adfm.201900386
- Guo H, Zhao H, Niu H, et al. Highly thermally conductive 3D printed graphene filled polymer composites for scalable thermal management applications. ACS Nano. 2021;15(4):6917-6928. doi: 10.1021/acsnano.0c10768
- Matai I, Kaur G, Seyedsalehi A, McClinton A, Laurencin CT. Progress in 3D bioprinting technology for tissue/organ regenerative engineering. Biomaterials. 2020;226:119536. doi: 10.1016/j.biomaterials.2019.119536
- Vikram SA, Hasan DAM, Wang S, et al. The adoption of three-dimensional additive manufacturing from biomedical material design to 3D organ printing. Appl Sci. 2019;9(4):811. doi: 10.3390/app9040811
25 Fedorovich NE, Alblas J, de Wijn JR, Hennink WE, Verbout AJ, Dhert WJA. Hydrogels as extracellular matrices for skeletal tissue engineering: state-of-the-art and novel application in organ printing. Tissue Eng. 2007;13(8):1905-1925. doi: 10.1089/ten.2006.0175
- Miri AK, Mirzaee I, Hassan S, et al. Effective bioprinting resolution in tissue model fabrication. Lab Chip. 2019;19(11):2019-2037. doi: 10.1039/c8lc01037d
- Lee A, Hudson A, Shiwarski D, et al. 3D bioprinting of collagen to rebuild components of the human heart. Science. 2019;365(6452):482-487. doi: 10.1126/science.aav9051
- Mehrotra S, Moses JC, Bandyopadhyay A, Mandal BB. 3D printing/bioprinting based tailoring of in vitro tissue models: recent advances and challenges. ACS Appl Bio Mater. 2019;2(4):1385-1405. doi: 10.1021/acsabm.9b00073
- Jin Y, Liu C, Chai W, Compaan A, Huang Y. Self-supporting nanoclay as internal scaffold material for direct printing of soft hydrogel composite structures in air. ACS Appl Mater Interfaces. 2017;9(20):17456-17465. doi: 10.1021/acsami.7b03613
- García-Villén F, Ruiz-Alonso S, Lafuente-Merchan M, et al. Clay minerals as bioink ingredients for 3D printing and 3D bioprinting: application in tissue engineering and regenerative medicine. Pharmaceutics. 2021;13(11):1806. doi: 10.3390/pharmaceutics13111806
- Zhou Y, LaChance AM, Smith AT, Cheng H, Liu Q, Sun L. Strategic design of clay-based multifunctional materials: from natural minerals to nanostructured membranes. Adv Funct Mater. 2019;29(16):1807611. doi: 10.1002/adfm.201807611
- Ahlfeld T, Cidonio G, Kilian D, et al. Development of a clay-based bioink for 3D cell printing for skeletal application. Biofabrication. 2017;9(3):034103. doi: 10.1088/1758-5090/aa7e96
- Cidonio G, Glinka M, Kim YH, et al. Nanoclay-based 3D printed scaffolds promote vascular ingrowth ex vivo and generate bone mineral tissue in vitro and in vivo. Biofabrication. 2020;12(3):035010. doi: 10.1088/1758-5090/ab8753
- Leu Alexa R, Ianchis R, Savu D, et al. 3D printing of alginate-natural clay hydrogel-based nanocomposites. Gels. 2021;7(4):211. doi: 10.3390/gels7040211
- Habib A, Khoda B. Development of clay based novel hybrid bio-ink for 3D bio-printing process. J Manuf Process. 2019;38:76-87. doi: 10.1016/j.jmapro.2018.12.034
- Cui ZK, Kim S, Baljon JJ, Wu BM, Aghaloo T, Lee M. Microporous methacrylated glycol chitosan-montmorillonite nanocomposite hydrogel for bone tissue engineering. Nat Commun. 2019;10(1):1-10. doi: 10.1038/s41467-019-11511-3
- Ding F, Liu J, Zeng S, et al. Biomimetic nanocoatings with exceptional mechanical, barrier, and flame-retardant properties from large-scale one-step coassembly. Sci Adv. 2017;3(7):e1701212. doi: 10.1126/sciadv.1701212
- Sun L, Parker ST, Syoji D, Wang X, Lewis JA, Kaplan DL. Directnarker ST, Syoji D, adv.1701212nal mechanical, barrier, and flame-retardanAdv Healthc Mater. 2012;1(6):729-735. doi: 10.1002/adhm.201200057
- Michna S, Wu W, Lewis JA. Concentrated hydroxyapatite inks for direct-write assembly of 3-D periodic scaffolds. Biomaterials. 2005;26(28):5632-5639. doi: 10.1016/j.biomaterials.2005.02.040
- Zhang T, Yan KC, Ouyang L, Sun W. Mechanical characterization of bioprinted in vitro soft tissue models. Biofabrication. 2013;5(4):045010. doi: 10.1088/1758-5082/5/4/045010
- Gao G, He Y, Fu J-z, Liu A, Ma L. Coaxial nozzle-assisted 3D bioprinting with built-in microchannels for nutrients delivery. Biomaterials. 2015;61:203-215. doi: 10.1016/j.biomaterials.2015.05.031
- Ozbolat IT, Peng W, Ozbolat V. Application areas of 3D bioprinting. Drug Discov Today. 2016;21(8):1257-1271. doi: 10.1016/j.drudis.2016.04.006
- Jia W, Gungor-Ozkerim PS, Zhang YS, et al. Direct 3D bioprinting of perfusable vascular constructs using a blend bioink. Biomaterials. 2016;106:58-68. doi: 10.1016/j.biomaterials.2016.07.038
- Florczyk SJ, Simon MH, Juba D, et al. A bioinformatics 3D cellular morphotyping strategy for assessing biomaterial scaffold niches. ACS Biomater Sci Eng. 2017;3(10):2302-2313. doi: 10.1021/acsbiomaterials.7b00473
- Skylar-Scott MA, Uzel SG, Nam LL, et al. Biomanufacturing of organ-specific tissues with high cellular density and embedded vascular channels. Sci Adv. 2019;5(9): eaaw2459. doi: 10.1126/sciadv.aaw2459
- Kolesky DB, Truby RL, Gladman AS, Busbee TA, Homan KA, Lewis JA. 3D bioprinting of vascularized, heterogeneous cellgh cellular density and emAdv Mater. 2014;26(19):3124-3130. doi: 10.1002/adma.201305506
- Wu W, DeConinck A, Lewis JA. Omnidirectional printing of 3D microvascular networks. Adv Mater. 2011;23(24):H178-H183. doi: 10.1002/adma.201004625
- Bhattacharjee T, Zehnder SM, Rowe KG, et al. Writing in the granular gel medium. Sci Adv. 2015;1(8):e1500655. doi: 10.1126/sciadv.1500655
- Hinton TJ, Jallerat Q, Palchesko RN, et al. Three-dimensional printing of complex biological structures by freeform reversible embedding of suspended hydrogels. Sci Adv. 2015;1(9):e1500758. doi: 10.1126/sciadv.1500758
- O’Bryan CS, Bhattacharjee T, Hart S, et al. Self-assembled micro-organogels for 3D printing silicone structures. Sci Adv. 2017;3(5):e1602800. doi: 10.1126/sciadv.1602800
- Choi Y-J, Jun Y-J, Kim DY, et al. A 3D cell printed muscle construct with tissue-derived bioink for the treatment of volumetric muscle loss. Biomaterials. 2019;206:160-169. doi: 10.1016/j.biomaterials.2019.03.036
- Jeon O, Lee YB, Jeong H, Lee SJ, Wells D, Alsberg E. Individual cell-only bioink and photocurable supporting medium for 3D printing and generation of engineered tissues with complex geometries. Mater Horiz. 2019;6(8):1625-1631. doi: 10.1039/c9mh00375d
- Ouyang L. Pushing the rheological and mechanical boundaries of extrusion-based 3D bioprinting. Trends Biotechnol. 2022;40(7):P891-902. doi: 10.1016/j.tibtech.2022.01.001
- Siacor FDC, Chen Q, Zhao JY, et al. On the additive manufacturing (3D printing) of viscoelastic materials and flow behavior: from composites to food manufacturing. Addit Manuf. 2021;45:102043. doi: 10.1016/j.addma.2021.102043
- Golzar H, Wu Y, Ganguly S, Tang XS. Micro-extrusion 3D printing of articular cartilage substitutes with a multizonal structure using hydrophilic and rapidly curing silicone-based ink materials. Addit Manuf. 2023;73:103691. doi: 10.1016/j.addma.2023.103691
- Dealy J, Tee T, Petersen J. A concentric-cylinder rheometer for polymer melts. In: Vallet G, Meskat W, eds. Rheological Theories Measuring Techniques in Rheology Test Methods in Rheology Fractures Rheological Properties of Materials Rheo- Optics Biorheology. Heidelberg: Steinkopff; 1975:466-474. doi: 10.1007/978-3-662-41458-3_69
- Tang S, Richardson BM, Anseth KS. Dynamic covalent hydrogels as biomaterials to mimic the viscoelasticity of soft tissues. Prog Mater Sci. 2021;120:100738. doi: 10.1016/j.pmatsci.2020.100738
- Shitikova MV, Krusser AI. Models of viscoelastic materials: a review on historical development and formulation. In: Giorgio I, Placidi L, Barchiesi E, Abali BE, Altenbach H, eds. Theoretical Analyses, Computations, and Experiments of Multiscale Materials: A Tribute to Francesco dell’Isola. Cham: Springer Nature; 2022: 285-326. doi: 10.1007/978-3-031-04548-6
- Pirani SI, Krishnamachari P, Hashaikeh R. Optimum loading level of nanoclay in PLA nanocomposites: impact on the mechanical properties and glass transition temperature. J Thermoplast Compos Mater. 2014;27(11):1461-1478. doi: 10.1177/0892705712473627
- Skylar-Scott MA, Mueller J, Visser CW, Lewis JA. Voxelated soft matter via multimaterial multinozzle 3D printing. Nature. 2019;575(7782):330-335. doi: 10.1038/s41586-019-1736-8
- Ganguly S, Das P, Das TK, et al. Acoustic cavitation assisted destratified clay tactoid reinforced in situ elastomer-mimetic semi-IPN hydrogel for catalytic and bactericidal application. Ultrason Sonochem. 2020;60:104797. doi: 10.1016/j.ultsonch.2019.104797
- Ganguly S, Das NC. Water uptake kinetics and control release of agrochemical fertilizers from nanoclay-assisted semi-interpenetrating sodium acrylate-based hydrogel. Polym Plast Technol and Eng. 2017;56(7):744-761. doi: 10.1080/03602559.2016.1233268
- Ganguly S, Das TK, Mondal S, Das NC. Synthesis of polydopamine-coated halloysite nanotube-based hydrogel for controlled release of a calcium channel blocker. RSC Adv. 2016;6(107):105350-105362. doi: 10.1039/C6RA24153K
- Weng Z, Wang J, Senthil T, Wu L. Mechanical and thermal properties of ABS/montmorillonite nanocomposites for fused deposition modeling 3D printing. Mater Des. 2016;102:276-283. doi: 10.1016/j.matdes.2016.04.045
- Cui ZK, Kim S, Baljon JJ, Wu BM, Aghaloo T, Lee M. Microporous methacrylated glycol chitosan-montmorillonite nanocomposite hydrogel for bone tissue engineering. Nat Commun. 2019;10(1):3523. doi: 10.1038/s41467-019-11511-3
- Ganguly S, Das P. Synthesis and production of polylactic acid (PLA). In: Parameswaranpillai J, Siengchin S, Salim NV, George JJ, Poulose A, eds. Polylactic Acid-Based Nanocellulose and Cellulose Composites. Boca Raton: CRC Press; 2022: 29-50. doi: 10.1201/9781003160458
- Paspali A, Bao Y, Gawne DT, Piestert F, Reinelt S. The influence of nanostructure on the mechanical properties of 3D printed polylactide/nanoclay composites. Compos B Eng. 2018;152:160-168. doi: 10.1016/j.compositesb.2018.07.005
- Abdul Haq RH, Wahab MS, Wahid MU. Fused deposition modeling of PCL/HA/MMT biocompatible polymer nano-composites. In: Öchsner A, Altenbach H, eds. Machining, Joining and Modifications of Adv Mater. Singapore: Springer; 2016: 23-32. doi: 10.1007/978-981-10-1082-8
- Mohan VB, Lau KT, Hui D, Bhattacharyya D. Graphene-based materials and their composites: a review on production, applications and product limitations. Compos B Eng. 2018;142:200-220. doi: 10.1016/j.compositesb.2018.01.013
- Terrones M, Martín O, González M, et al. Interphases in graphene polymer, esb.2018.01.013 review on production, applicationsAdv Mater. 2011;23(44):5302-5310. doi: 10.1002/adma.201102036
- Ganguly S. Preparation/processing of polymer-graphene composites by different techniques. In: Rahaman M, Nayak L, Hussein IA, Das NC, eds. Polymer Nanocomposites Containing Graphene. USA: Elsevier; 2022: 45-74. doi: 10.1016/B978-0-12-821639-2.00015-X
- Zaman A, Orasugh JT, Banerjee P, et al. Facile one-pot in-situ synthesis of novel graphene oxide-cellulose nanocomposite for enhanced azo dye adsorption at optimized conditions. Carbohydr Polym. 2020;246:116661. doi: 10.1016/j.carbpol.2020.116661
- Ganguly S, Mondal S, Das P, et al. An insight into the physico-mechanical signatures of silylated graphene oxide in poly (ethylene methyl acrylate) copolymeric thermoplastic matrix. Macromol Res. 2019;27:268-281. doi: 10.1007/s13233-019-7039-y
- Du J, Cheng HM. The fabrication, properties, and uses of graphene/polymer composites. Macromol Chem Phys. 2012;213(10(10ol1060-1077. doi: 10.1002/macp.201200029
- Dai JF, Wang GJ, Ma L, Wu C-K. Surface properties of graphene: relationship to graphene-polymer composites. Rev Adv Mater Sci. 2015;40(1):60-71.
- Khezri B, Villa K, Novotný F, Sofer Z, Pumera M. Smartdust 3Dla K, Novotný F, . 00029 of graphene/polymer composites. e oxide in poly (ethyleneSmall. 2020;16(33):2002111. doi: 10.1002/smll.202002111
- Wei X, Li D, Jiang W, et al. 3D printable graphene composite. Sci Rep. 2015;5(1):1-7. doi: 10.1038/srep11181
- Chen S, Wu Q, Mishra C, et al. Thermal conductivity of isotopically modified graphene. Nat Mater. 2012;11(3):203-207. doi: 10.1038/nmat3207
- Chen Q, Mangadlao JD, Wallat J, De Leon A, Pokorski JK, Advincula RC. 3D printing biocompatible polyurethane/poly(lactic acid)/graphene oxide nanocomposites: anisotropic properties. ACS Appl Mater Interfaces. 2017;9(4):4015-4023. doi: 10.1021/acsami.6b11793
- Iijima S. Helical microtubules of graphitic carbon. Nature. 1991;354(6348):56-58. doi: 10.1038/354056a0
- Stauffer D, Aharony A. Introduction to Percolation Theory. London: Taylor & Francis; 2018. doi: 10.1201/9781315274386
- Pang H, Xu L, Yan DX, Li Z-M. Conductive polymer composites with segregated structures. Prog Polym Sci. 2014;39(11):1908-1933. doi: 10.1016/j.progpolymsci.2014.07.007
- Gnanasekaran K, Heijmans T, Van Bennekom S, et al. 3D printing of CNT-and graphene-based conductive polymer nanocomposites by fused deposition modeling. Appl Mater Today. 2017;9:21-28. doi: 10.1016/j.apmt.2017.04.003
- Gonzalez G, Chiappone A, Roppolo I, et al. Development of 3D printable formulations containing CNT with enhanced electrical properties. Polymer. 2017;109:246-253. doi: 10.1016/j.polymer.2016.12.051
- Shin SR, Bae H, Cha JM, et al. Carbon nanotube reinforced hybrid microgels as scaffold materials for cell encapsulation. ACS Nano. 2012;6(1):362-272. doi: 10.1021/nn203711s
- Lee J, Manoharan V, Cheung L, et al. Nanoparticle-based hybrid scaffolds for deciphering the role of multimodal cues in cardiac tissue engineering. ACS Nano. 2019;13(11):12525-12539. doi: 10.1021/acsnano.9b03050
- Li T, Chen T, Shen X, Shi HH, Jabaria E, Naguib HE. A binder jet 3D printed MXene composite for strain sensing and energy storage application. Nanoscale Adv. 2022;4(3):916-925. doi: 10.1039/D1NA00698C
- Noor N, Shapira A, Edri R, Gal I, Wertheim L, Dvir T. 3D printing of personalized thick and perfusable cardiac patches and hearts. Adv Sci. 2019;6(11):1900344. doi: 10.1002/advs.201900344
- Hong SY, Sun Y, Lee J, et al. 3D printing of free-standing Ti3C2Tx/PEO architecture for electromagnetic interference shielding. Polymer. 2021;236:124312. doi: 10.1016/j.polymer.2021.124312
- Yuan W, Liu H, Wang X, Huang L, Yin F, Yuan Y. Conductive MXene/melamine sponge combined with 3D printing resin base prepared as an electromagnetic interference shielding switch. Compos Part A Appl Sci Manuf. 2021;143:106238. doi: 10.1016/j.compositesa.2020.106238
- Basara G, Saeidi-Javash M, Ren X, et al. Electrically conductive 3D printed Ti3C2Tx MXene-PEG composite constructs for cardiac tissue engineering. Acta Biomater. 2020;139:179-189. doi: 10.1016/j.actbio.2020.12.033
- Wilkinson N, Smith M, Kay R, Harris RA. A review of aerosol jet printing—a non-traditional hybrid process for micro-manufacturing. Int J Adv Manuf Technol. 2019;105(11):4599-4619. doi: 10.1007/s00170-019-03438-2
- Filgueira D, Holmen S, Melbø JK, Moldes D, Echtermeyer AT, Chinga-Carrasco G. 3D printable filaments made of biobased polyethylene biocomposites. Polymers. 2018;10(3):314. doi: 10.3390/polym10030314
- Liu R, Chen Y, Cao J. Effects of modifier type on properties of in situ organo-montmorillonite modified wood flour/ poly (lactic acid) composites. ACS Appl Mater Interfaces. 2016;8(1):161-168. doi: 10.1021/acsami.5b07989
- Yang Z, Bi H, Bi Y, Rodrigue D, Xu M, Feng X. Comparison between polyethylene glycol and tributyl citrate to modify the properties of wood fiber/polylactic acid biocomposites. Polym Compos. 2019;40(4):1384-1394. doi: 10.1002/pc.24872
- Vaidya AA, Collet C, Gaugler M, Lloyd-Jones G. Integrating softwood biorefinery lignin into polyhydroxybutyrate composites and application in 3D printing. Mater Today Commun. 2019;19:286-296. doi: 10.1016/j.mtcomm.2019.02.008
- Zhao X, Tekinalp H, Meng X, et al. Poplar as biofiber reinforcement in composites for large-scale 3D printing. ACS Appl Bio Mater. 2019;2(10):4557-4570. doi: 10.1021/acsabm.9b00675
- Liu H, He H, Peng X, Huang B, Li J. Three,He H, Peng X,021/ acsabm.9b00675omposites for large-scale 3D printing. and application in 3D printing. c acid biocomposites. . lastic matrix. ation. lPolym Adv Technol. 2019;30(4):910-922. doi: 10.1002/pat.4524
- Jang J, Yi H-G, Cho D-W. 3D printed tissue models: present and future. ACS Biomater Sci Eng. 2016;2(10):1722-1731. doi: 10.1021/acsbiomaterials.6b00129
- Mohan TS, Datta P, Nesaei S, Ozbolat V, Ozbolat IT. 3D coaxial bioprinting: process mechanisms, bioinks and applications. Prog Biomed Eng. 2022;4(2):022003. doi: 10.1088/2516-1091/ac631c
- Li W, Hu X, Yang S, et al. A novel tissue-engineered 3D tumor model for anti-cancer drug discovery. Biofabrication. 2018;11(1):015004. doi: 10.1088/1758-5090/aae270
- Bhise NS, Ribas J, Manoharan V, et al. Organ-on-a-chip platforms for studying drug delivery systems. J Control Release. 2014;190:82-93. doi: 10.1016/j.jconrel.2014.05.004
- Murphy SV, De Coppi P, Atala A. Opportunities and challenges of translational 3D bioprinting. Nat Biomed Eng. 2020;4(4):370-380. doi: 10.1038/s41551-019-0471-7
- Yi B, Xu Q, Liu W. An overview of substrate stiffness guided cellular response and its applications in tissue regeneration. Bioact Mater. 2022;15:82-102. doi: 10.1016/j.bioactmat.2021.12.005
- Xia H, Chen Q, Fang Y, et al. Directed neurite growth of rat dorsal root ganglion neurons and increased colocalization with Schwann cells on aligned poly(methyl methacrylate) electrospun nanofibers. Brain Res. 2014;1565:18–27. doi: 10.1016/j.brainres.2014.04.002
- Lu K, Qian Y, Gong J, et al. Biofabrication of aligned structures that guide cell orientation and applications in tissue engineering. Bio-Des Manuf. 2021;4:258-277. doi: 10.1007/s42242-020-00104-5
- Shin YM, Yang HS, Chun HJ. Directional cell migration guide for improved tissue regeneration. Adv Exp Med Biol. 2020;1249:131-140. doi: 10.1007/978-981-15-3258-0_9
- Ballester-Beltran J, Biggs M, Dalby MJ, Salmerón-Sánchez M, Leal-Egaña A. Sensing the differences: the influence of anisotropic cues on cell behavior. Front Mater. 2015;2:39. doi: 10.3389/fmats.2015.00039
- Zhuo Y, Choi JS, Martin T, Yu H, Harley BA, Cunningham BT. Quantitative analysis of focal adhesion dynamics using photonic resonator outcoupler microscopy (PROM). Light Sci Appl. 2018;7(1):9. doi: 10.1038/s41377-018-0001-5
- Bowers DT, Brown JL. Nanofibers as bioinstructive scaffolds capable of modulating differentiation through mechanosensitive pathways for regenerative engineering. Regen Eng Transl Med. 2019;5:22-29. doi: 10.1007/s40883-018-0076-9
- Wang S, Ameli A, Shaayegan V, et al. Modelling of rod-like fillers’ rotation and translation near two growing cells in conductive polymer composite foam processing. Polymers. 2018;10(3):261. doi: 10.3390/polym10030261
- Volpi M, Paradiso A, Costantini M, Świȩszkowski W. Hydrogel-based fiber biofabrication techniques for skeletal muscle tissue engineering. ACS Biomater Sci Eng. 2022;8(2):379-405. doi: 10.1021/acsbiomaterials.1c01145
- Kim WJ, Jang CH, Kim GH. A myoblast-laden collagen bioink with fully aligned Au nanowires for muscle-tissue regeneration. Nano Lett. 2019;19(12):8612-8620. doi: 10.1021/acs.nanolett.9b03182
- Pardo A, Bakht SM, Gomez-Florit M, et al. Magnetically-assisted 3D bioprinting of anisotropic tissue-mimetic constructs. Adv Funct Mater. 2022;32(50):2208940. doi: 10.1002/adfm.202208940
- Zhang S, Chen X, Shan M, et al. Convergence of 3D bioprinting and nanotechnology in tissue engineering scaffolds. Biomimetics. 2023;8(1):94. doi: 10.3390/biomimetics8010094
- Shin J, Lee Y, Li Z, Hu J, Park SS, Kim K. Optimized 3D bioprinting technology based on machine kearning: a review of recent trends and advances. Micromachines. 2022;13(3):363. doi: 10.3390/mi13030363
- Quan Z, Wu A, Keefe M, et al. Additive manufacturing of multi-directional preforms for composites: opportunities and challenges. Mater Today. 2015;18(9):503-512. doi: 10.1016/j.mattod.2015.05.001
- Rajabi M, McConnell M, Cabral J, Azam Ali M. Chitosan hydrogels in 3D printing for biomedical applications. Carbonhydr Polym. 2021;260:117768. doi: 10.1016/j.carbpol.2021.117768
- Xu W, Molino BZ, Cheng F, et al. On low-concentration inks formulated by nanocellulose assisted with gelatin methacrylate (GelMA) for 3D printing toward wound healing application. ACS Appl Mater Interfaces. 2019;11(9):8838-8848. doi: 10.1021/acsami.8b21268
- Wang X, Zhang X, Dai X, et al. Tumor-like lung cancer model based on 3D bioprinting. 3 Biotech. 2018;8(12):1-9. doi: 10.1007/s13205-018-1519-1
- Vijayavenkataraman S, Lu W, Fuh J. 3D bioprinting of skin: a state-of-the-art review on modelling, materials, and processes. Biofabrication. 2016;8(3):032001. doi: 10.1088/1758-5090/8/3/032001
- Cubo N, Garcia M, Del Canizo JF, Velasco D, Jorcano JL. 3D bioprinting of functional human skin: production and in vivo analysis. Biofabrication. 2016;9(1):015006. doi: 10.1088/1758-5090/9/1/015006
- Kim BS, Gao G, Kim JY, Cho D-W. 3D cell printing of perfusable vascularized human skin equivalent composed of epidermis, dermis, and hypodermis for better structural recapitulation of native skin. Adv Healthc Mater. 2019;8(7):1801019. doi: 10.1002/adhm.201801019
- Pei H, Jing J, Chen Y, Guo J, Chen N. 3D printing of PVDF-based piezoelectric nanogenerator from programmable metamaterial design: Promising strategy for flexible electronic skin. Nano Energy. 2023;109:108303. doi: 10.1016/j.nanoen.2023.108303
- Lackner F, Knechtl I, Novak M, et al. 3D-printed anisotropic nanofiber composites with Gradual mechanical properties. Adv Mater Technol. 2023;8(10):2201708. doi: 10.1002/admt.202201708
- Markstedt K, Mantas A, Tournier I, Ávila HM, Hägg D, Gatenholm P. 3D bioprinting human chondrocytes with nanocellulose–alginate bioink for cartilage tissue engineering applications. Biomacromolecules. 2015;16(5):1489-1496. doi: 10.1021/acs.biomac.5b00188
- Rathan S, Dejob L, Schipani R, Haffner B, Möbius ME, Kelly DJ. Fiber reinforced cartilage ECM functionalized bioinks for functional cartilage tissue engineering. Adv Healthc Mater. 2019;8(7):1801501. doi: 10.1002/adhm.201801501
- Acedo CA, de Vicente J, González GJ, Chocarro C. Bio-inspired hydrogel composed of hyaluronic acid and alginate as a potential bioink for 3D bioprinting of articular cartilage engineering constructs. Acta Biomater. 2020;106:114-123. doi: 10.1016/j.actbio.2020.01.046
- Schipani R, Scheurer S, Florentin R, Critchley SE, Kelly DJ. Reinforcing interpenetrating network hydrogels with 3D printed polymer networks to engineer cartilage mimetic composites. Biofabrication. 2020;12(3):035011. doi: 10.1088/1758-5090/ab8708
- Müller M, Öztürk E, Arlov Ø, Gatenholm P, Zenobi- Wong M. Alginate sulfate–nanocellulose bioinks for cartilage bioprinting applications. Ann Biomed Eng. 2017; 45:210-223. doi: 10.1007/s10439-016-1704-5
- Markstedt K, Mantas A, Tournier I, Ávila HM, Hägg D, Gatenholm P. 3D bioprinting human chondrocytes with nanocellulose−alginate bioink for cartilage tissue engineering applications. Biomacromolecules. 2015;16(5):1489-1496. doi: 10.1021/acs.biomac.5b00188
- Sun Y, Wu Q, Zhang Y, Dai K, Wei Y. 3D-bioprinted gradient-structured scaffold generates anisotropic cartilage with vascularization by pore-size-dependent activation of HIF1α/FAK signaling axis. Nanomedicine. 2021;37:102426. doi: 10.1016/j.nano.2021.102426
- Sun Y, You Y, Jiang W, Wang Bo, Wu Q, Dai K. 3D bioprinting dual-factor releasing and gradient-structured constructs ready to implant for anisotropic cartilage regeneration. Sci Adv. 2020;6(37):eaay1422. doi: 10.1126/sciadv.aay1422
- Idaszek J, Costantini M, Karlsen TA, et al. 3D bioprinting of hydrogel constructs with cell and material gradients for the regeneration of full-thickness chondral defect using a microfluidic printing head. Biofabrication. 2019;11(4): 044101. doi: 10.1088/1758-5090/ab2622
- Costantini M, Testa S, Mozetic P, et al. Microfluidic-enhanced 3D bioprinting of aligned myoblast-laden hydrogels leads to functionally organized myofibers in vitro and in vivo. Biomaterials. 2017;131:98-110. doi: 10.1016/j.biomaterials.2017.03.026
- Li X, Yuan Y, Liu L, et al. 3D printing of hydroxyapatite/ tricalcium phosphate scaffold with hierarchical porous structure for bone regeneration. Bio-Des Manuf. 2020;3(1):15-29. doi: 10.1007/s42242-019-00056-5
- Samavedi S, Whittington AR, Goldstein AS. Calcium phosphate ceramics in bone tissue engineering: a review of properties and their influence on cell behavior. Acta Biomater. 2013;9(9):8037-8045. doi: 10.1016/j.actbio.2013.06.014
- Nyberg E, Rindone A, Dorafshar A, Grayson WL. Comparison of 3D-printed poly-ɛ-caprolactone scaffolds functionalized with tricalcium phosphate, hydroxyapatite, bio-oss, or decellularized bone matrix. Tissue Eng Part A. 2017;23(11-12):503-514. doi: 10.1089/ten.TEA.2016.0418
- Byambaa B, Annabi N, Yue K, et al. Bioprinted osteogenic and vasculogenic patterns for engineering 3D bone tissue. Adv Healthc Mater. 2017;6(16):1700015. doi: 10.1002/adhm.201700015
- Chiesa I, De Maria C, Lapomarda A, et al. Endothelial cells support osteogenesis in an in vitro vascularized bone model developed by 3D bioprinting. Biofabrication. 2020;12(2):025013. doi: 10.1088/1758-5090/ab6a1d
- Roy A, Saxena V, Pandey LM. 3D printing for cardiovascular tissue engineering: a review. Mater Technol. 2018;33(6): 433-442. doi: 10.1080/10667857.2018.1456616
- Jin G, Li K. The electrically conductive scaffold as the skeleton of stem cell niche in regenerative medicine. Mater Sci Eng C. 2014;45:671-681. doi: 10.1016/j.msec.2014.06.004
- Borriello A, Guarino V, Schiavo L, Alvarez-Perez MA, Ambrosio L. Optimizing PANi doped electroactive substrates as patches for the regeneration of cardiac muscle. J Mater Sci Mater Med. 2011;22(4):1053-1062. doi: 10.1007/s10856-011-4259-x
- Dvir T, Timko BP, Brigham MD, et al. Nanowired three-dimensional cardiac patches. Nat Nano. 2011;6(11):720-725. doi: 10.1038/nnano.2011.160
- Liu W, Zhao L, Wang C, Zhou J. Conductive nanomaterials for cardiac tissues engineering. Eng Regener. 2020;1:88-94. doi: 10.1016/j.engreg.2020.09.001
- Ashtari K, Nazari H, Ko H, et al. Electrically conductive nanomaterials for cardiac tissue engineering. Adv Drug Deliv Rev. 2019;144:162-179. doi: 10.1016/j.addr.2019.06.001
- Lee J, Manoharan V, Cheung L, et al. Nanoparticle-based hybrid scaffolds for deciphering the role of multimodal cues in cardiac tissue engineering. ACS Nano. 2019;13(11):12525-12539. doi: 10.1021/acsnano.9b03050
- Zhu K, Shin SR, van Kempen T, et al. Gold nanocomposite bioink for printing 3D cardiac constructs. Adv Funct Mater. 2017;27(12):1605352. doi: 10.1002/adfm.201605352
- Tomasina C, Bodet T, Mota C, Moroni L, Camarero- Espinosa S. Bioprinting vasculature: materials, cells and emergent techniques. Materials. 2019;12(17):2701. doi: 10.3390/ma12172701
- Wu J, Hu C, Tang Z, Yu Q, Liu X, Chen H. Tissue-engineered vascular grafts: balance of the four major requirements. Colloid Interface Sci Commun. 2018;23:34-44.
doi: 10.1016/j.colcom.2018.01.005
- Moroni L, Burdick JA, Highley C, et al. Biofabrication strategies for 3D in vitro models and regenerative medicine. Nat Rev Mater. 2018;3(5):21-37. doi: 10.1038/s41578-018-0006-y
- Piterina AV, Cloonan AJ, Meaney CL, et al. ECM-based materials in cardiovascular applications: inherent healing potential and augmentation of native regenerative processes. Int J Mol Sci. 2009;10(10):4375-4417. doi: 10.3390/ijms10104375
- Heinrich MA, Liu W, Jimenez A, et al. 3D bioprinting: from benches to translational applications. Small. 2019;15(23):1970126. doi: 10.1002/smll.201805510
- Sasmal P, Datta P, Wu Y, Ozbolat IT. 3D bioprinting for modeling vasculature. Microphysiol Syst. 2018;2:4. doi: 10.21037/mps.2018.10.02
- Lee J, Kim G. Three-dimensional hierarchical nanofibrous collagen scaffold fabricated using fibrillated collagen and pluronic F-127 for regenerating bone tissue. ACS Appl Mater Interfaces. 2018;10(42):35801-35811. doi: 10.1021/acsami.8b14088
- Gao Q, Liu Z, Lin Z, et al. 3D bioprinting of vessel-like structures with multilevel fluidic channels. ACS Biomater Sci Eng. 2017;3(3):399-408. doi: 10.1021/acsbiomaterials.6b00643
- Gao G, Park JY, Kim BS, Jang J, Cho D-W. Coaxial cell printing of freestanding, perfusable, and functional in vitro vascular models for recapitulation of native vascular endothelium pathophysiology. Adv Healthc Mater. 2018;7(23):1801102. doi: 10.1002/adhm.201801102
- Dolati F, Yu Y, Zhang Y, De Jesus AM, Sander EA, Ozbolat IT. In vitro evaluation of carbon-nanotube-reinforced bioprintable vascular conduits. Nanotechnology. 2014;25(14):145101. doi: 10.1088/0957-4484/25/14/145101
- Zhang Y, Yu Y, Dolati F, Ozbolat IT. Effect of multiwall carbon nanotube reinforcement on coaxially extruded cellular vascular conduits. Mater Sci Eng C. 2014;39:126-133. doi: 10.1016/j.msec.2014.02.036
- Shao L, Gao Q, Xie C, Fu J, Xiang M, He Y. Directly coaxial 3D bioprinting of large-scale vascularized tissue constructs. Biofabrication. 2020;12(3):035014. doi: 10.1088/1758-5090/ab7e76
- Li S, Liu Y, Li Y, Liu C, Sun Y, Hu Q. A novel method for fabricating engineered structures with branched micro-channel using hollow hydrogel fibers. Biomicrofluidics. 2016;10(6):064104. doi: 10.1063/1.4967456
- Wu Y, Chee AJ, Golzar H, Yu ACH, Tang XS. Embedded 3D printing of ultrasound-compatible arterial phantoms with biomimetic elasticity. Adv Funct Mater. 2022;32:2110153. doi: 10.1002/adfm.202110153
- Wu Y, Lin ZYW, Wenger AC, Tam KC, Tang XS. 3D bioprinting of liver-mimetic construct with alginate/ cellulose nanocrystal hybrid bioink. Bioprinting. 2018;9:1-6. doi: 10.1016/j.bprint.2017.12.001
- Wu Y, Wenger A, Golzar H, Tang XS. 3D bioprinting of bicellular liver lobule-mimetic structures via microextrusion of cellulose nanocrystal-incorporated shear-thinning bioink. Sci Rep. 2020;10(1):1-12. doi: 10.1038/s41598-020-77146-3
- Nguyen DG, Funk J, Robbins JB, et al. Bioprinted 3D primary liver tissues allow assessment of organ-level response to clinical drug induced toxicity in vitro. PLoS One. 2016;11(7):e0158674. doi: 10.1371/journal.pone.0158674
- Zhuang P, An J, Chua CK, Tan LP. Bioprinting of 3D in vitro skeletal muscle models: a review. Mater Des. 2020;193:108794. doi: 10.1016/j.matdes.2020.108794
- Fan T, Wang S, Jiang Z, et al. Controllable assembly of skeletal muscle-like bundles through 3D bioprinting. Biofabrication. 2021;14(1):015009. doi: 10.1088/1758-5090/ac3aca
- Choi YJ, Kim TG, Jeong J, et al., 2016, 3D cell printing of functional skeletal muscle constructs using skeletal muscle-derived bioink. Adv Healthc Mater. 2016;5(20): 2636-2645. doi: 10.1002/adhm.201600483
- Ma X, Wang M, Ran Y, et al. Design and fabrication of polymeric hydrogel carrier for nerve repair. Polymers. 2022;14(8):1549. doi: 10.3390/polym14081549
- Lozano R, Stevens L, Thompson BC, et al. 3D printing of layered brain-like structures using peptide modified gellan gum substrates. Biomaterials. 2015;67:264-273. doi: 10.1016/j.biomaterials.2015.07.022
- Yu X, Zhang T, Li Y. 3D printing and bioprinting nerve conduits for neural tissue engineering. Polymers. 2020;12(8):1637. doi: 10.3390/polym12081637
- Balasubramanian S, Yu K, Meyer AS, Karana E, Aubin- Tam M-E. Bioprinting of regenerative photosynthetic living materials. Adv Func Mater. 2021;31(31):2011162. doi: 10.1002/adfm.202011162
- Zhang Y, Hsu H-H, Wheeler JJ, Tanga S, Jiang X. Emerging investigator series: emerging biotechnologies in wastewater treatment: from biomolecular engineering to multiscale integration. Environ Sci: Water Res Technol. 2020;6(8): 1967-1985. doi: 10.1039/D0EW00393J
- Hsu L, Jiang X. ‘Living’ inks for 3D bioprinting. Trends Biotechnol. 2019;37(8):795-796. doi: 10.1016/j.tibtech.2019.04.014
- Liu J, Chen Y, Jiang S, et al. Rapid removal of Cr (III) from high-salinity wastewater by cellulose-g-poly-(acrylamide-co-sulfonic acid) polymeric bio-adsorbent. Carbohydr Polym. 2021;270:118356. doi: 10.1016/j.carbpol.2021.118356
- Gao X, Guo C, Hao J, Zhao Z, Long H, Li M. Adsorption of heavy metal ions by sodium alginate based adsorbent-a review and new perspectives. Int J Biol Macromol. 2020;164:4423-4434. doi: 10.1016/j.ijbiomac.2020.09.046
- Yusoff NHM, Teo L-RI, Phang SJ, Wong V-L, Cheah KH, Lim S-S. Recent advances in polymer-based 3D printing for wastewater treatment application: an overview. Chem Eng J. 2022;429:132311. doi: 10.1016/j.cej.2021.132311
- Qian F, Zhu C, Knipe JM, et al. Direct writing of tunable living inks for bioprocess intensification. Nano Lett. 2019;19(9):5829-5835. doi: 10.1021/acs.nanolett.9b00066
- Seidel J, Ahlfeld T, Adolph M, et al. Green bioprinting: extrusion-based fabrication of plant cell-laden biopolymer hydrogel scaffolds. Biofabrication. 2017;9(4): 045011. doi: 10.1088/1758-5090/aa8854
- Schaffner M, Rühs PA, Coulter F, Kilcher S, Studart AR. 3D printing of bacteria into functional complex materials. Sci Adv. 2017;3(12):eaao6804. doi: 10.1126/sciadv.aao6804
- Liu J, Shen X, Zheng Z, et al. Immobilization of laccase by 3D bioprinting and its application in the biodegradation of phenolic compounds. Int J Biol Macromol. 2020;164: 518-525. doi: 10.1016/j.ijbiomac.2020.07.144
- Deng P, Sheng W, Xu A, et al. Bottom-up construction of electrochemically active living filters: from graphene oxide mediated formation of bacterial cables to 3D assembly of hierarchical architectures. ACS Appl Mater Interfaces. 2020;3(11):7376-7381. doi: 10.1021/acsabm.0c01172