Bioprinting technology for the management of diabetic foot disease: Emerging applications, challenges, and prospects
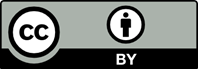
Most conventional therapies have limitations in the repair of complex wounds caused by chronic inflammation in patients with diabetic foot ulcers (DFUs). In response to the demand for more biotechnology strategies, bioprinting has been explored in the regeneration field in recent years. However, challenges remain regarding the structure of complex models and the selection of proper biomaterials. The purpose of this review is to introduce the current applications of bioprinting technology in chronic diabetic foot wound healing. First, the most common application of bioprinting in producing skin equivalents to promote wound healing is introduced; second, functional improvements in the treatment of chronic and difficult-to-heal DFU wounds facilitated by bioprinting applications are discussed; and last but not least, bioprinting applications in addressing unique diabetic foot disease characteristics are summarized. Furthermore, the present work summarizes material selection and correlations between three-dimensional (3D) bioprinting and a variety of biomimetic strategies for accelerating wound healing. Novel, biotechnological tools such as organoids for developing new biomaterials for bioprinting in the future are also discussed.
- Mota C, Camarero-Espinosa S, Baker MB, et al., 2020, Bioprinting: From tissue and organ development to in vitro models. Chem Rev, 120(19): 10547–10607.
- Valot L, Martinez J, Mehdi A, et al., 2019, Chemical insights into bioinks for 3D printing. Chem Soc Rev, 48(15): 4049– 4086.
- Xu HQ, Liu JC, Zhang ZY, et al., 2022, A review on cell damage, viability, and functionality during 3D bioprinting. Mil Med Res, 9(1): 70.
- GhavamiNejad A, Ashammakhi N, Wu XY, et al., 2020, Crosslinking strategies for 3D bioprinting of polymeric hydrogels. Small, 16(35): e2002931.
- Skeldon G, Lucendo-Villarin B, Shu W, 2018, Three-dimensional bioprinting of stem-cell derived tissues for human regenerative medicine. Philos Trans R Soc Lond B Biol Sci, 373(1750): 20170224.
- Ravanbakhsh H, Karamzadeh V, Bao G, et al., 2021, Emerging technologies in multi-material bioprinting. Adv Mater, 33(49): e2104730.
- Yan WC, Davoodi P, Vijayavenkataraman S, et al., 2018, 3D bioprinting of skin tissue: From pre-processing to final product evaluation. Adv Drug Deliv Rev, 132 (2018): 270–295.
- Las Heras K, Igartua M, Santos-Vizcaino E, et al., 2020, Chronic wounds: Current status, available strategies and emerging therapeutic solutions. J Control Release, 328 (2020): 532–550.
- Grennan D, 2019, Diabetic foot ulcers. JAMA, 321(1): 114.
- Schaper NC, van Netten JJ, Apelqvist J, et al, 2020, IWGDF Editorial Board. Practical Guidelines on the prevention and management of diabetic foot disease (IWGDF 2019 update). Diabetes Metab Res Rev, 36(Suppl 1): e3266.
- Abbas ZG, Boulton AJM, 2022, Diabetic foot ulcer disease in African continent: ‘From clinical care to implementation’ - Review of diabetic foot in last 60 years - 1960 to 2020. Diabetes Res Clin Pract, 183 (2022): 109155.
- Tan CT, Liang K, Ngo ZH, et al., 2020, Application of 3D bioprinting technologies to the management and treatment of diabetic foot ulcers. Biomedicines, 8(10): 441.
- Masri S, Zawani M, Zulkiflee I, et al., 2022, Cellular interaction of human skin cells towards natural bioink via 3D-bioprinting technologies for chronic wound: A comprehensive review. Int J Mol Sci, 23(1): 476.
- Glover K, Stratakos AC, Varadi A, et al., 2021, 3D scaffolds in the treatment of diabetic foot ulcers: New trends vs conventional approaches. Int J Pharm, 599(2021): 120423.
- Sun Y, Gao Y, Chen J, et al., 2019, Evidence mapping of recommendations on diagnosis and therapeutic strategies for diabetes foot: An international review of 22 guidelines. Metabolism, 100 (2019): 153956.
- Manita PG, Garcia-Orue I, Santos-Vizcaino E, et al., 2021, 3D bioprinting of functional skin substitutes: From current achievements to future goals. Pharmaceuticals (Basel), 14(4): 362.
- He P, Zhao J, Zhang J, et al., 2018, Bioprinting of skin constructs for wound healing. Burns Trauma, 6(2018): 5.
- Yan WC, Davoodi P, Vijayavenkataraman S, et al., 2018, 3D bioprinting of skin tissue: From pre-processing to final product evaluation. Adv Drug Deliv Rev, 132(2018): 270–295.
- Kim HS, Sun X, Lee JH, et al., 2019, Advanced drug delivery systems and artificial skin grafts for skin wound healing. Adv Drug Deliv Rev, 146(2019): 209–239.
- Chouhan D, Dey N, Bhardwaj N, et al., 2019, Emerging and innovative approaches for wound healing and skin regeneration: Current status and advances. Biomaterials, 216(2019): 119267.
- Jeong HJ, Nam H, Jang J, et al., 2020, 3D bioprinting strategies for the regeneration of functional tubular tissues and organs. Bioengineering (Basel), 7(2): 32.
- Yanez M, Rincon J, Dones A, et al., 2015, In vivo assessment of printed microvasculature in a bilayer skin graft to treat full-thickness wounds. Tissue Eng Part A, 21(1–2): 224–233.
- Datta P, Barui A, Wu Y, et al., 2018, Essential steps in bioprinting: From pre- to post-bioprinting. Biotechnol Adv, 36(5): 1481–1504.
- Assad H, Assad A, Kumar A, 2023, Recent developments in 3D bio-printing and its biomedical applications. Pharmaceutics, 15(1): 255.
- Huang C, Yuan W, Chen J, et al., 2023, Construction of smart biomaterials for promoting diabetic wound healing. Molecules, 28(3): 1110.
- Bittner SM, Guo JL, Melchiorri A, et al., 2018, Three-dimensional printing of multilayered tissue engineering scaffolds. Mater Today (Kidlington), 21(8): 861–874.
- Ng WL, Qi JTZ, Yeong WY, et al., 2018, Proof-of-concept: 3D bioprinting of pigmented human skin constructs. Biofabrication, 10(2): 025005.
- Lawlor KT, Vanslambrouck JM, Higgins JW, et al., 2021, Cellular extrusion bioprinting improves kidney organoid reproducibility and conformation. Nat Mater, 20(2): 260–271.
- LaBarge W, Morales A, Pretorius D, et al., 2019, Scaffold-free bioprinter utilizing layer-by-layer printing of cellular spheroids. Micromachines (Basel), 10(9): 570.
- Zhang Y, Enhejirigala, Yao B, et al., 2021, Using bioprinting and spheroid culture to create a skin model with sweat glands and hair follicles. Burns Trauma, 9(2021): tkab013.
- Samandari M, Mostafavi A, Quint J, et al., 2022, In situ bioprinting: Intraoperative implementation of regenerative medicine. Trends Biotechnol, 40(10): 1229–1247.
- Dong H, Hu B, Zhang W, et al., 2022, Robotic-assisted automated in situ bioprinting. Int J Bioprint, 9(1): 629.
- Cubo N, Garcia M, Del Cañizo JF, et al., 2016, 3D bioprinting of functional human skin: Production and in vivo analysis. Biofabrication, 9(1): 015006.
- Tan SH, Ngo ZH, Sci DB, et al., 2022, Recent advances in the design of three-dimensional and bioprinted scaffolds for full-thickness wound healing. Tissue Eng Part B Rev, 28(1): 160–181.
- Gungor-Ozkerim PS, Inci I, Zhang YS, et al., 2018, Bioinks for 3D bioprinting: An overview. Biomater Sci, 6(5): 915–946.
- Wang H, Yu H, Zhou X, et al., 2022, An overview of extracellular matrix-based bioinks for 3D bioprinting. Front Bioeng Biotechnol, 10(2022): 905438.
- Tao G, Wang Y, Cai R, et al., 2019, Design and performance of sericin/poly(vinyl alcohol) hydrogel as a drug delivery carrier for potential wound dressing application. Mater Sci Eng C Mater Biol Appl, 101(2019): 341–351.
- Chen CS, Zeng F, Xiao X, et al., 2018, Three-dimensionally printed silk-sericin-based hydrogel scaffold: A promising visualized dressing material for real-time monitoring of wounds. ACS Appl Mater Interfaces, 10(40): 33879–33890.
- Gonzalez-Fernandez T, Tenorio AJ, Campbell KT, et al., 2021, Alginate-based bioinks for 3D bioprinting and fabrication of anatomically accurate bone grafts. Tissue Eng Part A, 27(17–18): 1168–1181.
- Lee SC, Gillispie G, Prim P, et al., 2020, Physical and chemical factors influencing the printability of hydrogel-based extrusion bioinks. Chem Rev, 120(19): 10834–10886.
- Jin SG, 2022, Production and application of biomaterials based on polyvinyl alcohol (PVA) as wound dressing. Chem Asian J, 17(21): e202200595.
- Baltazar T, Merola J, Catarino C, et al., 2020, Three dimensional bioprinting of a vascularized and perfusable skin graft using human keratinocytes, fibroblasts, pericytes, and endothelial cells. Tissue Eng Part A, 26(5–6): 227–238.
- Pontiggia L, Van Hengel IA, Klar A, et al., 2022, Bioprinting and plastic compression of large pigmented and vascularized human dermo-epidermal skin substitutes by means of a new robotic platform. J Tissue Eng, 13(2022): 20417314221088513.
- Skylar-Scott MA, Huang JY, Lu A, et al., 2022, Orthogonally induced differentiation of stem cells for the programmatic patterning of vascularized organoids and bioprinted tissues. Nat Biomed Eng, 6(4): 449–462. https://doi.org/10.1038/s41551-022-00856-8. Epub 2022 Mar 24. PMID: 35332307; PMCID: PMC9506705.
- Abaci HE, Guo Z, Coffman A, et al., 2016, Human skin constructs with spatially controlled vasculature using primary and iPSC-derived endothelial cells. Adv Healthc Mater, 5(14): 1800–1807.
- Tasoglu S, Demirci U, 2013, Bioprinting for stem cell research. Trends Biotechnol, 31(1): 10–19.
- Ker ED, Nain AS, Weiss LE, et al., 2011, Bioprinting of growth factors onto aligned sub-micron fibrous scaffolds for simultaneous control of cell differentiation and alignment. Biomaterials, 32(32): 8097–8107.
- Elkhoury K, Chen M, Koçak P, et al., 2022, Hybrid extracellular vesicles-liposome incorporated advanced bioink to deliver microRNA. Biofabrication, 14(4). https://doi.org/10.1088/1758-5090/ac8621.
- Abaci A, Guvendiren M, 2020, Designing decellularized extracellular matrix-based bioinks for 3D bioprinting. Adv Healthc Mater, 9(24): e2000734.
- Choudhury D, Tun HW, Wang T, et al., 2018, Organ-derived decellularized extracellular matrix: A game changer for bioink manufacturing? Trends Biotechnol, 36(8): 787–805.
- Chen J, Zhou D, Nie Z, et al., 2021, A scalable coaxial bioprinting technology for mesenchymal stem cell microfiber fabrication and high extracellular vesicle yield. Biofabrication, 14(1).
- Wu J, Piao Y, Liu Q, et al., 2021, Platelet-rich plasma-derived extracellular vesicles: A superior alternative in regenerative medicine? Cell Prolif, 54(12): e13123. https://doi.org/10.1111/cpr.13123. Epub 2021 Oct 5. PMID: 34609779; PMCID: PMC8666280.
- Cui H, Zhu W, Holmes B, et al., 2016, Biologically inspired smart release system based on 3D bioprinted perfused scaffold for vascularized tissue regeneration. Adv Sci (Weinh), 3(8): 1600058. https://doi.org/10.1002/advs.201600058. PMID: 27818910; PMCID: PMC5074245.
- Zhao W, Chen H, Zhang Y, et al., 2022, Adaptive multi-degree-of-freedom in situ bioprinting robot for hair-follicle-inclusive skin repair: A preliminary study conducted in mice. Bioeng Transl Med, 7(3): e10303.
- Jorgensen AM, Varkey M, Gorkun A, et al., 2020, Bioprinted skin recapitulates normal collagen remodeling in full-thickness wounds. Tissue Eng Part A, 26(9–10): 512–526. https://doi.org/10.1089/ten.TEA.2019.0319. Epub 2020 Jan 28. PMID: 31861970; PMCID: PMC7249461.
- Maver T, Mohan T, Gradišnik L, et al., 2019, Polysaccharide thin solid films for analgesic drug delivery and growth of human skin cells. Front Chem, 7: 217.
- Jahanshahi M, Hamdi D, Godau B, et al., 2020, An engineered infected epidermis model for in vitro study of the skin’s pro-inflammatory response. Micromachines (Basel), 11(2): 227.
- Cui C, Kim DO, Pack MY, et al., 2020, 4D printing of self-folding and cell-encapsulating 3D microstructures as scaffolds for tissue-engineering applications. Biofabrication, 12(4): 045018.
- Spoerk M, Arbeiter F, Raguž I, et al., 2019, Mechanical recyclability of polypropylene composites produced by material extrusion-based additive manufacturing. Polymers (Basel), 11(8): 1318.
- Xu HQ, Liu JC, Zhang ZY, et al., 2022, A review on cell damage, viability, and functionality during 3D bioprinting. Mil Med Res, 9(1): 70. printed scaffolds for full-thickness wound healing. Tissue Eng Part B Rev, 28(1): 160–181.
- Guillemot F, Mironov V, Nakamura M, 2010, Bioprinting is coming of age: Report from the International Conference on Bioprinting and Biofabrication in Bordeaux (3B’09). Biofabrication, 2(1): 010201.
- Mu R, Campos de Souza S, Liao Z, et al., 2022, Reprograming the immune niche for skin tissue regeneration - From cellular mechanisms to biomaterials applications. Adv Drug Deliv Rev, 185(2022): 114298.
- Las Heras K, Igartua M, Santos-Vizcaino E, et al., 2020, Chronic wounds: Current status, available strategies and emerging therapeutic solutions. J Control Release, 328(2020): 532–550.
- Kolimi P, Narala S, Nyavanandi D, et al., 2022, Innovative treatment strategies to accelerate wound healing: Trajectory and recent advancements. Cells, 11(15): 2439.
- Martin P, Nunan R, 2015, Cellular and molecular mechanisms of repair in acute and chronic wound healing. Br J Dermatol, 173(2): 370–378.
- Felgueiras HP, 2023, Emerging antimicrobial and immunomodulatory fiber-based scaffolding systems for treating diabetic foot ulcers. Pharmaceutics, 15(1): 258.
- Eming SA, Martin P, Tomic-Canic M, 2014, Wound repair and regeneration: mechanisms, signaling, and translation. Sci Transl Med, 6(265): 265sr6.
- Gravel SP, Ben Khalifa Y, McGuirk S, et al., 2023, PGC-1s shape epidermal physiology by modulating keratinocyte proliferation and terminal differentiation. iScience, 26(4): 106314.
- Chouhan D, Dey N, Bhardwaj N, et al., 2019, Emerging and innovative approaches for wound healing and skin regeneration: Current status and advances. Biomaterials, 216(2019): 119267.
- Huang F, Lu X, Yang Y, et al., 2023, Microenvironment-based diabetic foot ulcer nanomedicine. Adv Sci (Weinh), 10(2): e2203308.
- Theocharidis G, Baltzis D, Roustit M, et al., 2020, Integrated skin transcriptomics and serum multiplex assays reveal novel mechanisms of wound healing in diabetic foot ulcers. Diabetes, 69(10): 2157–2169.
- Aitcheson SM, Frentiu FD, Hurn SE, et al., 2021, Skin wound healing: Normal macrophage function and macrophage dysfunction in diabetic wounds. Molecules, 26(16): 4917.
- Zhang J, Yang P, Liu D, et al., 2021, Inhibiting hyper-O-GlcNAcylation of c-Myc accelerate diabetic wound healing by alleviating keratinocyte dysfunction. Burns Trauma, 9(2021): tkab031.
- Qi W, Yang C, Dai Z, et al., 2015, High levels of pigment epithelium-derived factor in diabetes impair wound healing through suppression of Wnt signaling. Diabetes, 64(4): 1407–1419.
- Su D, Tsai HI, Xu Z, et al., 2019, Exosomal PD-L1 functions as an immunosuppressant to promote wound healing. J Extracell Vesicles, 9(1): 1709262.
- Sinha S, Sparks HD, Labit E, et al., 2022, Fibroblast inflammatory priming determines regenerative versus fibrotic skin repair in reindeer. Cell, 185(25): 4717–4736.e25.
- Pichu S, Vimalraj S, Sathiyamoorthy J, et al., 2018, Association of hypoxia inducible factor-1 alpha exon 12 mutation in diabetic patients with and without diabetic foot ulcer. Int J Biol Macromol, 119(2018): 833–837.
- Dhamodharan U, Karan A, Sireesh D, et al., 2019, Tissue-specific role of Nrf2 in the treatment of diabetic foot ulcers during hyperbaric oxygen therapy. Free Radic Biol Med, 138(2019): 53–62.
- Theocharidis G, Thomas BE, Sarkar D, et al., 2022, Single cell transcriptomic landscape of diabetic foot ulcers. Nat Commun, 13(1): 181.
- Chen J, Qin S, Liu S, et al., 2023, Targeting matrix metalloproteases in diabetic wound healing. Front Immunol, 14(2023): 1089001.
- Lobmann R, Ambrosch A, Schultz G, et al., 2002, Expression of matrix-metalloproteinases and their inhibitors in the wounds of diabetic and non-diabetic patients. Diabetologia, 45(7): 1011–1016.
- Li N, Zhan A, Jiang Y, et al., 2022, A novel matrix metalloproteinases-cleavable hydrogel loading deferoxamine accelerates diabetic wound healing. Int J Biol Macromol, 222(Pt A): 1551–1559.
- Yu FX, Lee PSY, Yang L, et al., 2022, The impact of sensory neuropathy and inflammation on epithelial wound healing in diabetic corneas. Prog Retin Eye Res, 89(2022): 101039.
- Srivastava P, Sondak T, Sivashanmugam K, et al., 2022, A review of immunomodulatory reprogramming by probiotics in combating chronic and acute diabetic foot ulcers (DFUs). Pharmaceutics, 14(11): 2436.
- Xiao Y, Zhao H, Ma X, et al., 2022, Hydrogel dressing containing basic fibroblast growth factor accelerating chronic wound healing in aged mouse model. Molecules, 27(19): 6361.
- Huang X, Liang P, Jiang B, et al., 2020, Hyperbaric oxygen potentiates diabetic wound healing by promoting fibroblast cell proliferation and endothelial cell angiogenesis. Life Sci, 259(2020): 118246.
- Sharma R, Sharma SK, Mudgal SK, et al., 2021, Efficacy of hyperbaric oxygen therapy for diabetic foot ulcer, a systematic review and meta-analysis of controlled clinical trials. Sci Rep, 11(1): 2189.
- Guo JL, Longaker MT, 2022, Bioprinted hydrogels for fibrosis and wound healing: Treatment and modeling. Gels, 9(1): 19.
- Nessbach P, Schwarz S, Becke TD, et al., 2022, Angiogenic potential of co-cultured human umbilical vein endothelial cells and adipose stromal cells in customizable 3D engineered collagen sheets. J Funct Biomater, 13(3): 107.
- Li Y, Xu T, Tu Z, et al., 2020, Bioactive antibacterial silica-based nanocomposites hydrogel scaffolds with high angiogenesis for promoting diabetic wound healing and skin repair. Theranostics, 10(11): 4929–4943.
- Prasad YS, Miryala S, Lalitha K, et al., 2020, An injectable self-healing anesthetic glycolipid-based oleogel with antibiofilm and diabetic wound skin repair properties. Sci Rep, 10(1): 18017.
- Xia S, Weng T, Jin R, et al., 2022, Curcumin-incorporated 3D bioprinting gelatin methacryloyl hydrogel reduces reactive oxygen species-induced adipose-derived stem cell apoptosis and improves implanting survival in diabetic wounds. Burns Trauma, 10(2022): tkac001.
- Ullah F, Javed F, Mushtaq I, et al., 2023, Development of highly-reproducible hydrogel based bioink for regeneration of skin-tissues via 3-D bioprinting technology. Int J Biol Macromol, 230: 123131.
- Ma J, Qin C, Wu J, et al., 2021, 3D printing of strontium silicate microcylinder-containing multicellular biomaterial inks for vascularized skin regeneration. Adv Healthc Mater, 10(16): e2100523.
- Wang X, Yang C, Yu Y, et al., 2022, In situ 3D bioprinting living photosynthetic scaffolds for autotrophic wound healing. Research (Wash D C), 2022(2022): 9794745.
- Guan G, Qizhuang Lv, Liu S, et al., 2021, 3D-bioprinted peptide coupling patches for wound healing. Mater Today Bio, 13(2021): 100188.
- Liao W, Duan X, Xie F, et al., 2023, 3D-bioprinted double-crosslinked angiogenic alginate/chondroitin sulfate patch for diabetic wound healing. Int J Biol Macromol, 236(2023): 123952.
- Jo Y, Hwang DG, Kim M, et al., 2023, Bioprinting-assisted tissue assembly to generate organ substitutes at scale. Trends Biotechnol, 41(1): 93–105.
- Albanna M, Binder KW, Murphy SV, et al., In situ bioprinting of autologous skin cells accelerates wound healing of extensive excisional full-thickness wounds. Sci Rep, 9(1): 1856.
- Wu Y, Fortunato GM, Okesola BO, et al., 2021, An interfacial self-assembling bioink for the manufacturing of capillary-like structures with tuneable and anisotropic permeability. Biofabrication, 13(3).
- Jia W, Gungor-Ozkerim PS, Zhang YS, et al., 2016, Direct 3D bioprinting of perfusable vascular constructs using a blend bioink. Biomaterials, 106(2016): 58–68.
- Fang Y, Guo Y, Wu B, et al., 2023, Expanding embedded 3D bioprinting capability for engineering complex organs with freeform vascular networks. Adv Mater, 35(22): e2205082.
- Skylar-Scott MA, Uzel SGM, Nam LL, et al., 2019, Biomanufacturing of organ-specific tissues with high cellular density and embedded vascular channels. Sci Adv, 5(9): eaaw2459.
- Wu SD, Dai NT, Liao CY, et al., 2022, Planar-/curvilinear-bioprinted tri-cell-laden hydrogel for healing irregular chronic wounds. Adv Healthc Mater, 11(16): e2201021.
- Pagan E, Stefanek E, Seyfoori A, et al., 2023, A handheld bioprinter for multi-material printing of complex constructs. Biofabrication, 15(3): 035012.
- Altunbek M, Afghah F, Caliskan OS, et al., 2023, Design and bioprinting for tissue interfaces. Biofabrication, 15(2).
- Monteiro-Soares M, Russell D, Boyko EJ, et al., 2020, Guidelines on the classification of diabetic foot ulcers (IWGDF 2019). Diabetes Metab Res Rev, 36(Suppl 1): e3273.
- Huang SM, Wu CS, Chiu MH, et al., 2019, High glucose environment induces M1 macrophage polarization that impairs keratinocyte migration via TNF-α: An important mechanism to delay the diabetic wound healing. J Dermatol Sci, 96(3): 159–167.
- Tian F, Jiang Y, Liu Y, et al., 2021, Can fractal dimension analysis be used in quantitating collagen structure? Exp Dermatol, 30(12): 1825–1828.
- Xu H, Zhang J, Jiang Y, et al., 2022, Fractal analysis of rat dermal tissue in the different injury states. Int Wound J, 19(5): 1016–1022.
- Pena G, Kuang B, Szpak Z, et al., 2020, Evaluation of a novel three-dimensional wound measurement device for assessment of diabetic foot ulcers. Adv Wound Care (New Rochelle), 9(11): 623–631.
- Parsa S, Wamsley C, Kim P, et al., 2022, Use of optical coherence tomography (OCT) in assessment of diabetic skin wound characteristics and blood flow. J Foot Ankle Surg, 62(3): 407–412.
- Li C, Chen J, Jie T, et al., 2022, Construction of biomimetic tissues with anisotropic structures via stepwise algorithm-assisted bioprinting. Small, 18(46): e2204316.
- Li C, Chen J, Jie T, et al., 2022, Construction of biomimetic tissues with anisotropic structures via stepwise algorithm-assisted bioprinting. Small, 18(46): e2204316.
- Gholami P, Ahmadi-Pajouh MA, Abolftahi N, et al., 2018, Segmentation and measurement of chronic wounds for bioprinting. IEEE J Biomed Health Inform, 22(4): 1269–1277.
- Zahia S, Garcia-Zapirain B, Elmaghraby A, 2020, Integrating 3D model representation for an accurate non-invasive assessment of pressure injuries with deep learning. Sensors (Basel), 20(10): 2933.
- Zhao R, Tang H, Xu C, et al., 2021, Automatic quantitative analysis of structure parameters in the growth cycle of artificial skin using optical coherence tomography. J Biomed Opt, 26(9): 095001.
- Wang M, Li W, Mille LS, et al., 2022, Digital light processing based bioprinting with composable gradients. Adv Mater, 34(1): e2107038.
- Nuutila K, Samandari M, Endo Y, et al., 2021, In vivo printing of growth factor-eluting adhesive scaffolds improves wound healing. Bioact Mater, 8(2021): 296–308.
- Biranje SS, Sun J, Cheng L, et al., 2022, Development of cellulose nanofibril/casein-based 3D composite hemostasis scaffold for potential wound-healing application. ACS Appl Mater Interfaces, 14(3): 3792–3808.
- Phang SJ, Arumugam B, Kuppusamy UR, et al., 2021, A review of diabetic wound models-Novel insights into diabetic foot ulcer. J Tissue Eng Regen Med, 15(12): 1051– 1068.
- Kim BS, Ahn M, Cho WW, et al., 2021, Engineering of diseased human skin equivalent using 3D cell printing for representing pathophysiological hallmarks of type 2 diabetes in vitro. Biomaterials, 272(2021): 120776.
- Lan CC, Wu CS, Huang SM, et al., 2012, High-glucose environment reduces human β-defensin-2 expression in human keratinocytes: Implications for poor diabetic wound healing. Br J Dermatol, 166(6): 1221–1229.
- Intini C, Elviri L, Cabral J, et al., 2018, 3D-printed chitosan-based scaffolds: An in vitro study of human skin cell growth and an in-vivo wound healing evaluation in experimental diabetes in rats. Carbohydr Polym, 199(2018): 593–602.
- Wu M, Zhang Y, Huang H, et al., 2020, Assisted 3D printing of microneedle patches for minimally invasive glucose control in diabetes. Mater Sci Eng C Mater Biol Appl, 117(2020): 111299.
- Economidou SN, Pere CPP, Reid A, et al., 2019, 3D printed microneedle patches using stereolithography (SLA) for intradermal insulin delivery. Mater Sci Eng C Mater Biol Appl, 102(2019): 743–755.
- Kamel R, El-Batanony R, Salama A, 2019, Pioglitazone-loaded three-dimensional composite polymeric scaffolds: A proof of concept study in wounded diabetic rats. Int J Pharm, 570(2019): 118667.
- Chen TY, Wen TK, Dai NT, et al., 2021, Cryogel/hydrogel biomaterials and acupuncture combined to promote diabetic skin wound healing through immunomodulation. Biomaterials, 269(2021): 120608.
- Crews RT, Candela J, 2018, Decreasing an offloading device’s size and offsetting its imposed limb-length discrepancy lead to improved comfort and gait. Diabetes Care, 41(7): 1400–1405.
- Potier L, François M, Dardari D, et al., 2020, Comparison of a new versus standard removable offloading device in patients with neuropathic diabetic foot ulcers: A French national, multicentre, open-label randomized, controlled trial. BMJ Open Diabetes Res Care, 8(1): e000954.
- Zhang X, Wang H, Du C, et al., 2022, Custom-molded offloading footwear effectively prevents recurrence and amputation, and lowers mortality rates in high-risk diabetic foot patients: A multicenter, prospective observational study. Diabetes Metab Syndr Obes, 15(2022): 103–109.
- Zhao Y, Zhong J, Wang Y, et al., 2023, Photocurable and elastic polyurethane based on polyether glycol with adjustable hardness for 3D printing customized flatfoot orthosis. Biomater Sci, 11(5): 1692–1703.
- Choi H, Seo A, Lee J, 2019, Mallet finger lattice casts using 3D printing. J Healthc Eng, 2019(2019): 4765043.
- Gelain F, Luo Z, Zhang S, 2020, Self-assembling peptide EAK16 and RADA16 nanofiber scaffold hydrogel. Chem Rev, 120(24): 13434–13460.
- Cao W, Peng S, Yao Y, et al., 2022, A nanofibrous membrane loaded with doxycycline and printed with conductive hydrogel strips promotes diabetic wound healing in vivo. Acta Biomater, 152(2022): 60–73.
- Manso GMDC, Elias-Oliveira J, Guimarães JB, et al., 2023, Xenogeneic mesenchymal stem cell biocurative improves skin wounds healing in diabetic mice by increasing mast cells and the regenerative profile. Regen Ther, 22(2023): 79–89.
137. Dong Y, Rodrigues M, Kwon SH, et al., 2018, Acceleration of diabetic wound regeneration using an in situ-formed stem-cell-based skin substitute. Adv Healthc Mater, 7(17): e1800432.
- Yastı AÇ, Akgun AE, Surel AA, et al., 2023, Graft of 3D bioprinted autologous minimally manipulated homologous adipose tissue for the treatment of diabetic foot ulcer. Wounds, 35(1): E22–E28.
- Bajuri MY, Kim J, Yu Y, et al., 2023, New paradigm in diabetic foot ulcer grafting techniques using 3D-bioprinted autologous minimally manipulated homologous adipose tissue (3D-AMHAT) with fibrin gel acting as a biodegradable scaffold. Gels, 9(1): 66.
- Kesavan R, Sheela Sasikumar C, Narayanamurthy VB, et al., 2021, Management of diabetic foot ulcer with MA-ECM (minimally manipulated autologous extracellular matrix) using 3D bioprinting technology - An innovative approach. Int J Low Extrem Wounds, 0 (0): 15347346211045625. doi:10.1177/15347346211045625
- Armstrong DG, Harris SG, Rasor Z, et al., 2022, Autologous minimally manipulated homologous adipose tissue (AMHAT) for treatment of nonhealing diabetic foot ulcers. Plast Reconstr Surg Glob Open, 10(10): e4588.
- Bernal PN, Bouwmeester M, Madrid-Wolff J, et al., 2022, Volumetric bioprinting of organoids and optically tuned hydrogels to build liver-like metabolic biofactories. Adv Mater, 34(15): e2110054.
- Zhao M, Wang J, Zhang J, et al., 2022, Functionalizing multi-component bioink with platelet-rich plasma for customized in-situ bilayer bioprinting for wound healing. Mater Today Bio, 16(2023): 100334.
- Huang Q, Wu T, Guo Y, et al., 2023, Platelet-rich plasma-loaded bioactive chitosan@sodium alginate@gelatin shell-core fibrous hydrogels with enhanced sustained release of growth factors for diabetic foot ulcer healing. Int J Biol Macromol, 234(2023): 123722.