Collagen-sodium alginate-silk fibroin 3D-printed scaffold loaded with polydatin promotes cartilage regeneration by improving lipid metabolism and cell apoptosis
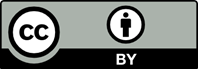
Cartilage defects negatively impact the quality of life of over 500 million people worldwide. 3D-printed scaffolds loaded with polydatin (PD) have shown significant potential in cartilage defect repair. This study aims to investigate their reparative effects and analyze the associated metabolic changes using lipidomics techniques, providing new strategies for treating cartilage defects. Biocompatible 3D-printed scaffolds containing PD were prepared and 30 New Zealand rabbits were divided into three groups (10 rabbits per group) that underwent either sham surgery (Normal group) or surgical creation of a cartilage defect without scaffold filling (Model group) or with the developed scaffold filling (Scaffold group). After three months of intervention, the repair of cartilage defects was evaluated through macroscopic observation, micro-CT, hematoxylin and eosin (H&E) staining, and Safranin O/fast green (SFO/FG) staining. The expression of vascular endothelial growth factor A vascular endothelial growth factor A (VEGFA), Col2a1, and biglycan was detected by immunofluorescence while lipid metabolic profiling analysis was conducted on newly formed cartilage tissue to comprehensively evaluate the scaffold’s mechanism of action. Macroscopic observation, micro-CT, H&E staining, and SFO/FG staining indicated that the repair of cartilage defects in the Scaffold group was significantly better than in the Model group, closely resembling the Normal group. Lipidomics revealed that the Scaffold group modulated 36 metabolites, with a recovery rate of 69.23%, including ceramides (Cers), glycerophospholipids (GPs), and sphingomyelins (SMs). Immunofluorescence analysis showed increased expression of cartilage cell markers Sox9, Col2a1, biglycan, and VEGFA, along with a reduction in cell apoptosis (all p <0.05) after scaffold implantation. These findings collectively suggest that the PD-loaded 3D-printed scaffold promotes cartilage repair by restoring lipid metabolites in cartilage tissue, inhibiting chondrocyte apoptosis, enhancing vascular-related protein expression, and accelerating cartilage collagen matrix remodeling.

- Paul S, Schrobback K, Tran PA, et al. GelMA-glycol chitosan hydrogels for cartilage regeneration: the role of uniaxial mechanical stimulation in enhancing mechanical, adhesive, and biochemical properties. APL Bioeng. 2023;7(3):036114. doi: 10.1063/5.0160472
- Wei W, Ma Y, Yao X, et al. Advanced hydrogels for the repair of cartilage defects and regeneration. Bioact Mater. 2020;6(4):998-1011. doi: 10.1016/j.bioactmat.2020.09.030
- Liu YY, Intini C, Dobricic M, O’Brien FJ, LLorca J, Echeverry-Rendon M. Collagen-based 3D printed poly (glycerol sebacate) composite scaffold with biomimicking mechanical properties for enhanced cartilage defect repair. Int J Biol Macromol. 2024;280(Pt 2):135827. doi: 10.1016/j.ijbiomac.2024.135827
- Peng L, Li H, Deng H, et al. Combination of a human articular cartilage-derived extracellular matrix scaffold and microfracture techniques for cartilage regeneration: a proof of concept in a sheep model. J Orthop Translat. 2023;44: 72-87. doi: 10.1016/j.jot.2023.09.004
- Li H, He Z, Li W, et al. Exploring the mechanism of microfracture in the treatment of porcine full-thickness cartilage defect. Am J Sports Med. 2023;51(4):1033-1046. doi: 10.1177/03635465231153630
- Huang Z, Liu C, Zheng G, et al. Articular cartilage regeneration via induced chondrocyte autophagy by sustained release of leptin inhibitor from thermo-sensitive hydrogel through STAT3/REDD1/mTORC1 cascade. Adv Healthc Mater. 2023;12(30):e2302181. doi: 10.1002/adhm.202302181
- Lee KW, Chung K, Nam DH, Jung M, Kim SH, Kim HG. Decellularized allogeneic cartilage paste with human costal cartilage and crosslinked hyaluronic acid-carboxymethyl cellulose carrier augments microfracture for improved articular cartilage repair. Acta Biomater. 2023;172: 297-308. doi: 10.1016/j.actbio.2023.10.008
- Migliorini F, Maffulli N, Baroncini A, et al. Allograft versus autograft osteochondral transplant for chondral defects of the talus: systematic review and meta-analysis. Am J Sports Med. 2022;50(12):3447-3455. doi: 10.1177/03635465211037349
- Wang AS, Nagelli CV, Lamba A, Saris DBF, Krych AJ, Hevesi M. Minimum 10-year outcomes of matrix-induced autologous chondrocyte implantation in the knee: a systematic review. Am J Sports Med. 2024;52(9):2407-2414. doi: 10.1177/03635465231205309
- Chen R, Pye JS, Li J, Little CB, Li JJ. Multiphasic scaffolds for the repair of osteochondral defects: Outcomes of preclinical studies. Bioact Mater. 2023;27:505-545. doi: 10.1016/j.bioactmat.2023.04.016
- Shaygani H, Mofrad YM, Demneh SMR, Hafezi S, Almasi- Jaf A, Shamloo A. Cartilage and bone injectable hydrogels: a review of injectability methods and treatment strategies for repair in tissue engineering. Int J Biol Macromol. 2024; 282(Pt 2):136689. doi: 10.1016/j.ijbiomac.2024.136689
- Cheah CM, Chua CK, Leong KF, Chua SW. Development of a tissue engineering scaffold structure library for rapid prototyping. Part 1: investigation and classification. Int J Adv Manuf Technol. 2003;21:291-301. doi: 10.1007/s001700300034
- Cheah CM, Chua CK, Leong KF, Chua SW. Development of a tissue engineering scaffold structure library for rapid prototyping. Part 2: parametric library and assembly program. Int J Adv Manuf Technol. 2003;21: 302-312. doi: 10.1007/s001700300035
- Du J, Zhu Z, Liu J, et al. 3D-printed gradient scaffolds for osteochondral defects: current status and perspectives. Int J Bioprint. 2023;9(4):724. doi: 10.18063/ijb.724
- O’Shea DG, Hodgkinson T, Curtin CM, O’Brien FJ. An injectable and 3D printable pro-chondrogenic hyaluronic acid and collagen type II composite hydrogel for the repair of articular cartilage defects. Biofabrication. 2023;16(1): 015007. doi: 10.1088/1758-5090/ad047a
- Du W, Wang Z, Han M, et al. Astragalus polysaccharide-containing 3D-printed scaffold for traumatized skin repair and proteomic study. J Cell Mol Med. 2024;28(16):e70023. doi: 10.1111/jcmm.70023
- Du W, Hu J, Huang X, et al. Feasibility of repairing skin defects by VEGF(165) gene-modified iPS-HFSCs seeded on a 3D printed scaffold containing astragalus polysaccharide. J Cell Mol Med. 2023;27(15):2136-2149. doi: 10.1111/jcmm.17800
- Gong W, Zhang N, Sun X, et al. Cardioprotective effects of polydatin against myocardial injury in HFD/stz and high glucose-induced diabetes via a Caveolin 1-dependent mechanism. Phytomedicine. 2024;135:156055. doi: 10.1016/j.phymed.2024.156055
- Zhang Z, Sun Z, Jia R, et al. Protective effects of polydatin against bone and joint disorders: the in vitro and in vivo evidence so far. Nutr Res Rev. 2024;37(1):96-107. doi: 10.1017/S0954422423000082.
- Bagheri Bavandpouri FS, Azizi A, Abbaszadeh F, et al. Polydatin attenuated neuropathic pain and motor dysfunction following spinal cord injury in rats by employing its anti-inflammatory and antioxidant effects. Front Pharmacol. 2024;15:1452989. doi: 10.3389/fphar.2024.1452989
- Ye Z, Lin J, He C, et al. Polydatin protects against articular cartilage degeneration by regulating autophagy mediated by the AMPK/mTOR signaling pathway. Histol Histopathol. 2024;39(11):1505-1515. doi: 10.14670/HH-18-739
- Tang S, Tang Q, Jin J, et al. Polydatin inhibits the IL-1beta-induced inflammatory response in human osteoarthritic chondrocytes by activating the Nrf2 signaling pathway and ameliorates murine osteoarthritis. Food Funct. 2018;9(3):1701-1712. doi: 10.1039/c7fo01555k
- Zhao Z, Wang Y, Yin B, et al. Defect-adaptive stem-cell-microcarrier construct promotes tissue repair in rabbits with knee cartilage defects. Stem Cell Rev Rep. 2023;19(1):201-212. doi: 10.1007/s12015-022-10421-0
- Ye C, Chen J, Qu Y, et al. Naringin in the repair of knee cartilage injury via the TGF-beta/ALK5/Smad2/3 signal transduction pathway combined with an acellular dermal matrix. J Orthop Translat. 2021;32:1-11. doi: 10.1016/j.jot.2021.06.004
- Liu H, Liu H, Yang Q, Fan Z. LncRNA SNHG1 enhances cartilage regeneration by modulating chondrogenic differentiation and angiogenesis potentials of JBMMSCs via mitochondrial function regulation. Stem Cell Res Ther. 2024;15(1):177. doi: 10.1186/s13287-024-03793-2
- Yang Z, Li H, Tian Y, et al. Biofunctionalized structure and ingredient mimicking scaffolds achieving recruitment and chondrogenesis for staged cartilage regeneration. Front Cell Dev Biol. 2021;9:655440. doi: 10.3389/fcell.2021.655440
- Hu G, Liang Z, Fan Z, et al. Construction of 3D-bioprinted cartilage-mimicking substitute based on photo-crosslinkable Wharton’s jelly bioinks for full-thickness articular cartilage defect repair. Mater Today Bio. 2023;21:100695. doi: 10.1016/j.mtbio.2023.100695
- Kim YB, Lee H, Kim GH. Strategy to achieve highly porous/ biocompatible macroscale cell blocks, using a collagen/ genipin-bioink and an optimal 3D printing process. ACS Appl Mater Interfaces. 2016;8(47):32230-32240. doi: 10.1021/acsami.6b11669
- Zhou Z, Cui J, Wu S, Geng Z, Su J. Silk fibroin-based biomaterials for cartilage/osteochondral repair. Theranostics. 2022;12(11):5103-5124. doi: 10.7150/thno.74548
- Fang Z, Liu G, Wang B, et al. An injectable self-healing alginate hydrogel with desirable mechanical and degradation properties for enhancing osteochondral regeneration. Carbohydr Polym. 2024;343:122424. doi: 10.1016/j.carbpol.2024.122424
- Fang K, Li P, Zhang B, et al. Insights on updates in sodium alginate/MXenes composites as the designer matrix for various applications: a review. Int J Biol Macromol. 2024;269:132032. doi: 10.1016/j.ijbiomac.2024.132032
- Sanchez-Ballester NM, Bataille B, Soulairol I. Sodium alginate and alginic acid as pharmaceutical excipients for tablet formulation: structure-function relationship. Carbohydr Polym. 2021;270:118399. doi: 10.1016/j.carbpol.2021.118399
- Chaurasia B, Summers SA. Ceramides in metabolism: key lipotoxic players. Annu Rev Physiol. 2021;83:303-330. doi: 10.1146/annurev-physiol-031620-093815
- Hu L, Luo D, Zhang H, He L. Polydatin inhibits IL-1β- mediated chondrocyte inflammation and ameliorates cartilage degradation: involvement of the NF-κB and Wnt/ β-catenin pathways. Tissue Cell. 2022;78:101865. doi: 10.1016/j.tice.2022.101865
- Alizadeh J, da Silva Rosa SC, Weng X, et al. Ceramides and ceramide synthases in cancer: focus on apoptosis and autophagy. Eur J Cell Biol. 2023;102(3):151337. doi: 10.1016/j.ejcb.2023.151337
- Armistead J, Höpfl S, Goldhausen P, et al. A sphingolipid rheostat controls apoptosis versus apical cell extrusion as alternative tumour-suppressive mechanisms. Cell Death Dis. 2024;15(10):746. doi: 10.1038/s41419-024-07134-2
- Peche VS, Pietka TA, Jacome-Sosa M, et al. Endothelial cell CD36 regulates membrane ceramide formation, exosome fatty acid transfer and circulating fatty acid levels. Nat Commun. 2023;14(1):4029. doi: 10.1038/s41467-023-39752-3
- Bao HN, Yin J, Wang LY, et al. Aberrant accumulation of ceramides in mitochondria triggers cell death by inducing autophagy in Arabidopsis. J Exp Bot. 2024;75(5): 1314-1330. doi: 10.1093/jxb/erad456
- Tu H, YL Li. Inflammation balance in skeletal muscle damage and repair. Front Immunol. 2023;14:1133355. doi: 10.3389/fimmu.2023.1133355
- Adada M, Luberto C, Canals D. Inhibitors of the sphingomyelin cycle: sphingomyelin synthases and sphingomyelinases. Chem Phys Lipids. 2016;197:45-59. doi: 10.1016/j.chemphyslip.2015.07.008
- Haseeb A, Kc R, Angelozzi M, et al. SOX9 keeps growth plates and articular cartilage healthy by inhibiting chondrocyte dedifferentiation/osteoblastic redifferentiation. Proc Natl Acad Sci USA. 2021;118(8):e2019152118. doi: 10.1073/pnas.2019152118
- Lefebvre V, Angelozzi M, Haseeb A. SOX9 in cartilage development and disease. Curr Opin Cell Biol. 2019; 61:39-47. doi: 10.1016/j.ceb.2019.07.008
- Song H, Park K. Regulation and function of SOX9 during cartilage development and regeneration. Semin Cancer Biol. 2020;67(Pt 1):12-23. doi: 10.1016/j.semcancer.2020.04.008
- Gilbert SJ, Blain EJ, Duance VC, Mason DJ. Sphingomyelinase decreases type II collagen expression in bovine articular cartilage chondrocytes via the ERK signaling pathway. Arthritis Rheum. 2008;58(1):209-220. doi: 10.1002/art.23172
- Piacentino ML, Fasse AJ, Camacho-Avila A, Grabylnikov I, Bronner ME. SMPD3 expression is spatially regulated in the developing embryo by SOXE factors. Dev Biol. 2024; 506:31-41. doi: 10.1016/j.ydbio.2023.11.011
- Lucki NC and MB Sewer. Nuclear sphingolipid metabolism. Annu Rev Physiol. 2012;74:131-51. doi: 10.1146/annurev-physiol-020911-153321
- Xu Z, Xu B, Lundström SL, et al. A subset of type-II collagen-binding antibodies prevents experimental arthritis by inhibiting FCGR3 signaling in neutrophils. Nat Commun. 2023;14(1):5949. doi: 10.1038/s41467-023-41561-7
- Zeltz C, Gullberg D. The integrin-collagen connection – a glue for tissue repair? J Cell Sci. 2016;129(4):653-664. doi: 10.1242/jcs.180992
- Lauer JC, Selig M, Hart ML, Kurz B, Rolauffs B. Articular chondrocyte phenotype regulation through the cytoskeleton and the signaling processes that originate from or converge on the cytoskeleton: towards a novel understanding of the intersection between actin dynamics and chondrogenic function. Int J Mol Sci. 2021;22(6):3279. doi: 10.3390/ijms22063279
- Renard E, Porée B, Chadjichristos C, et al. Sox9/Sox6 and Sp1 are involved in the insulin-like growth factor-I-mediated upregulation of human type II collagen gene expression in articular chondrocytes. J Mol Med (Berl). 2012;90(6):649-666. doi: 10.1007/s00109-011-0842-3
- Simental-Mendía M, Lara-Arias J, Álvarez-Lozano E, et al. Cotransfected human chondrocytes: over-expression of IGF-I and SOX9 enhances the synthesis of cartilage matrix components collagen-II and glycosaminoglycans. Braz J Med Biol Res. 2015;48(12):1063-1070. doi: 10.1590/1414-431X20154732