In-plane measurements and computational fluid dynamics prediction of permeability for biocompatible NiTi gyroid scaffolds fabricated via laser powder bed fusion
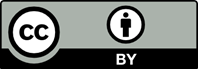
Laser powder bed fusion (LPBF) is considered a promising technology for manufacturing porous, biomimetic, and patient-specific scaffolds for bone repair. Scaffold permeability is one of the key factors to be considered for acquiring the required mass-transport properties in bone tissue engineering. This study aims to reveal the relationship between the design parameters of gyroid-based porous structure and scaffold permeability. A set of gyroid samples was manufactured from intermetallic NiTi alloy. Nine configurations of porous structures were obtained by varying the main design parameters, namely wall thickness and unit cell size. The in-plane method was employed to measure the permeability coefficient for the gyroid structures. Computational fluid dynamics simulations of the porous structures were performed to predict the targeted properties in an implant at the design stage before LPBF manufacturing. The results of the simulations were validated with the obtained experimental results. Geometrical accuracy and surface morphology of the as-built samples were investigated with various techniques. Biocompatibility assessment of the gyroid scaffolds was performed with human cell culture experiments.
- Mower TM, Long MJ. Mechanical behavior of additive manufactured, powder-bed laser-fused materials. Mater Sci Eng A. 2016;651: 198-213. doi: 10.1016/j.msea.2015.10.068
- Yuan L, Ding S, Wen C. Additive manufacturing technology for porous metal implant applications and triple minimal surface structures: A review. Bioact Mater. 2019;4: 56-70. doi: 10.1016/J.BIOACTMAT.2018.12.003
- Qin Y, Wen P, Guo H, et al. Additive manufacturing of biodegradable metals: Current research status and future perspectives. Acta Biomater. 2019;98: 3-22. doi: 10.1016/j.actbio.2019.04.046
- Yan C, Hao L, Hussein A, Young P. Ti-6Al-4V triply periodic minimal surface structures for bone implants fabricated via selective laser melting. J Mech Behav Biomed Mater. 2015;51: 61-73. doi: 10.1016/j.jmbbm.2015.06.024
- Elahinia MH, Hashemi M, Tabesh M, Bhaduri SB. Manufacturing and processing of NiTi implants: A review. Prog Mater Sci. 2012;57: 911-946. doi: 10.1016/j.pmatsci.2011.11.001
- Shishkovsky IV, Volova LT, Kuznetsov MV, Morozovc YG, Parkin IP. Porous biocompatible implants and tissue scaffolds synthesized by selective laser sintering from Ti and NiTi. J Mater Chem. 2008;18: 1309. doi: 10.1039/b715313a
- Hameed P, Liu CF, Ummethala R, Singh N. Biomorphic porous Ti6Al4V gyroid scaffolds for bone implant applications fabricated by selective laser melting. Prog Addit Manuf. 2021;6: 455-469. doi: 10.1007/s40964-021-00210-5
- Montazerian H, Davoodi E, Asadi-Eydivand M, Kadkhodapour J, Solati-Hashjin M. Porous scaffold internal architecture design based on minimal surfaces: A compromise between permeability and elastic properties. Mater Des. 2017;126: 98-114. doi: 10.1016/j.matdes.2017.04.009
- Ma S, Tang Q, Han X, et al. Manufacturability, mechanical properties, mass-transport properties and biocompatibility of triply periodic minimal surface (TPMS) porous scaffolds fabricated by selective laser melting. Mater Des. 2020;195: 109034. doi: 10.1016/j.matdes.2020.109034
- Ali D, Ozalp M, Blanquer SBG, Onel S. Permeability and fluid flow-induced wall shear stress in bone scaffolds with TPMS and lattice architectures: A CFD analysis. Eur J Mech B/Fluids. 2020;79: 376-385. doi: 10.1016/j.euromechflu.2019.09.015
- Pires T, Dunlop JWC, Fernandes PR, Castro APG. Computational fluid dynamics simulation of TPMS scaffolds for bone tissue engineering. Proc R Soc A Math Phys Eng Sci. 2022;478. doi: 10.1098/rspa.2021.0607
- Jalali M, Mohammadi K, Movahhedy MR, et al. SLM additive manufacturing of NiTi porous implants: A review of constitutive models, finite element simulations, manufacturing, heat treatment, mechanical, and biomedical studies. Metals Mater Int. 2023;2023: 1-34. doi: 10.1007/s12540-023-01401-1
- Schoen AH. Infinite periodic minimal surfaces without self-intersections. NASA TN D-5541. 1970.
- Jinnai H, Nishikawa Y, Ito M, Agard DA, Spontak RJ. Topological similarity of sponge-like bicontinuous morphologies differing in length scale. Adv Mater. 2002;14: 1615-1618. doi: 10.1002/1521-4095(20021118)14:22<1615::AID-ADMA1615>3.0.CO;2-S
- Beaudoin AJ, Mihalko WM, Krause WR. Finite element modelling of polymethylmethacrylate flow through cancellous bone. J Biomech. 1991;24: 127-136. doi: 10.1016/0021-9290(91)90357-S
- Nauman EA, Fong KE, Keaveny TM. Dependence of intertrabecular permeability on flow direction and anatomic site. Ann Biomed Eng. 1999;27: 517-524. doi: 10.1114/1.195
- Ali D, Sen S. Finite element analysis of mechanical behavior, permeability and fluid induced wall shear stress of high porosity scaffolds with gyroid and lattice-based architectures. J Mech Behav Biomed Mater. 2017;75: 262-270. doi: 10.1016/j.jmbbm.2017.07.035
- Ma S, Tang Q, Feng Q, Song J, Han X, Guo F. Mechanical behaviours and mass transport properties of bone-mimicking scaffolds consisted of gyroid structures manufactured using selective laser melting. J Mech Behav Biomed Mater. 2019;93: 158-169. doi: 10.1016/j.jmbbm.2019.01.023
- Shishkovsky I, Morozov Y, Smurov I. Nanofractal surface structure under laser sintering of titanium and nitinol for bone tissue engineering. Appl Surf Sci. 2007;254: 1145-1149. doi: 10.1016/j.apsusc.2007.09.021
- Chernyshikhin SV, Pelevin IA, Karimi F, Shishkovsky IV. The study on resolution factors of LPBF technology for manufacturing superelastic NiTi endodontic files. Materials (Basel). 2022;15: 6556. doi: 10.3390/ma15196556
- Bansiddhi A, Sargeant TD, Stupp SI, Dunand DC. Porous NiTi for bone implants: A review. Acta Biomater. 2008;4: 773-782. doi: 10.1016/j.actbio.2008.02.009
- Lagoudas DC, Entchev PB, Popov P, Patoor E, Brinson LC, Gao X. Shape memory alloys, part II: Modeling of polycrystals. Mech Mater. 2006;38: 430-462. doi: 10.1016/j.mechmat.2005.08.003
- Miyazaki S, Otsuka K. Development of shape memory alloys. ISIJ Int. 1989;29: 353-377. doi: 10.2355/isijinternational.29.353
- Hodgson D, Russell S. Nitinol melting, manufacture and fabrication. Minim Invasive Ther Allied Technol. 2000;9: 61-65. doi: 10.3109/13645700009063051
- Liu Y, Van Humbeeck J, Stalmans R, Stalmans R, Delaey L. Some aspects of the properties of NiTi shape memory alloy. J Alloys Compd. 1997;247: 115-121. doi: 10.1016/S0925-8388(96)02572-8
- Tang W, Sundman B, Sandström R, Qiu C. New modelling of the B2 phase and its associated martensitic transformation in the Ti-Ni system. Acta Mater. 1999;47: 3457-3468. doi: 10.1016/S1359-6454(99)00193-7
- Elahinia M, Koo J, Ahmadian M, Woolsey C. Backstepping control of a shape memory alloy actuated robotic arm. J Vib Control. 2005;11: 407-429. doi: 10.1177/1077546305051201
- Williams G. Book reviews. Crit Public Health. 2008;18: 425-427. doi: 10.1080/09581590802223709
- Hadi A, Yousefi-Koma A, Moghaddam MM, Elahinia M, Ghazavi A. Developing a novel SMA-actuated robotic module. Sensors Actuators A Phys. 2010;162: 72-81. doi: 10.1016/j.sna.2010.06.014
- Piquard R, D’Acunto A, Laheurte P, Dudzinski D. Micro-end milling of NiTi biomedical alloys, burr formation and phase transformation. Precis Eng. 2014;38: 356-364. doi: 10.1016/j.precisioneng.2013.11.006
- Lagoudas DC. Shape Memory Alloys. Boston, MA: Springer US. 2008. doi: 10.1007/978-0-387-47685-8
- Biermann D, Kahleyss F, Krebs E, Upmeier T. A study on micro-machining technology for the machining of NiTi: Five-axis micro-milling and micro deep-hole drilling. J Mater Eng Perform. 2011;20: 745-751. doi: 10.1007/s11665-010-9796-9
- Kanjwal K, Yeasting R, Maloney JD, et al. Retro-cardiac esophageal mobility and deflection to prevent thermal injury during atrial fibrillation ablation: an anatomic feasibility study. J Interv Card Electrophysio. 2011;30: 45-53. doi: 10.1007/s10840-010-9524-2
- Tarkesh Esfahani E, Elahinia MH. Developing an adaptive controller for a shape memory alloy walking assistive device. J Vib Control. 2010;16: 1897-1914. doi: 10.1177/1077546309344163
- Bobbert FSL, Lietaert K, Eftekhari AA, et al. Additively manufactured metallic porous biomaterials based on minimal surfaces: A unique combination of topological, mechanical, and mass transport properties. Acta Biomater. 2017;53: 572-584. doi: 10.1016/j.actbio.2017.02.024
- Gómez S, Vlad MD, López J, Fernández E. Design and properties of 3D scaffolds for bone tissue engineering. Acta Biomater. 2016;42: 341-350. doi: 10.1016/j.actbio.2016.06.032
- Van Bael S, Chai YC, Truscello S, et al. The effect of pore geometry on the in vitro biological behavior of human periosteum-derived cells seeded on selective laser-melted Ti6Al4V bone scaffolds. Acta Biomater. 2012;8: 2824-2834. doi: 10.1016/j.actbio.2012.04.001
- Chernyshikhin SV, Firsov DG, Shishkovsky IV. Selective laser melting of pre-alloyed NiTi powder: Single-track study and FE modeling with heat source calibration. Materials (Basel). 2021;14: 7486. doi: 10.3390/ma14237486
- Adams KL, Rebenfeld L. Permeability characteristics of multilayer fiber reinforcements. Part I: Experimental observations. Polym Compos. 1991;12: 179-185. doi: 10.1002/PC.750120307
- Syerko E, Schmidt T, May D, et al. Benchmark exercise on image-based permeability determination of engineering textiles: Microscale predictions. Compos Part A Appl Sci Manuf. 2023;167: 107397. doi: 10.1016/j.compositesa.2022.107397
- Silin D, Patzek T. Pore space morphology analysis using maximal inscribed spheres. Phys A Stat Mech Its Appl. 2006;371: 336-360. doi: 10.1016/j.physa.2006.04.048
- Ren Z, Wei D, Wang S, Zhang DZ, Mao S. On the role of pre- and postcontour scanning in laser powder bed fusion: Thermal-fluid dynamics and laser reflections. Int J Mech Sci. 2022;226: 107389. doi: 10.1016/J.IJMECSCI.2022.107389
- Tan C, Li S, Essa K, et al. Laser powder bed fusion of Ti-rich TiNi lattice structures: Process optimisation, geometrical integrity, and phase transformations. Int J Mach Tools Manuf. 2019;141: 19-29. doi: 10.1016/j.ijmachtools.2019.04.002
- Li X, Hao S, Du B, et al. High-performance self-expanding NiTi stents manufactured by laser powder bed fusion. Metal Mater Int. 2022;29: 1510-21. doi: 10.1007/s12540-022-01317-2
- Lv J, Jia Z, Li J, et al. Electron beam melting fabrication of porous Ti6Al4V scaffolds: Cytocompatibility and osteogenesis. Adv Eng Mater. 2015;17: 1391-1398. doi: 10.1002/ADEM.201400508
- Gu YW, Li H, Tay BY, Lim CS, Yong MS, Khor KA. In vitro bioactivity and osteoblast response of porous NiTi synthesized by SHS using nanocrystalline Ni-Ti reaction agent. J Biomed Mater Res Part A. 2006;78: 316-323. doi: 10.1002/JBM.A.30743