Bioprinting with human stem cell-laden alginate-gelatin bioink and bioactive glass for tissue engineering
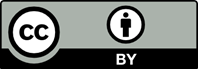
Three-dimensional (3D) bioprinting technologies have shown great potential in the fabrication of 3D models for different human tissues. Stem cells are an attractive cell source in tissue engineering as they can be directed by material and environmental cues to differentiate into multiple cell types for tissue repair and regeneration. In this study, we investigate the viability of human adipose-derived mesenchymal stem cells (ASCs) in alginate-gelatin (Alg-Gel) hydrogel bioprinted with or without bioactive glass. Highly angiogenic borate bioactive glass (13-93B3) in 50 wt% is added to polycaprolactone (PCL) to fabricate scaffolds using a solvent-based extrusion 3D bioprinting technique. The fabricated scaffolds with 12 × 12 × 1 mm3 in overall dimensions are physically characterized, and the glass dissolution from PCL/glass composite over a period of 28 days is studied. Alg-Gel composite hydrogel is used as a bioink to suspend ASCs, and scaffolds are then bioprinted in different configurations: Bioink only, PCL+bioink, and PCL/glass+bioink, to investigate ASC viability. The results indicate the feasibility of the solvent-based bioprinting process to fabricate 3D cellularized scaffolds with more than 80% viability on day 0. The decrease in viability after 7 days due to glass concentration and static culture conditions is discussed. The feasibility of modifying Alg-Gel with 13-93B3 glass for bioprinting is also investigated, and the results are discussed.
1. Moroni L, Boland T, Burdick JA, et al., 2018, Biofabrication: A Guide to Technology and Terminology. Trends Biotechnol, 36(4):384-402. DOI 10.1016/j.tibtech.2017.10.015.
2. Ozbolat IT, Hospodiuk M, 2016, Current Advances and Future Perspectives in Extrusion-based Bioprinting. Biomaterials, 76:321-43. DOI 10.1016/j.biomaterials.2015.10.076.
3. Pati F, Gantelius J, Svahn HA, 2016, 3D Bioprinting of Tissue/Organ Models. Angew Chemie Int Ed, 55(15):4650- 65. DOI 10.1002/anie.201505062.
4. Choudhury D, Anand S, Naing M, 2018, The Arrival of Commercial Bioprinters Towards 3D Bioprinting Revolution! Int J Bioprinting, 4(2):1-19. DOI http://dx.doi.org/10.18063/ IJB.v4i2.139.
5. Derakhshanfar S, Mbeleck R, Xu K, et al., 2018, 3D Bioprinting for Biomedical Devices and Tissue Engineering: A Review of Recent Trends and Advances. Bioact Mater, 3(2):144. DOI 10.1016/J.BIOACTMAT.2017.11.008.
6. Murphy C, Kolan K, Li W, et al., 2017, 3D Bioprinting of Stem Cells and Polymer/Bioactive Glass Composite Scaffolds for Tissue Engineering. Int J Bioprinting, 3(1):54- 64. DOI 10.18063/IJB.2017.01.005.
7. Guo SZ, Gosselin F, Guerin N, et al., 2013, Solvent-cast Three- Dimensional Printing of Multifunctional Microsystems. Small, 9(24):4118-22. DOI 10.1002/smll.201300975.
8. Kaur G, Dufour JM, 2012, Cell Lines: Valuable Tools or Useless Artifacts. Spermatogenesis, 2(1):1-5. DOI 10.4161/ spmg.19885.
9. Ong CS, Yesantharao P, Huang CY, et al., 2018, 3D Bioprinting Using Stem Cells. Pediatr Res, 83(1-2):223-31. DOI 10.1038/pr.2017.252.
10. Tasoglu S, Demirci U, 2013, Bioprinting for Stem Cell Research. Trends Biotechnol, 31(1):10-9. DOI 10.1016/j. tibtech.2012.10.005.
11. Uccelli A, Moretta L, Pistoia V, 2008, Mesenchymal Stem Cells in Health and Disease. Nat Rev Immunol, 8(9):726-36. DOI 10.1038/nri2395.
12. D’Andrea F, De Francesco F, Ferraro GA, et al., 2008, Large-scale Production of Human Adipose Tissue from Stem Cells: A New Tool for Regenerative Medicine and Tissue Banking. Tissue Eng Part C Methods, 14(3):233-42. DOI 10.1089/ten. tec.2008.0108.
13. Casteilla L, Dani C, 2006, Adipose Tissue-derived Cells: From Physiology to Regenerative Medicine. Diabetes Metab, 32(5 Pt 1):393-401. DOI DM-11-2006-32-5-1262-3636- 101019-200519820.
14. Wang Y, Yin P, Bian GL, et al., 2017, The Combination of Stem Cells and Tissue Engineering: An Advanced Strategy for Blood Vessels Regeneration and Vascular Disease Treatment. Stem Cell Res Ther, 8(1):194. DOI 10.1186/s13287-017- 0642-y.
15. Apelgren P, Amoroso M, Lindahl A, et al., 2017, Chondrocytes and Stem Cells in 3D-Bioprinted Structures Create Human Cartilage In Vivo. PLoS One, 12(12):e0189428. DOI 10.1371/ journal.pone.0189428.
16. Kim Y, Kang K, Yoon S, et al., 2018, Prolongation of Liver-specific Function for Primary Hepatocytes Maintenance in 3D Printed Architectures. Organogenesis, 14(1):1-12. DOI 10.1080/15476278.2018.1423931.
17. Kolesky DB, Truby RL, Gladman AS, et al., 2014, 3D Bioprinting of Vascularized, Heterogeneous Cell-laden Tissue Constructs. Adv Mater, 26(19):3124-30. DOI 10.1002/ adma.201305506.
18. Sasaki JI, Hashimoto M, Yamaguchi S, et al., 2015, Fabrication of Biomimetic Bone Tissue Using Mesenchymal Stem Cell-derived Three-dimensional Constructs Incorporating Endothelial Cells. PLoS One, 10(6):e0129266. DOI 10.1371/ journal.pone.0129266.
19. Wang X, Tolba E, Schröder HC, et al., 2014, Effect of Bioglass on Growth and Biomineralization of SaOS-2 Cells in Hydrogel after 3D Cell Bioprinting. PLoS One, 9(11):e112497. DOI 10.1371/journal.pone.0112497.
20. Ojansivu M, Rashad A, Ahlinder AE, et al., 2019, Wood-based Nanocellulose and Bioactive Glass Modified Gelatin-alginate Bioinks for 3D Bioprinting of Bone Cells. Biofabrication, 11(3):35010. DOI 10.1088/1758-5090/ab0692.
21. Zhang J, Chen Y, Xu J, et al., 2018, Tissue Engineering Using 3D Printed Nano-bioactive Glass Loaded with NELL1 Gene for Repairing Alveolar Bone Defects. Regen Biomater, 5(4):213-20. DOI 10.1093/rb/rby015.
22. Jung SB, Day DE, 2011, Revolution in Wound Care? Inexpensive, Easy-to-use Cotton Candy-like Glass Fibers Appear to Speed Healing in Initial Venous Stasis Wound Trial. Am Ceram Soc Bull, 90(4):25-9. Available from: https:// www.ceramics.org/ceramic-tech-today/revolution-in-wound-care-inexpensive-easy-to-use-cotton-candy-like-glass-fibers-appear-to-speed-healing-in-initial-venous-stasis-wound-trial. [Last accessed on 2019 Apr 29].
23. Jung S, 2010, Borate Based Bioactive Glass Scaffolds for Hard and Soft Tissue Engineering. Doctoral Dissertations. Available from: http://www.scholarsmine.mst.edu/doctoral_ dissertations/2075. [Last accessed on 2019 Apr 29].
24. Food and Drug Administration, 2016, Department of Health and Human Services. Food and Drug Administration. Available from: https://www.etswoundcare.com. [Last accessed on 2019 Apr 29].
25. Lin Y, Brown RF, Jung SB, et al., 2014, Angiogenic Effects of Borate Glass Microfibers in a Rodent Model. J Biomed Mater Res Part A 102A:4491-9. DOI 10.1002/jbm.a.35120.
26. Karageorgiou V, Kaplan D, 2005, Porosity of 3D Biomaterial Scaffolds and Osteogenesis. Biomaterials, 26(27):5474-91. DOI 10.1016/j.biomaterials.2005.02.002.
27. Luo Y, Lode A, Akkineni AR, et al., 2015, Concentrated Gelatin/Alginate Composites for Fabrication of Predesigned Scaffolds with a Favorable Cell Response by 3D Plotting. RSC Adv, 5(54):43480-8. DOI 10.1039/C5RA04308E.
28. Shi L, Xiong L, Hu Y, et al., 2018, Three-dimensional Printing Alginate/Gelatin Scaffolds as Dermal Substitutes for Skin Tissue Engineering. Polym Eng Sci, 58(10):1782-90. DOI 10.1002/pen.24779.
29. Zhou J, Wang H, Zhao S, et al., 2016, In vivo and in vitro Studies of Borate Based Glass Micro-fibers for Dermal Repairing. Mater Sci Eng C, 60:437-45. DOI 10.1016/J. MSEC.2015.11.068.
30. Earl P, Stoecker W, Jung S, 2018, Clinical Case Studies ETS Wound Care. Available from: https://www.etswoundcare.com/ clinical-case-studies.html. [Last accessed on 2019 Apr 29].
31. Gu Y, Huang W, Rahaman MN, et al., 2013, Bone Regeneration in Rat Calvarial Defects Implanted with Fibrous Scaffolds Composed of a Mixture of Silicate and Borate Bioactive Glasses. Acta Biomater, 9(11):9126-36. DOI 10.1016/j.actbio.2013.06.039.
32. Woodruff MA, Hutmacher DW, 2010, The Return of a Forgotten Polymer Polycaprolactone in the 21st Century. Prog Polym Sci, 35(10):1217-56. DOI 10.1016/j. progpolymsci.2010.04.002.
33. Lee H, Won Koo Y, Yeo M, et al., 2017, Recent Cell Printing Systems for Tissue Engineering. Int J Bioprinting, 3(1):27- 41. DOI 10.18063/IJB.2017.01.004.
34. Rezaei S, Shakibaie M, Kabir-Salmani M, et al., 2016, Improving the Growth Rate of Human Adipose-derived Mesenchymal Stem Cells in Alginate/Gelatin Versus Alginate Hydrogels. Iran J Biotechnol, 14(1):1-8. DOI 10.15171/ ijb.1185.
35. Li Z, Huang S, Liu Y, et al., 2018, Tuning Alginate-gelatin Bioink Properties by Varying Solvent and Their Impact on Stem Cell Behavior. Sci Rep, 8(1):8020. DOI 10.1038/ s41598-018-26407-3.
36. Jeon O, Alsberg E, 2013, Photofunctionalization of Alginate Hydrogels to Promote Adhesion and Proliferation of Human Mesenchymal Stem Cells. Tissue Eng Part A, 19(11- 12):1424-32. DOI 10.1089/ten.TEA.2012.0581.
37. Rowley JA, Madlambayan G, Mooney DJ, 1999, Alginate Hydrogels as Synthetic Extracellular Matrix Materials. Biomaterials, 20(1):45-53. DOI 10.1016/S0142- 9612(98)00107-0.
38. Qazi T H, Hafeez S, Schmidt J, et al., 2017, Comparison of the Effects of 45S5 and 1393 Bioactive Glass Microparticles on hMSC Behavior. J Biomed Mater Res A, 105(10):2772-82. DOI 10.1002/jbm.a.36131.
39. Gholami S, Labbaf S, Houreh AB, et al., 2017, Long Term Effects of Bioactive Glass Particulates on Dental Pulp Stem Cells In Vitro. 3(1):97-103. DOI 10.1515/bglass-2017-0009.
40. Kolan K, Li J, Roberts S, et al., 2018, Near-field Electrospinning of a Polymer/Bioactive Glass Composite to Fabricate 3D Biomimetic Structures. Int J Bioprinting, 5(1):1-6. DOI 10.18063/ijb.v5i1.163.
41. Thyparambil N, Bromet B, Gutgesell L, et al., 2019, Borate Bioactive Glass Triggers Phenotypic Changes in Adipose Stem Cells. Manuscript Submitted for Publication.
42. Patel RG, Purwada A, Cerchietti L, et al., 2014, Microscale Bioadhesive Hydrogel Arrays for Cell Engineering Applications. Cell Mol Bioeng, 7(3):394-408. DOI 10.1007/ s12195-014-0353-8.
43. Xing Q, Yates K, Vogt C, et al., 2015, Increasing Mechanical Strength of Gelatin Hydrogels by Divalent Metal Ion Removal. Sci Rep, 4(1):4706. DOI 10.1038/srep04706.
44. Kargozar S, Baino F, Hamzehlou S, et al., 2018, Bioactive Glasses: Sprouting Angiogenesis in Tissue Engineering. Trends Biotechnol, 36(4):430-44. DOI 10.1016/j. tibtech.2017.12.003.