Fabrication and characterization of photosensitive non-isocyanate polyurethane acrylate resin for 3D printing of customized biocompatible orthopedic surgical guides
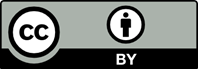
Three-dimensional (3D)-printed orthopedic surgical guides have the potential to provide personalized precision treatment. Non-isocyanate polyurethane (NIPU) is commonly used in the 3D printing of biomedical materials but its application in the orthopedic surgical guide is limited by poor mechanical properties and biocompatibility. In this study, we fabricated non-isocyanate polyurethane acrylate (NIPUA) photosensitive resin with superior biocompatibility and mechanical properties required for 3D-printed orthopedic surgical guides. NIPU prepolymer was synthesized by a ring-opening reaction and a ring acrylation reaction. NIPUA was further synthesized using polyethylene glycol diacrylate (PEGDA) as a modified material based on sustainable synthesis with reduced synthesis time. NIPUA showed the best tensile and flexural strengths when the PEGDA content reached 12 wt.%. NIPUA exhibited higher thermal stability, hemocompatibility, superior biocompatibility to ME3T3-E1 bone cells and C1C12 muscle cells, and non-immunogenic effect toward macrophages compared with commercial photosensitive resins. Commercial resins triggered a severe inflammatory response during in vivo implantation, but this effect was not observed during NIPUA implantation. Transcriptome analysis showed downregulation of cell death and cell cycle disruption-related genes, such as CDK2, CDKN1a, and GADD45a, and upregulation of autophagy and anti-tumor activity-related genes, such as MYC, PLK1, and BUB1b, in NIPUA-treated MC3T3-E1 cells compared with commercial resin-treated MC3T3-E1 cells. In conclusion, NIPUA resin showed excellent mechanical and thermal properties as well as good biocompatibility toward bone cells, muscle cells, and macrophages, suggesting its possible application in the 3D printing of customized orthopedic surgical guides.
1. Papadimitriou A, Mousoulea S, Gkantidis N, et al., 2018, Clinical effectiveness of Invisalign(R) orthodontic treatment: A systematic review. Prog Orthod, 19(1):37. https://doi.org/10.1186/s40510-018-0235-z
2. Xia JJ, Gateno J, Teichgraeber JF, 2009, New clinical protocol to evaluate craniomaxillofacial deformity and plan surgical correction. J Oral Maxillofac Surg, 67(10):2093–2106. https://doi.org/10.1016/j.joms.2009.04.057
3. Eglin D, Mortisen D, Alini M, 2009, Degradation of synthetic polymeric scaffolds for bone and cartilage tissue repairs. Soft Matter, 5(5):938–947.
4. Cui X, Breitenkamp K, Finn MG, et al., 2012, Direct human cartilage repair using three-dimensional bioprinting technology. Tissue Eng Part A, 18(11-12):1304–1312. https://doi.org/10.1089/ten.TEA.2011.0543
5. Prabhakaran MP, Venugopal J, Ramakrishna S, 2009, Electrospun nanostructured scaffolds for bone tissue engineering. Acta Biomater, 5(8):2884–2893. https://doi.org/10.1016/j.actbio.2009.05.007
6. Nadi A, Khodaei M, Javdani M, et al., 2022, Fabrication of functional and nano-biocomposite scaffolds using strontium-doped bredigite nanoparticles/polycaprolactone/ poly lactic acid via 3D printing for bone regeneration. Int J Biol Macromol, 219:1319–1336. https://doi.org/10.1016/j.ijbiomac.2022.08.136
7. Mahendiran B, Muthusamy S, Sampath S, et al., 2021, Recent trends in natural polysaccharide based bioinks for multiscale 3D printing in tissue regeneration: A review. Int J Biol Macromol, 183:564–588. https://doi.org/10.1016/j.ijbiomac.2021.04.179
8. Singhal P, Small W, Cosgriff-Hernandez E, et al., 2014, Low density biodegradable shape memory polyurethane foams for embolic biomedical applications. Acta Biomater, 10(1):67–76. https://doi.org/10.1016/j.actbio.2013.09.027
9. Sun N, Di M, Liu Y, 2021, Lignin-containing polyurethane elastomers with enhanced mechanical properties via hydrogen bond interactions. Int J Biol Macromol, 184:1–8. https://doi.org/10.1016/j.ijbiomac.2021.06.038
10. Gunatillake PA, Dandeniyage LS, Adhikari R, et al., 2019, Advancements in the development of biostable polyurethanes, Polym Rev, 59(3):391–417. https://doi.org/10.1080/15583724.2018.1493694
11. Zhou X, Ren Z, Sun H, et al., 2022, 3D printing with high content of lignin enabled by introducing polyurethane, Int J Biol Macromol, 221:1209–1217. https://doi.org/10.1016/j.ijbiomac.2022.09.076
12. Panwar H, Raghuram GV, Jain D, et al., 2014, Cell cycle deregulation by methyl isocyanate: Implications in liver carcinogenesis. Environ Toxicol, 29(3):284–297. https://doi.org/10.1002/tox.21757
13. Ochiai B, Inoue S, Endo T, 2005, One-pot non-isocyanate synthesis of polyurethanes from bisepoxide, carbon dioxide, and diamine. J Polym Sci A: Polym Chem, 43(24):6613–6618. https://doi.org/10.1002/pola.21103
14. Błażek K, Datta J, 2019, Renewable natural resources as green alternative substrates to obtain bio-based non-isocyanate polyurethanes-review. Crit Rev Environ Sci Technol, 49(3):173–211. https://doi.org/10.1080/10643389.2018.1537741
15. Gomez-Lopez A, Elizalde F, Calvo I, et al., 2021, Trends in non-isocyanate polyurethane (NIPU) development. Chem Commun (Camb), 57(92):12254–12265. https://doi.org/10.1039/d1cc05009e
16. Pyo S-H, Persson P, Mollaahmad MA, et al., 2011, Cyclic carbonates as monomers for phosgene- and isocyanate-free polyurethanes and polycarbonates. Pure Appl Chem, 84(3):637–661. https://doi.org/10.1351/PAC-CON-11-06-14
17. Heederik D, Henneberger PK, Redlich CA, et al., 2012, Primary prevention: Exposure reduction, skin exposure and respiratory protection. Eur Respir Rev, 21(124):112–124. https://doi.org/10.1183/09059180.00005111
18. Kim S, Lee H, Choi H, et al., 2022, Investigation on photopolymerization of PEGDA to fabricate high-aspect-ratio microneedles. RSC Adv, 12(16):9550–9555. https://doi.org/10.1039/D2RA00189F
19. Duan J, Cao Y, Shen Z, et al., 2022, 3D bioprinted GelMA/ PEGDA hybrid scaffold for establishing an in vitro model of melanoma. J Microbiol Biotechnol, 32(4):531–540. https://doi.org/10.4014/jmb.2111.11003
20. Bao Z, Gao M, Fan X, et al., 2020, Development and characterization of a photo-cross-linked functionalized type-I collagen (Oreochromis niloticus) and polyethylene glycol diacrylate hydrogel. Int J Biol Macromol, 155:163–173. https://doi.org/10.1016/j.ijbiomac.2020.03.210
21. Asemani H, Zareanshahraki F, Mannari V, 2019, Design of hybrid nonisocyanate polyurethane coatings for advanced ambient temperature curing applications. J Appl Polym Sci, 136(13):47266. https://doi.org/10.1002/app.47266
22. Donnelly J, Hernández FE, 2018, Trends in bond dissociation energies for the homolytic cleavage of successive molecular bonds. J Chem Educ, 95(9):1672–1678. https://doi.org/10.1021/acs.jchemed.7b00962
23. Beniah G, Uno BE, Lan T, et al., 2017, Tuning nanophase separation behavior in segmented polyhydroxyurethane via judicious choice of soft segment. Polymer, 110:218–227. https://doi.org/10.1016/j.polymer.2017.01.017
24. Mo O, Yanez M, Eckert-Maksic M, et al., 2005, Periodic trends in bond dissociation energies. A theoretical study. J Phys Chem A, 109(19):4359–4365. https://doi.org/10.1021/jp050857o
25. Ghanemi A, Melouane A, Yoshioka M, et al., 2019, Secreted protein acidic and rich in cysteine and bioenergetics: Extracellular matrix, adipocytes remodeling and skeletal muscle metabolism. Int J Biochem Cell Biol, 117:105627. https://doi.org/10.1016/j.biocel.2019.105627
26. Jiang R, Wang M, Shi L, et al., 2019, Panax ginseng total protein facilitates recovery from dexamethasone-induced muscle atrophy through the activation of glucose consumption in C2C12 myotubes. Biomed Res Int, 2019:3719643. https://doi.org/10.1155/2019/3719643
27. Hur S, Kim JH, Yun J, et al., 2020, Protein phosphatase 1H, cyclin-dependent kinase inhibitor p27, and cyclin-dependent kinase 2 in paclitaxel resistance for triple negative breast cancers. J Breast Cancer, 23(2):162–170. https://doi.org/10.4048/jbc.2020.23.e20
28. Wang Y, Xie BH, Lin WH, et al., 2019, Amplification of SMYD3 promotes tumorigenicity and intrahepatic metastasis of hepatocellular carcinoma via upregulation of CDK2 and MMP2. Oncogene, 38(25):4948–4961. https://doi.org/10.1038/s41388-019-0766-x
29. Seng NS, Megyesi J, Tarcsafalvi A, et al., 2016, Mimicking Cdk2 phosphorylation of Bcl-xL at Ser73 results in caspase activation and Bcl-xL cleavage. Cell Death Discov, 2(1):16001. https://doi.org/10.1038/cddiscovery.2016.1
30. Barreto G, Schafer A, Marhold J, et al., 2007, Gadd45a promotes epigenetic gene activation by repair-mediated DNA demethylation. Nature, 445(7128):671–675. https://doi.org/10.1038/nature05515
31. Liu J, Jiang G, Mao P, et al., 2018, Down-regulation of GADD45A enhances chemosensitivity in melanoma. Sci Rep, 8(1):4111. https://doi.org/10.1038/s41598-018-22484-6
32. Arab K, Karaulanov E, Musheev M, et al., 2019, GADD45A binds R-loops and recruits TET1 to CpG island promoters. Nat Genet, 51(2):217–223. https://doi.org/10.1038/s41588-018-0306-6
33. Satta N, Weppe R, Pagano S, et al., 2020, Auto-antibodies against apolipoprotein A-1 block cancer cells proliferation and induce apoptosis. Oncotarget, 11(46):4266–4280. https://doi.org/10.18632/oncotarget.27814
34. Zhan Q, 2005, Gadd45a, a p53- and BRCA1-regulated stress protein, in cellular response to DNA damage. Mutat Res, 569(1-2):133–43. https://doi.org/10.1016/j.mrfmmm.2004.06.055
35. Wang Y, Zhang J, Li J, et al., 2019, CircRNA_014511 affects the radiosensitivity of bone marrow mesenchymal stem cells by binding to miR-29b-2-5p. Bosn J Basic Med Sci, 19(2):155–163. https://doi.org/10.17305/bjbms.2019.3935
36. Mattia M, Gottifredi V, McKinney K, et al., 2007, p53- dependent p21 mRNA elongation is impaired when DNA replication is stalled. Mol Cell Biol, 27(4):1309–1320. https://doi.org/10.1128/MCB.01520-06
37. Chen YC, Chang MY, Shiau AL, et al., 2010, Mitochondrial ribosomal protein S36 delays cell cycle progression in association with p53 modification and p21(WAF1/CIP1) expression. J Cell Biochem, 100(4):981–990.
38. Dang CV, 2013, MYC, metabolism, cell growth, and tumorigenesis. Cold Spring Harb Perspect Med, 3(8):a014217. https://doi.org/10.1101/cshperspect.a014217
39. Chou YT, Lin HH, Lien YC, et al., 2010, EGFR promotes lung tumorigenesis by activating miR-7 through a Ras/ERK/ Myc pathway that targets the Ets2 transcriptional repressor ERF. Cancer Res, 70(21):8822–8831. https://doi.org/10.1158/0008-5472.CAN-10-0638
40. Palomero T, Lim WK, Odom DT, et al., 2006, NOTCH1 directly regulates c-MYC and activates a feed-forward-loop transcriptional network promoting leukemic cell growth. Proc Natl Acad Sci U S A, 103(48):18261–18266. https://doi.org/10.1073/pnas.0606108103
41. Konishi H, Asano N, Imatani A, et al., 2016, Notch1 directly induced CD133 expression in human diffuse type gastric cancers. Oncotarget, 7(35):56598–56607. https://doi.org/10.18632/oncotarget.10967
42. Liu K, Zheng M, Lu R, et al., 2020, The role of CDC25C in cell cycle regulation and clinical cancer therapy: A systematic review. Cancer Cell Int, 20:213. https://doi.org/10.1186/s12935-020-01304-w
43. Alfaro E, Lopez-Jimenez P, Gonzalez-Martinez J, et al., 2021, PLK1 regulates centrosome migration and spindle dynamics in male mouse meiosis. EMBO Rep, 22(4):e51030. https://doi.org/10.15252/embr.202051030
44. Jang MS, Lee SJ, Kim CJ, et al., 2011, Phosphorylation by polo-like kinase 1 induces the tumor-suppressing activity of FADD. Oncogene, 30(4):471–481. https://doi.org/10.1038/onc.2010.423
45. Basu J, Logarinho E, Herrmann S, et al., 1998, Localization of the drosophila checkpoint control protein Bub3 to the kinetochore requires Bub1 but not Zw10 or Rod. Chromosoma, 107(6-7):376–385.
46. Basu J, Bousbaa H, Logarinho E, et al., 1999, Mutations in the essential spindle checkpoint gene bub1 cause chromosome missegregation and fail to block apoptosis in Drosophila. J Cell Biol, 146(1):13–28. https://doi.org/10.1083/jcb.146.1.13
47. Sharp-Baker H, Chen RH, 2001, Spindle checkpoint protein Bub1 is required for kinetochore localization of Mad1, Mad2, Bub3, and Cenp-E, independently of its kinase activity. J Cell Biol, 153(6):1239–1250.
48. Nath D, Shadan S, 2009, The ubiquitin system. Nature, 458(7237):421. https://doi.org/10.1038/458421a
49. Jeganathan K, Malureanu L, Baker DJ, et al., 2007, Bub1 mediates cell death in response to chromosome missegregation and acts to suppress spontaneous tumorigenesis. J Cell Biol, 179(2):255–267.