Chondrocyte spheroid-laden microporous hydrogel-based 3D bioprinting for cartilage regeneration
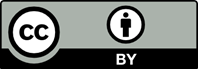
Three-dimensional (3D) bioprinting has brought new promising strategies for the regeneration of cartilage with specific shapes. In cartilage bioprinting, chondrocyte-laden hydrogels are the most commonly used bioinks. However, the dispersion of cells and the dense texture of the hydrogel in the conventional bioink may limit cell–cell/ cell–extracellular matrix (ECM) interactions, counting against cartilage regeneration and maturation. To address this issue, in this study, we developed a functional bioink for cartilage bioprinting based on chondrocyte spheroids (CSs) and microporous hydrogels, in which CSs as multicellular aggregates can provide extensive cell– cell/cell–ECM interactions to mimic the natural cartilage microenvironment, and microporous hydrogels can provide space and channel for the growth and fusion of the CSs. Firstly, we used a non-adhesive microporous system to produce homogeneous self-assembled CSs in high-throughput and evaluated the influence of different CSs preparation parameters (cell number and culture time) on CSs, which aids in the preparation of bioink suitable for cartilage bioprinting. Then, polyethylene oxide (PEO) was introduced into gelatin methacrylate (GelMA) to prepare microporous hydrogel. Finally, the CS-laden microporous hydrogels were printed, and the constructs were implanted into nude mice. The results showed that the CSs with 500 cells cultured for 1 day exhibited better proliferation and growth ability in microporous hydrogels compared to those with more cells and cultured for longer time. In addition, the results also demonstrated that the CS-laden bioink can be successfully printed into predefined lattice-shape constructs with little cell damage and regenerated cartilage tissue in vivo with a structure similar to natural cartilage characterized by typical lacunae structure and abundant cartilage-specific ECM deposition. In summary, our study verified the feasibility and advantages of using CSs as building blocks in cartilage bioprinting, which provides novel strategies for the fabrication and regeneration of patient-specific shaped cartilage.
- Jessop ZM, Hague A, Dobbs TD, Stewart KJ, Whitaker IS. Facial cartilaginous reconstruction-A historical perspective, state-of-the-art, and future directions. Front Surg. 2021;8:680186. doi: 10.3389/fsurg.2021.680186
- Cao Y, Sang S, An Y, Xiang C, Li Y, Zhen Y. Progress of 3D printing techniques for nasal cartilage regeneration. Aesthetic Plast Surg. 2022;46(2):947–964. doi: 10.1007/s00266-021-02472-4
- Cao Y, Vacanti JP, Paige KT, Upton J, Vacanti CA. Transplantation of chondrocytes utilizing a polymer-cell construct to produce tissue-engineered cartilage in the shape of a human ear. Plast Reconstr Surg. 1997,100(2): 297–302; discussion 303–294. doi: 10.1097/00006534-199708000-00001
- Tan B, Gan S, Wang X, Liu W, Li Xiaoming. Applications of 3D bioprinting in tissue engineering: Advantages, deficiencies, improvements, and future perspectives. J Mater Chem B. 2021;9(27):5385–5413. doi: 10.1039/d1tb00172h
- Wang G, Zhang X, Bu X, Yang An, Bi Hongsen, Zhao Z. The application of cartilage tissue engineering with cell-laden hydrogel in plastic surgery: A systematic review. Tissue Eng Regen Med. 2022;19(1):1–9. doi: 10.1007/s13770-021-00394-5
- Tang P, Song P, Peng Z, et al. Chondrocyte-laden GelMA hydrogel combined with 3D printed PLA scaffolds for auricle regeneration. Mater Sci Eng C Mater Biol Appl. 2021;130(1):112423. doi: 10.1016/j.msec.2021.112423
- Zeng J, Jia L, Wang D, et al. Bacterial nanocellulose-reinforced gelatin methacryloyl hydrogel enhances biomechanical property and glycosaminoglycan content of 3D-bioprinted cartilage. Int J Bioprint. 2023;9(1):631. doi: 10.18063/ijb.v9i1.631
- Achilli TM, Meyer J, Morgan JR. Advances in the formation, use and understanding of multi-cellular spheroids. Expert Opin Biol Ther. 2012;12(10):1347–1360. doi: 10.1517/14712598.2012.707181
- Kronemberger GS, Matsui RAM, Miranda G, Granjeiro JM, Baptista LS. Cartilage and bone tissue engineering using adipose stromal/stem cells spheroids as building blocks. World J Stem Cells. 2020;12(2):110–122. doi: 10.4252/wjsc.v12.i2.110
- Zhang J, Xin W, Qin Y, et al. “All-in-one” zwitterionic granular hydrogel bioink for stem cell spheroids production and 3D bioprinting. Chem Eng J. 2022;430(50):132713. doi: 10.1016/j.cej.2021.132713
- Chen H, Tan XN, Hu S, et al. Molecular mechanisms of chondrocyte proliferation and differentiation. Front Cell Dev Biol. 2021;9:664168.doi: 10.3389/fcell.2021.664168
- Ghosh S, Laha M, Mondal S, Sengupta S, Kaplana DL. In vitro model of mesenchymal condensation during chondrogenic development. Biomaterials. 2009;30(33): 6530–6540. doi: 10.1016/j.biomaterials.2009.08.019
- Chae S, Hong J, Hwangbo H, Kim G. The utility of biomedical scaffolds laden with spheroids in various tissue engineering applications. Theranostics. 2021;11(14): 6818–6832. doi: 10.7150/thno.58421
- Decarli MC, Amaral R, Santos DPD, et al. Cell spheroids as a versatile research platform: formation mechanisms, high throughput production, characterization and applications. Biofabrication. 2021;13(3):1-37. doi: 10.1088/1758-5090/abe6f2
- Mironov V, Visconti RP, Kasyanov V, Gabor Forgacs, Drake CJ, Markwald RR. Organ printing: Tissue spheroids as building blocks. Biomaterials. 2009;30(12):2164–2174. doi: 10.1016/j.biomaterials.2008.12.084
- Grevenstein D, Mamilos A, Schmitt VH, et al. Excellent histological results in terms of articular cartilage regeneration after spheroid-based autologous chondrocyte implantation (ACI). Knee Surg Sports Traumatol Arthrosc. 2021;29(2):417–421. doi: 10.1007/s00167-020-05976-9
- Gryadunova A, Kasamkattil J, Gay MHP, et al. Nose to spine: Spheroids generated by human nasal chondrocytes for scaffold-free nucleus pulposus augmentation. Acta Biomater. 2021;134(4):240–251. doi: 10.1016/j.actbio.2021.07.064
- Huang BJ, Hu JC, Athanasiou KA. Effects of passage number and post-expansion aggregate culture on tissue engineered, self-assembled neocartilage. Acta Biomater. 2016;43(3): 150–159. doi: 10.1016/j.actbio.2016.07.044
- Jeon JH, Yun BG, Lim MJ, et al. Rapid cartilage regeneration of spheroids composed of human nasal septum-derived chondrocyte in rat osteochondral defect model. Tissue Eng Regen Med. 2020;17(1):81–90. doi: 10.1007/s13770-019-00231-w
- Wang G, An Y, Zhang X, Pengbing Ding, Bi H, Zhao Z. Chondrocyte spheroids laden in GelMA/HAMA hybrid hydrogel for tissue-engineered cartilage with enhanced proliferation, better phenotype maintenance, and natural morphological structure. Gels. 2021;7(4):247. doi: 10.3390/gels7040247
- De Moor L, Minne M, Tytgat L, et al. Tuning the phenotype of cartilage tissue mimics by varying spheroid maturation and methacrylamide-modified gelatin hydrogel characteristics. Macromol Biosci. 2021;21(5):e2000401. doi: 10.1002/mabi.202000401
- Kang HW, Lee SJ, Ko IK, Yoo JJ, Atala A. A 3D bioprinting system to produce human-scale tissue constructs with structural integrity. Nat Biotechnol. 2016;34(3):312–319. doi: 10.1038/nbt.3413
- Ying GL, Jiang N, Maharjan S, et al. Aqueous two-phase emulsion bioink-enabled 3D bioprinting of porous hydrogels. Adv Mater. 2018;30(50):e1805460. doi: 10.1002/adma.201805460
- Ying G, Jiang N, Parra C, et al. Bioprinted injectable hierarchically porous gelatin methacryloyl hydrogel constructs with shape-memory properties. Adv Funct Mater. 2020;30(46). doi: 10.1002/adfm.202003740
- Shi W, Shi H, Fu G, et al. Application of agarose gelatin in tissue pre-embedding before dehydration. J Clin Exp Pathol. 2021;37(3):365–366. doi: 10.13315/j.cnki.cjcep.2021.03.031
- Jia L, Hua Y, Zeng J, et al. Bioprinting and regeneration of auricular cartilage using a bioactive bioink based on microporous photocrosslinkable acellular cartilage matrix. Bioact Mater. 2022;16(1):66–81. doi: 10.1016/j.bioactmat.2022.02.032
- Van Winkle AP, Gates ID, Kallos MS. Mass transfer limitations in embryoid bodies during human embryonic stem cell differentiation. Cells Tissues Organs. 2012;196(1):34–47. doi: 10.1159/000330691
- Lin RZ, Chang HY. Recent advances in three-dimensional multicellular spheroid culture for biomedical research. Biotechnol J. 2008;3(9–10):1172–1184. doi: 10.1002/biot.200700228
- Shi W, Kwon J, Huang Y, et al. Facile tumor spheroids formation in large quantity with controllable size and high uniformity. Sci Rep. 2018;8(1):6837. doi: 10.1038/s41598-018-25203-3
- Niebruegge S, Bauwens CL, Peerani R, et al. Generation of human embryonic stem cell-derived mesoderm and cardiac cells using size-specified aggregates in an oxygen-controlled bioreactor. Biotechnol Bioeng. 2009;102(2):493–507. doi: 10.1002/bit.22065
- Sart S, Tsai AC, Li Y, et al. Three-dimensional aggregates of mesenchymal stem cells: Cellular mechanisms, biological properties, and applications. Tissue Eng Part B Rev. 2014;20(5):365–380. doi: 10.1089/ten.TEB.2013.0537
- Behan K, Dufour A, Garcia O, Kelly D. Methacrylated cartilage ECM-based hydrogels as injectables and bioinks for cartilage tissue engineering. Biomolecules. 2022;12(2):216. doi: 10.3390/biom12020216
- 33. Ozbolat IT, Hospodiuk M. Current advances and future perspectives in extrusion-based bioprinting. Biomaterials. 2016;76:321–343. doi: 10.1016/j.biomaterials.2015.10.076