Polydopamine-modified 3D-printed polycaprolactone scaffolds for promoting bone regeneration
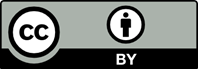
Polycaprolactone (PCL) is one of the most widely used three-dimensional (3D) printing materials with excellent biocompatibility and mechanical properties. However, its hydrophobic nature hinders cell adhesion and proliferation. Polydopamine (PDA) has been shown to promote proliferation and induce osteogenic differentiation of bone marrow mesenchymal stem cells (BMSCs) on polymer surfaces. Despite this, the impact of varying PDA coating thicknesses on the osteogenic differentiation of BMSCs has been minimally explored. In this paper, PCL scaffolds were fabricated using 3D printing technology, and PDA-coated PCL scaffolds (PDA-PCL-0, PDA-PCL-3, PDA-PCL-6, PDA-PCL-24) were prepared by immersing the scaffolds in an aqueous dopamine solution for fixed time points (0, 3, 6, 24 h) under constant shaking. The scaffolds were characterized and subjected to physicochemical performance tests to evaluate their effects on BMSC proliferation, adhesion, and osteogenic differentiation. The results showed that PDA-PCL-6 scaffolds exhibited significant immunomodulatory properties, promoting BMSC proliferation, adhesion, and osteogenic differentiation more effectively than the other groups. In vivo validation experiments, including micro-computed tomography, hematoxylin and eosin staining, Masson staining, and immunohistochemical analysis of bone morphologenetic protein 2 (BMP-2) and type I collagen (COL-I), confirmed that PDA-PCL-6 scaffolds significantly enhanced bone regeneration, histocompatibility, and hemocompatibility compared to uncoated scaffolds at 1, 2, and 3 months postoperation. In conclusion, our results indicate that a PDA coating obtained through 6-h immersion significantly enhances the biocompatibility and osteoinductive properties of PCL scaffolds, providing a promising strategy for bone defect repair.

- Nauth A, McKee MD, Einhorn TA, Watson JT, Li R, Schemitsch EH. Managing bone defects. J Orthop Trauma. 2011;25(8):462-466. doi: 10.1097/BOT.0b013e318224caf0
- Schemitsch EH. Size matters: defining critical in bone defect size! J Orthop Trauma. 2017;31(Suppl 5):S20-S22. doi: 10.1097/BOT.0000000000000978
- Khodakaram-Tafti A, Mehrabani D, Shaterzadeh-Yazdi H, Zamiri B, Omidi M. Tissue engineering in maxillary bone defects. World J Plast Surg. 2018;7(1):3-11.
- Dewey MJ, Milner DJ, Weisgerber D, et al. Repair of critical-size porcine craniofacial bone defects using a collagen-polycaprolactone composite biomaterial. Biofabrication. 2021;14(1):1-24. doi: 10.1088/1758-5090/ac30d5
- van der Heide D, Cidonio G, Stoddart MJ, D’Este M. 3D printing of inorganic-biopolymer composites for bone regeneration. Biofabrication. 2022;14(4):1-22. doi: 10.1088/1758-5090/ac8cb2
- Turnbull G, Clarke J, Picard F, et al. 3D bioactive composite scaffolds for bone tissue engineering. Bioact Mater. 2017;3(3):278-314. doi: 10.1016/j.bioactmat.2017.10.001
- Gharibshahian M, Salehi M, Beheshtizadeh N, et al. Recent advances on 3D-printed PCL-based composite scaffolds for bone tissue engineering. Front Bioeng Biotechnol. 2023;11:1168504. doi: 10.3389/fbioe.2023.1168504
- Nobles KP, Janorkar AV, Williamson RS. Surface modifications to enhance osseointegration-Resulting material properties and biological responses. J Biomed Mater Res B Appl Biomater. 2021;109(11):1909-1923. doi: 10.1002/jbm.b.34835
- Gharibshahian M, Salehi M, Kamalabadi-Farahani M, Alizadeh M. Magnesium-oxide-enhanced bone regeneration: 3D-printing of gelatin-coated composite scaffolds with sustained Rosuvastatin release. Int J Biol Macromol. 2024;266(Pt 1):130995. doi: 10.1016/j.ijbiomac.2024.130995
- Kikionis S, Ioannou E, Aggelidou E, et al. The marine polysaccharide ulvan confers potent osteoinductive capacity to PCL-based scaffolds for bone tissue engineering applications. Int J Mol Sci. 2021;22(6):3086. doi: 10.3390/ijms22063086
- Wang B, Zeng Y, Liu S, et al. ZIF-8 induced hydroxyapatite-like crystals enabled superior osteogenic ability of MEW printing PCL scaffolds. J Nanobiotechnol. 2023; 21(1):264. doi: 10.1186/s12951-023-02007-w
- Ha YM, Kim YN, Jung YC. Rapid and local self-healing ability of polyurethane nanocomposites using photothermal polydopamine-coated graphene oxide triggered by near-infrared laser. Polymers. 2021;13(8):1274. doi: 10.3390/polym13081274
- Yan J, Wu R, Liao S, Jiang M, Qian Y. Applications of polydopamine-modified scaffolds in the peripheral nerve tissue engineering. Front Bioeng Biotechnol. 2020;8:590998. doi: 10.3389/fbioe.2020.590998
- Rezk AI, Ramachandra Kurup Sasikala A, Nejad AG, et al. Strategic design of a mussel-inspired in situ reduced Ag/Au-nanoparticle coated magnesium alloy for enhanced viability, antibacterial property and decelerated corrosion rates for degradable implant applications. Sci Rep. 2019;9(1):117. doi: 10.1038/s41598-018-36545-3
- Teixeira BN, Aprile P, Mendonça RH, Kelly DJ, Thiré RM da SM. Evaluation of bone marrow stem cell response to PLA scaffolds manufactured by 3D printing and coated with polydopamine and type I collagen. J Biomed Mater Res B, Appl Biomater. 2019;107(1):37-49. doi: 10.1002/jbm.b.34093
- Lin Y, Ou Y, Xu M, Chen J. Enhancing bone regeneration with bionic hydrolysis and biomimetic polydopamine coating on 3D-printed PCL scaffolds: a comparative study. Mater Today Commun. 2023;37:107262. doi: 10.1016/j.mtcomm.2023.107262
- Xu Z, Wang N, Liu P, et al. Poly(Dopamine) coating on 3D-printed poly-lactic-co-glycolic acid/β-tricalcium phosphate scaffolds for bone tissue engineering. Molecules. 2019;24(23):4397. doi: 10.3390/molecules24234397
- Lee H, Dellatore SM, Miller WM, Messersmith PB. Mussel-inspired surface chemistry for multifunctional coatings. Science. 2007;318(5849):426-430. doi: 10.1126/science.1147241
- Li Y, Yang L, Hou Y, et al. Polydopamine-mediated graphene oxide and nanohydroxyapatite-incorporated conductive scaffold with an immunomodulatory ability accelerates periodontal bone regeneration in diabetes. Bioact Mater. 2022;18:213-227. doi: 10.1016/j.bioactmat.2022.03.021
- Zhou S, Liu S, Wang Y, et al. Advances in the study of bionic mineralized collagen, PLGA, magnesium ionomer materials, and their composite scaffolds for bone defect treatment. J Funct Biomater. 2023;14(8):406. doi: 10.3390/jfb14080406
- Guo R, Zhang R, Liu S, et al. Biomimetic, biodegradable and osteoinductive treated dentin matrix/α-calcium sulphate hemihydrate composite material for bone tissue engineering. Regener Biomater. 2023;10:rbad061. doi: 10.1093/rb/rbad061
- Wang S, Gu R, Wang F, et al. 3D-Printed PCL/Zn scaffolds for bone regeneration with a dose-dependent effect on osteogenesis and osteoclastogenesis. Mater Today Bio. 2022;13:100202. doi: 10.1016/j.mtbio.2021.100202
- Tang A, Ji J, Li J, et al. Nanocellulose/PEGDA aerogels with tunable poisson’s ratio fabricated by stereolithography for mouse bone marrow mesenchymal stem cell culture. Nanomaterials (Basel). 2021;11(3):603. doi: 10.3390/nano11030603
- Wu Y, Liu J, Kang L, et al. An overview of 3D printed metal implants in orthopedic applications: present and future perspectives. Heliyon. 2023;9(7):e17718. doi: 10.1016/j.heliyon.2023.e17718
- Dong J, Ding H, Wang Q, Wang L. A 3D-printed scaffold for repairing bone defects. Polymers. 2024;16(5):706. doi: 10.3390/polym16050706
- Zhao Q, Gao S. Poly (butylene succinate)/silicon nitride nanocomposite with optimized physicochemical properties, biocompatibility, degradability, and osteogenesis for cranial bone repair. J Funct Biomater. 2022;13(4):231. doi: 10.3390/jfb13040231
- Jia L, Han F, Wang H, et al. Polydopamine-assisted surface modification for orthopaedic implants. J Orthop Transl. 2019;17:82-95. doi: 10.1016/j.jot.2019.04.001
- Xiao L, Li Y, Geng R, et al. Polymer composite microspheres loading 177Lu radionuclide for interventional radioembolization therapy and real-time SPECT imaging of hepatic cancer. Biomater Res. 2023;27(1):110. doi: 10.1186/s40824-023-00455-x
- Mahnavi A, Shahriari-Khalaji M, Hosseinpour B, et al. Evaluation of cell adhesion and osteoconductivity in bone substitutes modified by polydopamine. Front Bioeng Biotechnol. 2022;10:1057699. doi: 10.3389/fbioe.2022.1057699
- Du J, Zhou Y, Bao X, et al. Surface polydopamine modification of bone defect repair materials: Characteristics and applications. Front Bioeng Biotechnol. 2022; 10:974533. doi: 10.3389/fbioe.2022.974533
- Caihong T, Fusheng M, Baoyong L, et al. Research status of the surface modification method based on polydopamine [in Chinese]. Chem Bull 2015;78: 983-990. doi: 10.14159/j.cnki.0441-3776.2015.11.003
- Huang S, Liang N, Hu Y, Zhou X, Abidi N. Polydopamine-assisted surface modification for bone biosubstitutes. BioMed Res Int. 2016;2016:2389895. doi: 10.1155/2016/2389895
- Li H, Zhao T, Cao F, et al. Integrated bioactive scaffold with aptamer-targeted stem cell recruitment and growth factor-induced pro-differentiation effects for anisotropic meniscal regeneration. Bioeng Transl Med. 2022; “7(3):e10302. doi: 10.1002/btm2.10302
- Chang B, Liu X. Osteon: Structure, Turnover, and Regeneration. Tissue Eng Part B Rev. 2022;28(2):261-278. doi: 10.1089/ten.TEB.2020.0322
- V K AD, Ray S, Arora U, Mitra S, Sionkowska A, Jaiswal AK. Dual drug delivery platforms for bone tissue engineering. Front Bioeng Biotechnol. 2022;10:969843. doi: 10.3389/fbioe.2022.969843
- Ma YX, Jiao K, Wan QQ, et al. Silicified collagen scaffold induces semaphorin 3A secretion by sensory nerves to improve in-situ bone regeneration. Bioact Mater. 2022;9:475-490. doi: 10.1016/j.bioactmat.2021.07.016
- Chen X, Yang W, Zhang J, Zhang L, Shen H, Shi D. Alkalinity triggered the degradation of polydopamine nanoparticles. Polym Bull. 2021;78(8):4439-4452. doi: 10.1007/s00289-020-03312-2
- Wang CX, Ge XY, Wang MY, Ma T, Zhang Y, Lin Y. Dopamine D1 receptor-mediated activation of the ERK signaling pathway is involved in the osteogenic differentiation of bone mesenchymal stem cells. Stem Cell Res Ther. 2020;11(1):12. doi: 10.1186/s13287-019-1529-x
- Xia S, Liu D, Jiang K, et al. Photothermal driven BMSCs osteogenesis and M2 macrophage polarization on polydopamine-coated Ti3C2 nanosheets/poly(vinylidene fluoride trifluoroethylene) nanocomposite coatings. Mater Today Bio. 2024;27:101156. doi: 10.1016/j.mtbio.2024.101156
- Sun H, Feng Y, Tu S, et al. Dopamine promotes osteogenic differentiation of PDLSCs by activating DRD1 and DRD2 during orthodontic tooth movement via ERK1/2 signaling pathway. Regen Ther. 2024;27:268-278. doi: 10.1016/j.reth.2024.03.025
- Pei D, Zeng Z, Geng Z, et al. Modulation of macrophage polarization by secondary cross-linked hyaluronan-dopamine hydrogels. Int J Biol Macromol. 2024;270:132417. doi: 10.1016/j.ijbiomac.2024.132417
- Donkiewicz P, Benz K, Kloss-Brandstätter A, Jackowski J. Survival rates of dental implants in autogenous and allogeneic bone blocks: a systematic review. Medicina (Kaunas). 2021;57(12):1388. doi: 10.3390/medicina57121388
- Li X, Zhou Q, Wu Y, et al. Enhanced bone regenerative properties of calcium phosphate ceramic granules in rabbit posterolateral spinal fusion through a reduction of grain size. Bioact Mater. 2022;11:90-106. doi: 10.1016/j.bioactmat.2021.10.006