Melt electrowriting-printed peritoneal scaffold prevents peritoneal adhesion and facilitates peritoneal repair
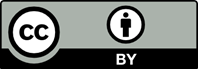
Peritoneal adhesion is a critical issue after abdominal surgery. Cell-based methods for preventing peritoneal adhesion have not yet been fully investigated. Here, we constructed a highly biomimetic peritoneal scaffold by seeding mesothelial cells, the natural physiological barrier of the peritoneum, onto a melt electrowriting-printed scaffold. The scaffolds with the microfibers crossed at different angles (30°, 60°, and 90°) were screened based on mesothelial cell proliferation and orientation. Thirty degrees were more suitable for improving proliferation of mesothelial cells and cell growth in a single direction; therefore, the 30° peritoneal scaffold could better mimic the physiological structure of native peritoneum. Mechanistically, such a peritoneal scaffold was able to act as a barrier to prevent peritoneal resident macrophages from migrating to the site of the peritoneal lesion. In vivo mesothelial cell tracking using lentivirus technology confirmed that the peritoneal scaffold, compared to the scaffold without mesothelial cells, could prevent peritoneal adhesion and was directly involved in the repair of injured peritoneum. This study suggests that the peritoneal scaffolds can potentially prevent peritoneal adhesion, offering a new approach for clinical treatment.
1. Molegraaf MJ, Torensma B, Lange CP, et al., 2017, Twelve-year outcomes of laparoscopic adhesiolysis in patients with chronic abdominal pain: A randomized clinical trial. Surgery, 161(2):415–421. https://doi.org/10.1016/j.surg.2016.08.014
2. Herrick SE, Allen JE, 2021, Surgical adhesions: A sticky macrophage problem. Science, 371(6533):993–994. https://doi.org/10.1126/science.abg5416
3. Ten BR, Issa Y, van Santbrink EJ, et al., 2013, Burden of adhesions in abdominal and pelvic surgery: Systematic review and met-analysis. BMJ, 347(oct03 1):f5588. https://doi.org/10.1136/bmj.f5588
4. Krielen P, Stommel MWJ, Pargmae P, et al., 2020, Adhesion-related readmissions after open and laparoscopic surgery: A retrospective cohort study (SCAR update). Lancet (British edition), 395(10217):33–41. https://doi.org/10.1016/S0140-6736(19)32636-4
5. Wiseman DM, 2008, Disorders of adhesions or adhesion-related disorder: Monolithic entities or part of something bigger--CAPPS? Semin Reprod Med, 26(4):356–368. https://doi.org/10.1055/s-0028-1082394
6. Tang J, Xiang Z, Bernards MT, et al., 2020, Peritoneal adhesions: Occurrence, prevention and experimental models. Acta Biomater, 116:84–104. https://doi.org/10.1016/j.actbio.2020.08.036
7. Trochsler M, Maddern GJ, 2014, Adhesion barriers for abdominal surgery: A sticky problem. Lancet, 383(9911):8–10. https://doi.org/10.1016/S0140-6736(13)62002-4
8. Ten BR, Stommel M, Strik C, et al., 2014, Benefits and harms of adhesion barriers for abdominal surgery: A systematic review and meta-analysis. Lancet, 383(9911):48–59. https://doi.org/10.1016/S0140-6736(13)61687-6
9. Carmichael SP, Shin J, Vaughan JW, et al., 2022, Regenerative medicine therapies for prevention of abdominal adhesions: A scoping review. J Surg Res, 275:252–264. https://doi.org/10.1016/j.jss.2022.02.005
10. Ito T, Shintani Y, Fields L, et al., 2021, Cell barrier function of resident peritoneal macrophages in post-operative adhesions. Nat Commun, 12(1):2232. https://doi.org/10.1038/s41467-021-22536-y.
11. Inagaki NF, Inagaki FF, Kokudo N, et al., 2015, Use of mouse liver mesothelial cells to prevent postoperative adhesion and promote liver regeneration after hepatectomy. J Hepatol, 62(5):1141–1147. https://doi.org/10.1016/j.jhep.2014.12.010
12. van Baal JO, Van de Vijver KK, Nieuwland R, et al., 2017, The histophysiology and pathophysiology of the peritoneum. Tissue Cell, 49(1):95–105. https://doi.org/10.1016/j.tice.2016.11.004
13. Mutsaers SE, Prele CM, Pengelly S, et al., 2016, Mesothelial cells and peritoneal homeostasis. Fertil Steril, 106(5): 1018–1024. https://doi.org/10.1016/j.fertnstert.2016.09.005
14. Capobianco A, Cottone L, Monno A, et al., 2017, The peritoneum: Healing, immunity, and diseases. J Pathol, 243(2):137–147. https://doi.org/10.1002/path.4942
15. Mutsaers SE, Prêle CM, Pengelly S, et al., 2016, Mesothelial cells and peritoneal homeostasis. Fertil Steril, 106(5):1018–1024. https://doi.org/10.1016/j.fertnstert.2016.09.005
16. Koffler J, Zhu W, Qu X, et al., 2019, Biomimetic 3D-printed scaffolds for spinal cord injury repair. Nat Med, 25(2):263–269. https://doi.org/10.1038/s41591-018-0296-z
17. Kim BS, Kwon YW, Kong JS, et al., 2018, 3D cell printing of in vitro stabilized skin model and in vivo pre-vascularized skin patch using tissue-specific extracellular matrix bioink: A step towards advanced skin tissue engineering. Biomaterials, 168:38–53. https://doi.org/10.1016/j.biomaterials.2018.03.040
18. Murphy SV, De Coppi P, Atala A, 2020, Opportunities and challenges of translational 3D bioprinting. Nat Biomed Eng, 4(4):370–380. https://doi.org/10.1038/s41551-019-0471-7
19. Mandrycky C, Wang Z, Kim K, et al., 2016, 3D bioprinting for engineering complex tissues. Biotechnol Adv, 34(4):422–434. https://doi.org/10.1016/j.biotechadv.2015.12.011
20. Ye W, Xie C, Liu Y, et al., 2021, 3D printed high-resolution scaffold with hydrogel microfibers for providing excellent biocompatibility. J Biomater Appl, 35(6):633–642. https://doi.org/10.1177/0885328220962606
21. Tylek T, Blum C, Hrynevich A, et al., 2020, Precisely defined fiber scaffolds with 40 μm porosity induce elongation driven M2-like polarization of human macrophages. Biofabrication, 12(2):25007. https://doi.org/10.1088/1758-5090/ab5f4e
22. Huang J, Liu Y, Chi X, et al., 2021, Programming electronic skin with diverse skin-like properties. J Mater Chem A, 9(2):963–973. https://doi.org/10.1039/D0TA09101D
23. Huang J, Jiang Y, Liu Y, et al., 2021, Marine-inspired molecular mimicry generates a drug-free, but immunogenic hydrogel adhesive protecting surgical anastomosis. Bioact Mater, 6(3):770–782. https://doi.org/10.1016/j.bioactmat.2020.09.010
24. Ito T, Shintani Y, Fields L, et al., 2021, Cell barrier function of resident peritoneal macrophages in post-operative adhesions. Nat Commun, 12(1):2232. https://doi.org/10.1038/s41467-021-22536-y
25. Tsai JM, Sinha R, Seita J, et al., 2018, Surgical adhesions in mice are derived from mesothelial cells and can be targeted by antibodies against mesothelial markers. Sci Transl Med, 10(469):n6735. https://doi.org/10.1126/scitranslmed.aan6735
26. Zindel J, Peiseler M, Hossain M, et al., 2021, Primordial GATA6 macrophages function as extravascular platelets in sterile injury. Science, 371(6533):e595. https://doi.org/10.1126/science.abe0595
27. Zhang W, Wang X, Ma J, et al., 2022, Adaptive injectable carboxymethyl cellulose/poly (gamma-glutamic acid) hydrogels promote wound healing. Biomater Adv, 136:212753. https://doi.org/10.1016/j.bioadv.2022.212753
28. Xie C, Gao Q, Wang P, et al., 2019, Structure-induced cell growth by 3D printing of heterogeneous scaffolds with ultrafine fibers. Mater Design, 181:108092. https://doi.org/10.1016/j.matdes.2019.108092
29. Laronda MM, Rutz AL, Xiao S, et al., 2017, A bioprosthetic ovary created using 3D printed microporous scaffolds restores ovarian function in sterilized mice. Nat Commun, 8(1):15261. https://doi.org/10.1038/ncomms15261
30. Liang W, He W, Huang R, et al., 2022, Peritoneum‐inspired Janus porous hydrogel with anti‐deformation, anti‐adhesion, and pro‐healing characteristics for abdominal wall defect treatment. Adv Mater, 34(15):2108992. https://doi.org/10.1002/adma.202108992
31. Li Y, Lv S, Yuan H, et al., 2021, Peripheral nerve regeneration with 3D printed bionic scaffolds loading neural crest stem cell derived Schwann cell progenitors. Adv Funct Mater, 31(16):2010215. https://doi.org/https://doi.org/10.1002/adfm.202010215