Nanoclay-reinforced alginate/salecan composite inks for 3D printing applications
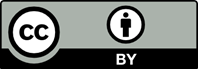
The main objective of the present work was to produce three-dimensional (3D)- printable nanocomposite hydrogels based on two kinds of marine-sourced polysaccharides doped with nanoclay with potential biomedical application. First part of the research study investigated the preparation of the polysaccharide bicomponent hydrogel formulations followed by the selection of the optimal ratio of polysaccharides concentrations which ensured proper morphostructural stability of the 3D-printed constructs. Second step aimed to generate 3D scaffolds with high printing fidelity by modulating the nanoclay amount doped within the previously selected biopolymer ink. In compliance with the additive manufacturing experiments, the alginate–salecan hydrogels enriched with the highest nanofiller concentrations demonstrated the highest suitability for 3D printing process. The morphological and structural studies confirmed the ability of the nanocomposite formulations to efficiently produce porous 3D-printed constructs with improved fidelity. The morphostructural findings underlined the implication of choosing the appropriate ratio between components, as they have a considerable impact on the functionality of printing formulations and subsequent 3D-printed structures. Hence, from the obtained results, these novel hydrogel nanocomposites inks are considered valuable biomaterials with suitable features for applications in the additive manufacturing of 3D structures with precise shape for customized regenerative therapy.
- Mohammed ASA, Naveed M, Jost N. Polysaccharides; classification, chemical properties, and future perspective applications in fields of pharmacology and biological medicine (a review of current applications and upcoming potentialities). J Polym Environ. 2021;29(8):2359-2371. doi: 10.1007/s10924-021-02052-2
- Zhang X, Kim G, Kang M, et al. Marine biomaterial-based bioinks for generating 3D printed tissue constructs. Marine Drugs. 2018;16(12):484. doi: 10.3390/md16120484
- Sharma A, Kaur I, Dheer D, et al. A propitious role of marine sourced polysaccharides: Drug delivery and biomedical applications. Carbohydr Polym. 2023;308:120448. doi: 10.1016/j.carbpol.2022.120448
- Aderibigbe B, Buyana B. Alginate in wound dressings. Pharmaceutics. 2018;10(2):42. doi: 10.3390/pharmaceutics10020042
- Ahmad Raus R, Wan Nawawi WMF, Nasaruddin RR. Alginate and alginate composites for biomedical applications. Asian J Pharm Sci. 2021;16(3):280-306. doi: 10.1016/j.ajps.2020.10.001
- Datta S, Barua R, Das J. Importance of alginate bioink for 3D bioprinting in tissue engineering and regenerative medicine, in Alginates - Recent Uses of This Natural Polymer, IntechOpen, UK. 2020. doi: 10.5772/intechopen.90426
- Axpe E, Oyen M. Applications of alginate-based bioinks in 3D bioprinting. IJMS. 2016;17(12):1976. doi: 10.3390/ijms17121976
- Mallakpour S, Azadi E, Hussain CM. State-of-the-art of 3D printing technology of alginate-based hydrogels—An emerging technique for industrial applications. Adv Colloid Interface Sci. 2021;293:102436. doi: 10.1016/j.cis.2021.102436
- Hazur J, Detsch R, Karakaya E, et al. Improving alginate printability for biofabrication: Establishment of a universal and homogeneous pre-crosslinking technique. Biofabrication. 2020;12(4):045004. doi: 10.1088/1758-5090/ab98e5
- Falcone G, Mazzei P, Piccolo A, et al. Advanced printable hydrogels from pre-crosslinked alginate as a new tool in semi solid extrusion 3D printing process. Carbohydr Polym. 2022;276:118746. doi: 10.1016/j.carbpol.2021.118746
- Piras CC, Smith DK. Multicomponent polysaccharide alginate-based bioinks. J Mater Chem B. 2020;8(36):8171-8188. doi: 10.1039/D0TB01005G
- Distler T, Solisito AA, Schneidereit D, Friedrich O, Detsch R, Boccaccini AR. 3D printed oxidized alginate-gelatin bioink provides guidance for C2C12 muscle precursor cell orientation and differentiation via shear stress during bioprinting. Biofabrication. 2020;12(4):045005. doi: 10.1088/1758-5090/ab98e4
- Amr M, Dykes I, Counts M, et al. 3D printed, mechanically tunable, composite sodium alginate, gelatin and Gum Arabic (SA-GEL-GA) scaffolds. Bioprinting. 2021;22:e00133. doi: 10.1016/j.bprint.2021.e00133
- Alruwaili M, Lopez JA, McCarthy K, Reynaud EG, Rodriguez BJ. Liquid-phase 3D bioprinting of gelatin alginate hydrogels: Influence of printing parameters on hydrogel line width and layer height. Bio-des Manuf. 2019;2(3):172-180. doi: 10.1007/s42242-019-00043-w
- Huang J, Fu H, Wang Z, et al. BMSCs-laden gelatin/ sodium alginate/carboxymethyl chitosan hydrogel for 3D bioprinting. RSC Adv. 2016;6(110):108423-108430. doi: 10.1039/C6RA24231F
- Sadeghianmaryan A, Naghieh S, Yazdanpanah Z, et al. Fabrication of chitosan/alginate/hydroxyapatite hybrid scaffolds using 3D printing and impregnating techniques for potential cartilage regeneration. Int J Biol Macromol. 2022;204:62-75. doi: 10.1016/j.ijbiomac.2022.01.201
- Li H, Tan YJ, Leong KF, Li L. 3D bioprinting of highly thixotropic alginate/methylcellulose hydrogel with strong interface bonding. ACS Appl Mater Interfaces. 2017;9(23):20086-20097. doi: 10.1021/acsami.7b04216
- Kanafi NM, Rahman NA, Rosdi NH. Citric acid cross-linking of highly porous carboxymethyl cellulose/poly(ethylene oxide) composite hydrogel films for controlled release applications. Mater Today: Proc. 2019;7(Part 2):721-731. doi: 10.1016/j.matpr.2018.12.067
- Aljohani W, Ullah MW, Li W, Shi L, Zhang X, Yang G. Three-dimensional printing of alginate-gelatin-agar scaffolds using free-form motor assisted microsyringe extrusion system. J Polym Res. 2018;25(3):62. doi: 10.1007/s10965-018-1455-0
- Wang J, Liu Y, Zhang X, et al. 3D printed agar/ calcium alginate hydrogels with high shape fidelity and tailorable mechanical properties. Polymer. 2021;214:123238. doi: 10.1016/j.polymer.2020.123238
- Bednarzig V, Schrüfer S, Schneider TC, Schubert DW, Detsch R, Boccaccini AR. Improved 3D printing and cell biology characterization of inorganic-filler containing alginate-based composites for bone regeneration: Particle shape and effective surface area are the dominant factors for printing performance. Int J Mol Sci. 2022;23(9):4750. doi: 10.3390/ijms23094750
- Bider F, Karakaya E, Mohn D, Boccaccini AR. Advantages of nanoscale bioactive glass as inorganic filler in alginate hydrogels for drug delivery and biofabrication. EJMS. 2022;2(1):33-53. doi: 10.1080/26889277.2022.2039078
- Shahbazi M, Jäger H, Ahmadi SJ, Lacroix M. Electron beam crosslinking of alginate/nanoclay ink to improve functional properties of 3D printed hydrogel for removing heavy metal ions. Carbohydr Polym. 2020;240:116211. doi: 10.1016/j.carbpol.2020.116211
- Ahlfeld T, Cidonio G, Kilian D, et al. Development of a clay based bioink for 3D cell printing for skeletal application. Biofabrication. 2017;9(3):034103. doi: 10.1088/1758-5090/aa7e96
- Alexa RL, Iovu H, Trica B, et al. Assessment of naturally sourced mineral clays for the 3D printing of biopolymer-based nanocomposite inks. Nanomaterials. 2021;11(3):703. doi: 10.3390/nano11030703
- Cidonio G, Glinka M, Kim YH, et al. Nanoclay-based 3D printed scaffolds promote vascular ingrowth ex vivo and generate bone mineral tissue in vitro and in vivo. Biofabrication. 2020;12(3):035010. doi: 10.1088/1758-5090/ab8753
- Qi X, Wei W, Shen J, Dong W. Salecan polysaccharide-based hydrogels and their applications: A review. J Mater Chem B. 2019;7(16):2577-2587. doi: 10.1039/C8TB03312A
- Fu R, Li J, Zhang T, et al. Salecan stabilizes the microstructure and improves the rheological performance of yogurt. Food Hydrocoll. 2018;81:474-480. doi: 10.1016/j.foodhyd.2018.03.034
- Fan Z, Cheng P, Gao Y, et al. Understanding the rheological properties of a novel composite salecan/gellan hydrogels, Food Hydrocolloids. 2022;123(2):107162 doi: 10.1016/j.foodhyd.2021.107162
- Zhang Q, Ren T, Gan J, et al. Synthesis and rheological characterization of a novel salecan hydrogel. Pharmaceutics; 2022;14(7):1492. doi: 10.3390/pharmaceutics14071492
- Fan Z, Cheng P, Yin G, Wang Z, Han J. In situ forming oxidized salecan/gelatin injectable hydrogels for vancomycin delivery and 3D cell culture. J Biomater Sci. 2020;31(6):762-780. doi: 10.1080/09205063.2020.1717739
- Gan J, Sun L, Guan C, et al. Preparation and properties of salecan-soy protein isolate composite hydrogel induced by thermal treatment and transglutaminase. Int J Mol Sci. 2022;23(16):9383. doi: 10.3390/ijms23169383
- Qi X, Su T, Tong X, et al. Facile formation of salecan/agarose hydrogels with tunable structural properties for cell culture. Carbohydr Polym. 2019;224:115208. doi: 10.1016/j.carbpol.2019.115208
- Hu X, Wang Y, Zhang L, Xu M, Dong W, Zhang J. Redox/pH dual stimuli-responsive degradable Salecan-g-SS-poly(IA-co-HEMA) hydrogel for release of doxorubicin. Carbohydr Polym. 2017;155:242-251. doi: 10.1016/j.carbpol.2016.08.077
- Qi X, Wei W, Li J, et al. Design of salecan-containing semi- IPN hydrogel for amoxicillin delivery. Mater Sci Eng C. 2017;75:487-494. doi: 10.1016/j.msec.2017.02.089
- Wei W, Hu X, Qi X, et al. A novel thermo-responsive hydrogel based on salecan and poly(N-isopropylacrylamide): Synthesis and characterization. Colloids Surf B: Biointerfaces. 2015;125:1-11. doi: 10.1016/j.colsurfb.2014.10.057
- Munteanu T, Ninciuleanu CM, Gifu IC, et al. The effect of clay type on the physicochemical properties of new hydrogel clay nanocomposites, IntechOpen, UK. 2018. doi: 10.5772/intechopen.74478
- Florian PE, Icriverzi M, Ninciuleanu CM, et al. Salecan-clay based polymer nanocomposites for chemotherapeutic drug delivery systems; characterization and in vitro biocompatibility studies. Materials. 2020;13(23):5389. doi: 10.3390/ma13235389
- Ianchis R, Alexa RL, Gifu IC, et al. Novel green crosslinked salecan hydrogels and preliminary investigation of their use in 3D printing. Pharmaceutics. 2023;15(2):373. doi: 10.3390/pharmaceutics15020373
- Ianchis R, Ninciuleanu C, Gifu IC, et al. Hydrogel-clay nanocomposites as carriers for controlled release. CMC. 2018;25(6):919-954. doi: 10.2174/0929867325666180831151055
- Jafarbeglou M, Abdouss M, Shoushtari AM, Jafarbeglou M. Clay nanocomposites as engineered drug delivery systems. RSC Adv. 2016;6(55):50002-50016. doi: 10.1039/C6RA03942A
- Marin MM, Ianchis R, Leu Alexa R, et al. Development of new collagen/clay composite biomaterials. Int J Mol Sci. 2022;23(1):401. doi: 10.3390/ijms23010401
- Gaharwar AK, Cross LM, Peak CW, et al. 2D nanoclay for biomedical applications: regenerative medicine, therapeutic delivery, and additive manufacturing. Adv Mater. 2019;31(23):1900332. doi: 10.1002/adma.201900332
- Ninciuleanu CM, Ianchiş R, Alexandrescu E, et al. The effects of monomer, crosslinking agent, and filler concentrations on the viscoelastic and swelling properties of poly(methacrylic acid) hydrogels: A comparison. Materials (Basel). 2021;14(9):2305. doi: 10.3390/ma14092305
- Fialová L, Capek I, Ianchis R, Corobea MC, Donescu D, Berek D. Kinetics of styrene and butyl acrylate polymerization in anionic microemulsions in presence of layered silicates. Polym J. 2008;40(2):163-170. doi: 10.1295/polymj.PJ2007160
- Fan D, Li Y, Wang X, et al. Progressive 3D printing technology and its application in medical materials. Front Pharmacol. 2020;11:122. doi: 10.3389/fphar.2020.00122
- Ghilan A, Chiriac AP, Nita LE, Rusu AG, Neamtu I, Chiriac VM. Trends in 3D printing processes for biomedical field: Opportunities and challenges. J Polym Environ. 2020;28(5):1345-1367. doi: 10.1007/s10924-020-01722-x
- Jiang T, Munguia-Lopez JG, Flores-Torres S, Kort-Mascort J, Kinsella JM. Extrusion bioprinting of soft materials: An emerging technique for biological model fabrication. Appl Phys Rev. 2019;6(1):011310. doi: 10.1063/1.5059393
- Jiang Z, Diggle B, Tan ML, Viktorova J, Bennett CW, Connal LA. Extrusion 3D printing of polymeric materials with advanced properties. Adv Sci. 2020;7(17):2001379. doi: 10.1002/advs.202001379
- Joas S, Tovar G, Celik O, Bonten C, Southan A. Extrusion-based 3D printing of poly(ethylene glycol) diacrylate hydrogels containing positively and negatively charged groups. Gels. 2018;4(3):69. doi: 10.3390/gels4030069
- Suntornnond R, Ng WL, Huang X, Yeow CHE, Yeong WY. Improving printability of hydrogel-based bio-inks for thermal inkjet bioprinting applications via saponification and heat treatment processes. J Mater Chem B. 2022;10(31):5989-6000. doi: 10.1039/D2TB00442A
- Ng WL, Lee JM, Zhou M, et al. Vat polymerization-based bioprinting—process, materials, applications and regulatory challenges. Biofabrication. 2020;12(2):022001. doi: 10.1088/1758-5090/ab6034
- Coppola B, Cappetti N, Di Maio L, Scarfato P, Incarnato L. 3D printing of PLA/clay nanocomposites: Influence of printing temperature on printed samples properties. Materials. 2018;11(10):1947. doi: 10.3390/ma11101947
- Kianfar F, Dempster N, Gaskell E, Hutcheon MR and GA. Lyophilised biopolymer-clay hydrogels for drug delivery. MJNDR. 2017;1(1):1-9. doi: 10.18689/mjndr-1000101
- Chaudhuri SD, Dey A, Upganlawar S, Chakrabarty D. Influence of clay concentration on the absorption and rheological attributes of modified cellulose /acrylic acid based hydrogel and the application of such hydrogel. Mater Chem Phys. 2022;282:125942. doi: 10.1016/j.matchemphys.2022.125942
- Kumar A, Won SY, Sood A, et al. Triple-networked hybrid hydrogels reinforced with montmorillonite clay and graphene nanoplatelets for soft and hard tissue regeneration. IJMS. 2022;23(22):14158. doi: 10.3390/ijms232214158
- Leu Alexa R, Ianchis R, Savu D, et al. 3D printing of alginate-natural clay hydrogel-based nanocomposites. Gels. 2021;7(4):211. doi: 10.3390/gels7040211
- Stloukal P, Pekařová S, Kalendova A, et al. Kinetics and mechanism of the biodegradation of PLA/clay nanocomposites during thermophilic phase of composting process. Waste Manag. 2015;42:31-40. doi: 10.1016/j.wasman.2015.04.006
- Pluta M, Paul MA, Alexandre M, Dubois P. Plasticized polylactide/clay nanocomposites. II. The effect of aging on structure and properties in relation to the filler content and the nature of its organo-modification. J Polym Sci B Polym Phys. 2006;44(2):312-325. doi: 10.1002/polb.20697
- Serafin A, Culebras M, Collins MN. Synthesis and evaluation of alginate, gelatin, and hyaluronic acid hybrid hydrogels for tissue engineering applications. Int J Biol Macromol. 2023;233:123438. doi: 10.1016/j.ijbiomac.2023.123438
- Serafin A, Murphy C, Rubio MC, Collins MN. Printable alginate/gelatin hydrogel reinforced with carbon nanofibers as electrically conductive scaffolds for tissue engineering. Mater Sci Eng C. 2021;122:111927. doi: 10.1016/j.msec.2021.111927
- Dávila JL, d’Ávila MA. Rheological evaluation of Laponite/ alginate inks for 3D extrusion-based printing. Int J Adv Manuf Technol. 2019;101(1-4):675-686. doi: 10.1007/s00170-018-2876-y
- Hickey RJ, Pelling AE. Cellulose biomaterials for tissue engineering. Front Bioeng Biotechnol. 2019;7:45. doi: 10.3389/fbioe.2019.00045
- Saveleva MS, Eftekhari K, Abalymov A, et al. Hierarchy of hybrid materials—The place of inorganics-in-organics in it, their composition and applications. Front Chem. 2019;7:179. doi: 10.3389/fchem.2019.00179
- Sachot N, Engel E, Castano O. Hybrid organic-inorganic scaffolding biomaterials for regenerative therapies. COC. 2014;18(18):2299-2314. doi: 10.2174/1385272819666140806200355
- Handorf AM, Zhou Y, Halanski MA, Li WJ. Tissue stiffness dictates development, homeostasis, and disease progression. Organogenesis. 2015;11(1):1-15. doi: 10.1080/15476278.2015.1019687
- Marin MM, Gifu IC, Pircalabioru GG, et al. Microbial polysaccharide-based formulation with silica nanoparticles; A new hydrogel nanocomposite for 3D printing. Gels. 2023;9(5):425. doi: 10.3390/gels9050425
- Hu X, Yan L, Wang Y, Xu M. Microwave-assisted synthesis of nutgall tannic acid–based salecan polysaccharide hydrogel for tunable release of β-lactoglobulin. Int J Biol Macromol. 2020;161:1431-1439. doi: 10.1016/j.ijbiomac.2020.07.250
- Hu X, Wang Y, Zhang L, Xu M, Zhang J, Dong W. Design of a pH-sensitive magnetic composite hydrogel based on salecan graft copolymer and Fe3O4@SiO2 nanoparticles as drug carrier. Int J Biol Macromol. 2018;107:1811-1820. doi: 10.1016/j.ijbiomac.2017.10.043
- Mollah MZI, Faruque MRI, Bradley DA, Khandaker MU, Assaf SA. FTIR and rheology study of alginate samples: Effect of radiation. Radiat Phys Chem. 2023;202:110500. doi: 10.1016/j.radphyschem.2022.110500
- Zheng H, Yang J, Han S. The synthesis and characteristics of sodium alginate/graphene oxide composite films crosslinked with multivalent cations. J Appl Polym Sci. 2016;133(27):43616. doi: 10.1002/app.43616
- Xinyu H, Linlin Y, Man X, Lihua T. Photo-degradable salecan/xanthan gum ionic gel induced by iron (III) coordination for organic dye decontamination. Int J Biol Macromol. 2023;238:124132. doi: 10.1016/j.ijbiomac.2023.124132
- Qi X, Su T, Zhang M, et al. Macroporous hydrogel scaffolds with tunable physicochemical properties for tissue engineering constructed using renewable polysaccharides. ACS Appl Mater Interfaces. 2020;12(11):13256-13264. doi: 10.1021/acsami.9b20794
- Fan Z, Cheng P, Wang D, Zhao Y, Wang Z, Han J. Design and investigation of salecan/chitosan hydrogel formulations with improved antibacterial performance and 3D cell culture function. J Biomater Sci. 2020;31(17):2268-2284. doi: 10.1080/09205063.2020.1800907
- Foudazi R, Zowada R, Manas-Zloczower I, Feke DL. Porous hydrogels: Present challenges and future opportunities. Langmuir. 2023;39(6):2092-2111. doi: 10.1021/acs.langmuir.2c02253
- 76. Lee KY, Mooney DJ. Alginate: Properties and biomedical applications. Prog Polym Sci. 2012;37(1):106-126. doi: 10.1016/j.progpolymsci.2011.06.003