3D-printed dual drug delivery nanoparticleloaded hydrogels to combat antibiotic-resistant bacteria
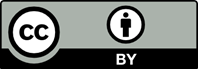
Implant-associated infections are not easy to diagnose and very difficult to treat, due to the ability of major pathogens, such as Staphylococcus aureus, to develop biofilms and escape the immune response and antibiotic treatment. We, therefore, aimed to develop a 3D-printed dual rifampicin (Rif)- and vancomycin (Van)-loaded polylacticco-glycolic acid (PLGA) nanoparticles (NPs) delivery system based on hydrogels made of gelatin methacrylate (GelMA). The release of Rif and Van from NPs manufactured from different PLGA molecular weights was studied in phosphate-buffered saline for 21 days. Low molecular weight PLGA NPs exhibited the fastest release of Rif and Van within the first 7 days and were selected for antimicrobial evaluation. Four different GelMA-based 3D-printed samples were successfully produced, carrying non-loaded NPs, Rif-NPs, Van-NPs, or alternating layers of Rif-NPs and Van-NP. The exposition of S. aureus against increased concentrations of Rif or Van produced new resistant strains to Rif (RifR ) or Van (VanR ). The GelMA hydrogel co-delivering Rif and Van eradicated S. aureus RN4220 RifR and RN4220 VanR strains. S. aureus RN4220 and S. aureus AMC 201 colonies developed resistance to Rif after contact with the GelMA hydrogel containing only Rif-NPs which appeared to be due to known mutations in the rpoB gene. In conclusion, 3D-printed GelMA hydrogel loaded with PLGA Rif-Van-NPs drug delivery system show promising in vitro results to prevent implant-associated infections caused by antimicrobial-resistant bacteria.
1. ter Boo G-JA, Grijpma DW, Moriarty TF, et al., 2015, Antimicrobial delivery systems for local infection prophylaxis in orthopedic- and trauma surgery. Biomaterials, 52:113–125. https://doi.org/10.1016/j.biomaterials.2015.02.020
2. Masters EA, Trombetta RP, de Mesy Bentley KL, et al., 2019, Evolving concepts in bone infection: Redefining “biofilm,” “acute vs. chronic osteomyelitis,” “the Immune Proteome” and “local antibiotic therapy.” Bone Res, 7(1):20. https://doi.org/10.1038/s41413-019-0061-z
3. Kavanagh N, Ryan EJ, Widaa A, et al., 2018, Staphylococcal osteomyelitis: Disease progression, treatment challenges, and future directions. Clin Microbiol Reviews, 31(2):e00084- 17, /cmr/31/2/e00084-17.atom. https://doi.org/10.1128/CMR.00084-17
4. Zimmerli W, Trampuz A, 2011, Implant-associated infection, in Biofilm Infections, Bjarnsholt T, Jensen PØ, Moser C, et al., Eds., Springer New York, New York, NY, 69–90. https://doi.org/10.1007/978-1-4419-6084-9_5
5. Riool M, de Boer L, Jaspers V, et al., 2014, Staphylococcus epidermidis originating from titanium implants infects surrounding tissue and immune cells. Acta Biomater, 10(12):5202–5212. https://doi.org/10.1016/j.actbio.2014.08.012
6. Morgenstern M, Vallejo A, McNally MA, et al., 2018, The effect of local antibiotic prophylaxis when treating open limb fractures: A systematic review and meta-analysis. Bone Joint Res, 7(7):447–456. https://doi.org/10.1302/2046-3758.77.BJR-2018-0043.R1
7. Turgut H, Sacar S, Kaleli I, et al., 2005, Systemic and local antibiotic prophylaxis in the prevention of Staphylococcus epidermidis graft infection. BMC Infect Dis, 5(1):91. https://doi.org/10.1186/1471-2334-5-91
8. Hendriks JGE, Neut D, van Horn JR, et al., 2005, Bacterial survival in the interfacial gap in gentamicin-loaded acrylic bone cements. J Bone Joint Surg Br Vol, 87-B(2):272–276. https://doi.org/10.1302/0301-620X.87B2.14781
9. Achermann Y, Eigenmann K, Ledergerber B, et al., 2013, Factors associated with rifampin resistance in Staphylococcal periprosthetic joint infections (PJI): A matched case–control study. Infection, 41(2):431–437. https://doi.org/10.1007/s15010-012-0325-7
10. Papkou A, Hedge J, Kapel N, et al., 2020, Efflux pump activity potentiates the evolution of antibiotic resistance across S. aureus isolates. Nat Commun, 11(1):3970. https://doi.org/10.1038/s41467-020-17735-y
11. Zimmerli W, Sendi P, 2019, Role of rifampin against Staphylococcal biofilm infections in vitro, in animal models, and in orthopedic-device-related infections. Antimicrob Agents Chemother, 63(2):e01746-18. https://doi.org/10.1128/AAC.01746-18
12. Beeching NJ, Thomas MG, Roberts S, et al., 1986, Comparative in-vitro activity of antibiotics incorporated in acrylic bone cement. J Antimicrob Chemother, 17(2):173–184. https://doi.org/10.1093/jac/17.2.173
13. Darouiche RO, Mansouri MD, Zakarevicz D, et al., 2007, In vivo efficacy of antimicrobial-coated devices. J Bone Joint Surg, 89(4):792–797. https://doi.org/10.2106/JBJS.F.00414
14. Inzana J, Trombetta R, Schwarz E, et al., 2015, 3D printed bioceramics for dual antibiotic delivery to treat implant-associated bone infection. eCM, 30:232–247. https://doi.org/10.22203/eCM.v030a16
15. Sanz-Ruiz P, Carbó-Laso E, Del Real-Romero JC, et al., 2017, Microencapsulation of rifampicin: A technique to preserve the mechanical properties of bone cement: Rifampicin-loaded polymethylmethacrylate. J Orthop Res, 36(1):459–466 https://doi.org/10.1002/jor.23614
16. Zadpoor AA, Malda J, 2017, Additive manufacturing of biomaterials, tissues, and organs. Ann Biomed Eng, 45(1):1–11. https://doi.org/10.1007/s10439-016-1719-y
17. Alexander AE, Wake N, Chepelev L, et al., 2021, A guideline for 3D printing terminology in biomedical research utilizing ISO/ASTM standards. 3D Print Med, 7(1):8. https://doi.org/10.1186/s41205-021-00098-5
18. Ziaee M, Crane NB, 2019, Binder jetting: A review of process, materials, and methods. Addit Manufac, 28:781–801. https://doi.org/10.1016/j.addma.2019.05.031
19. Basit AW, Gaisford S, Eds, 2018, 3D printing of pharmaceuticals, in AAPS Advances in the Pharmaceutical Sciences Series, vol. 31, Springer International Publishing, Cham https://doi.org/10.1007/978-3-319-90755-0.
20. Murr LE, Gaytan SM, Ramirez DA, et al., 2012, Metal fabrication by additive manufacturing using laser and electron beam melting technologies. J Mater Sci Technol, 28(1):1–14. https://doi.org/10.1016/S1005-0302(12)60016-4
21. Jiang T, Munguia-Lopez JG, Flores-Torres S, et al., 2019, Extrusion bioprinting of soft materials: An emerging technique for biological model fabrication. Appl Phys Rev, 6(1):011310. https://doi.org/10.1063/1.5059393
22. Zhuang P, Ng WL, An J, et al., 2019, Layer-by-layer ultraviolet assisted extrusion-based (UAE) bioprinting of hydrogel constructs with high aspect ratio for soft tissue engineering applications. PLoS One, 14(6):e0216776. https://doi.org/10.1371/journal.pone.0216776
23. Li X, Liu B, Pei B, et al., 2020, Inkjet bioprinting of biomaterials. Chem Rev, 120(19):10793–10833. https://doi.org/10.1021/acs.chemrev.0c00008
24. Ng WL, Huang X, Shkolnikov V, et al., 2021, Controlling droplet impact velocity and droplet volume: Key factors to achieving high cell viability in sub-nanoliter droplet-based bioprinting. Int J Bioprint, 8(1):424. https://doi.org/10.18063/ijb.v8i1.424
25. Sing SL, An J, Yeong WY, et al., 2016, Laser and electron-beam powder-bed additive manufacturing of metallic implants: A review on processes, materials and designs: Laser and electron-beam additive manufacturing of metallic implants. J Orthop Res, 34(3):369–385. https://doi.org/10.1002/jor.23075
26. Dermeik B, Travitzky N, 2020, Laminated object manufacturing of ceramic‐based materials. Adv Eng Mater, 22(9):2000256. https://doi.org/10.1002/adem.202000256
27. Li W, Mille LS, Robledo JA, et al., 2020, Recent advances in formulating and processing biomaterial inks for vat polymerization‐based 3D printing. Adv Healthcare Mater, 9(15):2000156. https://doi.org/10.1002/adhm.202000156
28. Ng WL, Lee JM, Zhou M, et al., 2020, Vat polymerization-based bioprinting—Process, materials, applications and regulatory challenges. Biofabrication, 12(2):022001. https://doi.org/10.1088/1758-5090/ab6034
29. Slaughter BV, Khurshid SS, Fisher OZ, et al., 2009, Hydrogels in regenerative medicine. Adv Mater, 21(32–33):3307–3329. https://doi.org/10.1002/adma.200802106
30. Bohara S, Suthakorn J, 2022, Surface coating of orthopedic implant to enhance the osseointegration and reduction of bacterial colonization: A review. Biomater Res, 26(1):26. https://doi.org/10.1186/s40824-022-00269-3
31. Liu M, Zeng X, Ma C, et al., 2017, Injectable hydrogels for cartilage and bone tissue engineering. Bone Res, 5(1):17014. https://doi.org/10.1038/boneres.2017.14
32. Ottenbrite, R. M., Park, K., Okano, T., Eds.;, 2010, Biomedical Applications of Hydrogels Handbook, Springer New York, New York, NY. https://doi.org/10.1007/978-1-4419-5919-5
33. Celikkin N, Mastrogiacomo S, Jaroszewicz J, et al., 2018, Gelatin methacrylate scaffold for bone tissue engineering: The influence of polymer concentration: Gelatin methacrylate scaffold for bone tissue engineering. J Biomed Mater Res, 106(1):201–209. https://doi.org/10.1002/jbm.a.36226
34. Wang Y, Qin B, Xia G, 2021, FDA’s poly (lactic-co-glycolic acid) research program and regulatory outcomes. AAPS J, 23(4). https://doi.org/10.1208/s12248-021-00611-y
35. Anjum A, Chung P-Y, Ng S-F, 2019, PLGA/xylitol nanoparticles enhance antibiofilm activity via penetration into biofilm extracellular polymeric substances. RSC Adv, 9(25):14198–14208. https://doi.org/10.1039/C9RA00125E
36. Toti US, 2011, Targeted delivery of antibiotics to intracellular chlamydial infections using PLGA nanoparticles. Biomaterials, 32(27):6606–6613. https://doi.org/10.1016/j.biomaterials.2011.05.038
37. Khalil NM, Nascimento TCFd, Casa DM, et al., 2013, Pharmacokinetics of curcumin-loaded PLGA and PLGA– PEG blend nanoparticles after oral administration in rats. Colloids Surf B Biointerfaces, 101:353–360. https://doi.org/10.1016/j.colsurfb.2012.06.024
38. Wang H, Zhao Y, Wu Y, et al., 2011, Enhanced anti-tumor efficacy by co-delivery of doxorubicin and paclitaxel with amphiphilic methoxy PEG-PLGA copolymer nanoparticles. Biomaterials, 32(32):8281–8290. https://doi.org/10.1016/j.biomaterials.2011.07.032
39. Rooijakkers SHM, van Wamel WJB, Ruyken M, et al., 2005, Anti-opsonic properties of staphylokinase. Microbes Infect, 7(3):476–484. https://doi.org/10.1016/j.micinf.2004.12.014
40. Kwakman PHS, Velde AAte, Boer L, et al., 2010, How honey kills bacteria. FASEB J, 24(7):2576–2582. https://doi.org/10.1096/fj.09-150789
41. Kwakman PHS, te Velde AA, de Boer L, et al., 2011, Two major medicinal honeys have different mechanisms of bactericidal activity. PLoS One, 6(3):e17709. https://doi.org/10.1371/journal.pone.0017709
42. Aubry-Damon H, Soussy C-J, Courvalin P, 1998, Characterization of mutations in the RpoB gene that confer rifampin resistance in Staphylococcus aureus. Antimicrob Agents Chemother, 42(10):2590–2594. https://doi.org/10.1128/AAC.42.10.2590
43. Boyle-Vavra S, Berke SK, Lee JC, et al., 2000, Reversion of the glycopeptide resistance phenotype in Staphylococcus aureus clinical isolates. Antimicrob Agents Chemother, 44(2):272–277. https://doi.org/10.1128/AAC.44.2.272-277.2000
44. Kang H, Shih Y-RV, Hwang Y, et al., 2014, Mineralized gelatin methacrylate-based matrices induce osteogenic differentiation of human induced pluripotent stem cells. Acta Biomater, 10(12):4961–4970. https://doi.org/10.1016/j.actbio.2014.08.010
45. ter Boo G-JA, Grijpma DW, Moriarty TF, et al., 2015, Antimicrobial delivery systems for local infection prophylaxis in orthopedic- and trauma surgery. Biomaterials, 52:113–125. https://doi.org/10.1016/j.biomaterials.2015.02.020
46. Pitarresi G, Palumbo FS, Calascibetta F, et al., 2013, Medicated hydrogels of hyaluronic acid derivatives for use in orthopedic field. Int J Pharm, 449(1–2):84–94. https://doi.org/10.1016/j.ijpharm.2013.03.059
47. Makino K, Nakajima T, Shikamura M, et al., 2004, Efficient intracellular delivery of rifampicin to alveolar macrophages using rifampicin-loaded PLGA microspheres: Effects of molecular weight and composition of PLGA on release of rifampicin. Colloids Surf B Biointerfaces, 36(1):35–42. https://doi.org/10.1016/j.colsurfb.2004.03.018
48. Özalp Y, Özdemir N, Kocag S, et al., 2001, Controlled release of vancomycin from biodegradable microcapsules. J Microencapsul, 18(1):89–110. https://doi.org/10.1080/026520401750038638
49. Alexis F, 2005, Factors affecting the degradation and drug-release mechanism of poly(lactic acid) and poly[(lactic acid)-co-(glycolic acid)]. Polym Int, 54(1):36–46. https://doi.org/10.1002/pi.1697
50. Wi YM, Greenwood-Quaintance KE, Brinkman CL, et al., 2018, Rifampicin resistance in Staphylococcus epidermidis: Molecular characterisation and fitness cost of RpoB mutations. Int J Antimicrob Agents, 51(5): 670–677. https://doi.org/10.1016/j.ijantimicag.2017.12.019
51. O’Neill A, Oliva B, Storey C, et al., 2000, RNA polymerase inhibitors with activity against rifampin-resistant mutants of Staphylococcus aureus. Antimicrob Agents Chemother, 44(11):3163–3166. https://doi.org/10.1128/AAC.44.11.3163-3166.2000
52. Williams K, 1998, Accumulation of rifampicin by Escherichia coli and Staphylococcus aureus. J Antimicrob Chemother, 42(5):597–603. https://doi.org/10.1093/jac/42.5.597
53. Goldstein BP, 2014, Resistance to rifampicin: A review. J Antibiot, 67(9):625–630. https://doi.org/10.1038/ja.2014.107