Biomimetically hierarchical scaffolds drive critical-sized osteochondral tissue regeneration
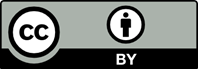
This study presents a pioneering approach utilizing hierarchically functionalized scaffolds to foster anisotropic osteochondral tissue regeneration, leveraging the integration of distinct yet interconnected layers. We developed 3D-printed polydopamine-modified polycaprolactone (PCL) scaffolds, which were subsequently covered with a layer of electrospun PCL-gelatin fibers, and then functionalized with gelatin-bone morphogenetic protein-2 (BMP-2) following oxygen plasma surface treatments, creating a hierarchically organized multi-phasic architecture. This interconnected porous microstructure enabled controllable degradation while maintaining mechanical integrity and hydroxyapatite mineralization. In vitro assessments demonstrated that the scaffolds provided superior support for rat bone marrow mesenchymal stem cells, marked by their enhanced adhesion, viability, and proliferation. Increased alkaline phosphatase activity and osteocalcin expression over 14 days indicated that the scaffolds enhanced osteogenic performance, likely due to BMP-2 interaction with serum proteins, as supported by simulation studies, augmenting growth factor bioavailability. In vivo investigations in rabbit critical- sized osteochondral defects at 4- and 12-week post-implantation demonstrated that the multi-phasic scaffolds notably promoted secretion of type I and II collagen, neo-tissue formation, and integration with surrounding tissue, with significant results observed at 12 weeks. These findings indicate the potential of multi-phasic scaffolds for osteochondral tissue regeneration.

- Yue S, Zhai G, Zhao S, et al. The biphasic role of the infrapatellar fat pad in osteoarthritis. Biomed Pharmacother. 2024;179:117364. doi: 10.1016/j.biopha.2024.117364
- Fang J, Liao J, Zhong C, Lu X, Ren F. High-strength, biomimetic functional chitosan-based hydrogels for full-thickness osteochondral defect repair. ACS Biomater Sci Eng. 2022;8(10):4449-4461. doi: 10.1021/acsbiomaterials.2c00187
- Liu J, Li L, Suo H, Yan M, Yin J, Fu J. 3D printing of biomimetic multi-layered GelMA/nHA scaffold for osteochondral defect repair. Mater Des. 2019;171:107708.doi: 10.1016/j.matdes.2019.107708
- Pagan E, Stefanek E, Seyfoori A, et al. A handheld bioprinter for multi-material printing of complex constructs. Biofabrication. 2023;15(3):035012. doi: 10.1088/1758-5090/acc42c
- He M, Li L, Liu Y, et al. Decellularized extracellular matrix coupled with polycaprolactone/laponite to construct a biomimetic barrier membrane for bone defect repair. Int J Biol Macromol. 2024;276:133775. doi: 10.1016/j.ijbiomac.2024.133775
- Ghalandari B, Yu Y, Ghorbani F, et al. Polydopamine nanospheres coated with bovine serum albumin permit enhanced cell differentiation: fundamental mechanism and practical application for protein coating formation. Nanoscale. 2021;13(47):20098-20110. doi: 10.1039/D1NR07469E
- Ghorbani F, Ghalandari B, Khajehmohammadi M, et al. Photo-cross-linkable hyaluronic acid bioinks for bone and cartilage tissue engineering applications. Int Mater Rev. 2023;68(7):901-942. doi: 10.1080/09506608.2023.2167559
- Yakufu M, Wang Z, Li C, et al. Carbene-mediated gelatin and hyaluronic acid hydrogel paints with ultra adhesive ability for arthroscopic cartilage repair. Int J Biol Macromol. 2024;273:133122. doi: 10.1016/j.ijbiomac.2024.133122
- Gu Z, Wang J, Fu Y, et al. Smart biomaterials for articular cartilage repair and regeneration. Adv Funct Mater. 2023;33(10):2212561. doi: 10.1002/adfm.202212561
- Lee SJ, Nowicki M, Harris B, Zhang LG. Fabrication of a highly aligned neural scaffold via a table top stereolithography 3D printing and electrospinning. Tissue Eng Part A. 2017;23(11-12):491-502. doi: 10.1089/ten.tea.2016.0353
- Zarei M, Shabani Dargah M, Hasanzadeh Azar M, et al. Enhanced bone tissue regeneration using a 3D-printed poly(lactic acid)/Ti6Al4V composite scaffold with plasma treatment modification. Sci Rep. 2023;13(1):3139. doi: 10.1038/s41598-023-30300-z
- Teimouri R, Abnous K, Taghdisi SM, Ramezani M, Alibolandi M. Surface modifications of scaffolds for bone regeneration. J Mater Res Technol. 2023;24:7938-7973. doi: 10.1016/j.jmrt.2023.05.076
- Ghorbani F, Kim M, Ghalandari B, et al. Architecture of β-lactoglobulin coating modulates bioinspired alginate dialdehyde-gelatine/polydopamine scaffolds for subchondral bone regeneration. Acta Biomater. 2024;181:188-201. doi: 10.1016/j.actbio.2024.04.028
- Lin S, Maekawa H, Moeinzadeh S, et al. An osteoinductive and biodegradable intramedullary implant accelerates bone healing and mitigates complications of bone transport in male rats. Nat Commun. 2023;14(1):4455. doi: 10.1038/s41467-023-40149-5
- Kim P, Park J, Lee DJ, et al. Mast4 determines the cell fate of MSCs for bone and cartilage development. Nat Commun. 2022;13(1):3960. doi: 10.1038/s41467-022-31697-3
- Yang X, Tian S, Fan L, et al. Integrated regulation of chondrogenic differentiation in mesenchymal stem cells and differentiation of cancer cells. Cancer Cell Int. 2022;22(1):169. doi: 10.1186/s12935-022-02598-8
- Nokoorani YD, Shamloo A, Bahadoran M, Moravvej H. Fabrication and characterization of scaffolds containing different amounts of allantoin for skin tissue engineering. Sci Rep. 2021;11(1):16164. doi: 10.1038/s41598-021-95763-4
- Montes A, Valor D, Delgado L, Pereyra C, Martínez de la Ossa E. An attempt to optimize supercritical CO2 polyaniline-polycaprolactone foaming processes to produce tissue engineering scaffolds. Polymers (Basel). 2022;14(3):488. doi: 10.3390/polym14030488
- Cai Q. Enzymatic degradation behavior and mechanism of Poly(lactide-co-glycolide) foams by trypsin. Biomaterials. 2003;24(4):629-638. doi: 10.1016/S0142-9612(02)00377-0
- Yan Y, Tao H, He J, Huang SY. The HDOCK server for integrated protein–protein docking. Nat Protoc. 2020;15(5):1829-1852. doi: 10.1038/s41596-020-0312-x
- Yan Y, Zhang D, Zhou P, Li B, Huang SY. HDOCK: a web server for protein–protein and protein–DNA/RNA docking based on a hybrid strategy. Nucleic Acids Res. 2017;45(W1):W365-W373. doi: 10.1093/nar/gkx407
- Protein data bank, www.rcsb.org. www.rcsb.org
- Naghshineh A, Dadras A, Ghalandari B, et al. Safranal as a novel anti-tubulin binding agent with potential use in cancer therapy: an in vitro study. Chem Biol Interact. 2015;238:151-160. doi: 10.1016/j.cbi.2015.06.023
- Micalizzi S, Russo L, Giacomelli C, et al. Multimaterial and multiscale scaffold for engineering enthesis organ. Int J Bioprinting. 2023;9(5):763. doi: 10.18063/ijb.763
- Criscenti G, Longoni A, Di Luca A, et al. Triphasic scaffolds for the regeneration of the bone–ligament interface. Biofabrication. 2016;8(1):015009. doi: 10.1088/1758-5090/8/1/015009
- Song Y, Zhang Y, Qu Q, et al. Biomaterials based on hyaluronic acid, collagen and peptides for three-dimensional cell culture and their application in stem cell differentiation. Int J Biol Macromol. 2023;226:14-36. doi: 10.1016/j.ijbiomac.2022.11.213
- Harati J, Tao X, Shahsavarani H, et al. Polydopamine-mediated protein adsorption alters the epigenetic status and differentiation of primary human adipose-derived stem cells (hASCs). Front Bioeng Biotechnol. 2022;10:934179. doi: 10.3389/fbioe.2022.934179
- Zhang Y, Sun N, Zhu M, et al. The contribution of pore size and porosity of 3D printed porous titanium scaffolds to osteogenesis. Biomater Adv. 2022;133:112651. doi: 10.1016/j.msec.2022.112651
- Maji K, Pramanik K. Electrospun scaffold for bone regeneration. Int J Polym Mater Polym Biomater. 2022;71(11):842-857. doi: 10.1080/00914037.2021.1915784
- Escobar Jaramillo M, Covarrubias C, Patiño González E, Ossa Orozco CP. Optimization by mixture design of chitosan/multi-phase calcium phosphate/BMP-2 biomimetic scaffolds for bone tissue engineering. J Mech Behav Biomed Mater. 2024;152:106423. doi: 10.1016/j.jmbbm.2024.106423
- Alfieri ML, Weil T, Ng DYW, Ball V. Polydopamine at biological interfaces. Adv Colloid Interface Sci. 2022;305:102689. doi: 10.1016/j.cis.2022.102689
- Kim YJ, Kang IK, Huh MW, Yoon SC. Surface characterization and in vitro blood compatibility of poly(ethylene terephthalate) immobilized with insulin and/or heparin using plasma glow discharge. Biomaterials. 2000;21(2):121-130. doi: 10.1016/S0142-9612(99)00137-4
- Yoshinyari M, Hakawa T, Matsuzaka K, et al. Oxygen plasma surface modification enhances immobilization of simvastatin acid. Biomed Res. 2006;27(1):29-36. doi: 10.2220/biomedres.27.29
- Adipurnama I, Yang MC, Ciach T, Butruk-Raszeja B. Surface modification and endothelialization of polyurethane for vascular tissue engineering applications: a review. Biomater Sci. 2017;5(1):22-37. doi: 10.1039/C6BM00618C
- Pohl TLM, Schwab EH, Cavalcanti-Adam EA. Covalent binding of BMP-2 on surfaces using a self-assembled monolayer approach. J Vis Exp. 2013;(78):e50842. doi: 10.3791/50842
- Oliveira JM, Ribeiro VP, Reis RL. Advances on gradient scaffolds for osteochondral tissue engineering. Prog Biomed Eng. 2021;3(3):033001. doi: 10.1088/2516-1091/abfc2c
- Ginebra MP. Cements as bone repair materials. In: Bone Repair Biomaterials. Elsevier; 2009:271-308. doi: 10.1533/9781845696610.2.271
- Auyson K, Koomsap P, Chanthakulchan A, Supaphol P. Investigation of applying electrospinning in fused deposition modeling for scaffold fabrication. In: High Value Manufacturing: Advanced Research in Virtual and Rapid Prototyping. CRC Press; 2013:149-154. doi: 10.1201/b15961-28
- Kim G, Son J, Park S, Kim W. Hybrid process for fabricating 3D hierarchical scaffolds combining rapid prototyping and electrospinning. Macromol Rapid Commun. 2008;29(19):1577-1581. doi: 10.1002/marc.200800277
- Khatiwala CB, Peyton SR, Metzke M, Putnam AJ. The regulation of osteogenesis by ECM rigidity in MC3T3-E1 cells requires MAPK activation. J Cell Physiol. 2007;211(3):661-672. doi: 10.1002/jcp.20974
- Kim K, Yeatts A, Dean D, Fisher JP. Stereolithographic bone scaffold design parameters: osteogenic differentiation and signal expression. Tissue Eng Part B Rev. 2010;16(5):523-539. doi: 10.1089/ten.teb.2010.0171
- Lee SJ, Heo DN, Park JS, et al. Characterization and preparation of bio-tubular scaffolds for fabricating artificial vascular grafts by combining electrospinning and a 3D printing system. Phys Chem Chem Phys. 2015;17(5):2996-2999. doi: 10.1039/C4CP04801F
- Alfieri ML, Panzella L, Napolitano A. Multifunctional coatings hinging on the catechol/amine interplay. Eur J Org Chem. 2024;27(1):e202301002. doi: 10.1002/ejoc.202301002
- Ebrahimi Z, Irani S, Ardeshirylajimi A, Seyedjafari E. Enhanced osteogenic differentiation of stem cells by 3D printed PCL scaffolds coated with collagen and hydroxyapatite. Sci Rep. 2022;12(1):12359. doi: 10.1038/s41598-022-15602-y
- Yang Z, Xi Y, Bai J, et al. Covalent grafting of hyperbranched poly-L-lysine on Ti-based implants achieves dual functions of antibacteria and promoted osteointegration in vivo. Biomaterials. 2021;269:120534. doi: 10.1016/j.biomaterials.2020.120534
- Buck E, Lee S, Stone LS, Cerruti M. Protein adsorption on surfaces functionalized with COOH groups promotes anti-inflammatory macrophage responses. ACS Appl Mater Interfaces. 2021;13(6):7021-7036. doi: 10.1021/acsami.0c16509
- Marsell R, Einhorn TA. The biology of fracture healing. Injury. 2011;42(6):551-555. doi: 10.1016/j.injury.2011.03.031
- Behrens P. Matrixgekoppelte Mikrofrakturierung. Arthroskopie. 2005;18(3):193-197. doi: 10.1007/s00142-005-0316-0
- Hettiaratchi MH, Krishnan L, Rouse T, Chou C, McDevitt TC, Guldberg RE. Heparin-mediated delivery of bone morphogenetic protein-2 improves spatial localization of bone regeneration. Sci Adv. 2020;6(1):aay1240. doi: 10.1126/sciadv.aay1240
- Franco RAG, McKenna E, Shajib MS, et al. Microtissue culture provides clarity on the relative chondrogenic and hypertrophic response of bone-marrow-derived stromal cells to TGF-β1, BMP-2, and GDF-5. Cells. 2023;13(1):37. doi: 10.3390/cells13010037
- Tonda-Turo C, Gentile P, Saracino S, et al. Comparative analysis of gelatin scaffolds crosslinked by genipin and silane coupling agent. Int J Biol Macromol. 2011;49(4):700-706. doi: 10.1016/j.ijbiomac.2011.07.002
- Kim BR, Nguyen TBL, Min YK, Lee BT. In vitro and in vivo studies of BMP-2-loaded PCL–gelatin–BCP electrospun scaffolds. Tissue Eng Part A. 2014;20(23-24):3279-3289. doi: 10.1089/ten.tea.2014.0081
- Sy KHS, Ho LWC, Lau WCY, Ko H, Choi CHJ. Morphological diversity, protein adsorption, and cellular uptake of polydopamine-coated gold nanoparticles. Langmuir. 2018;34(46):14033-14045. doi: 10.1021/acs.langmuir.8b02572
- Firoozi N, Kang Y. Immobilization of FGF on poly(xylitol dodecanedioic Acid) polymer for tissue regeneration. Sci Rep. 2020;10(1):10419. doi: 10.1038/s41598-020-67261-6
- Ghosh S, Scott AK, Seelbinder B, et al. Dedifferentiation alters chondrocyte nuclear mechanics during in vitro culture and expansion. Biophys J. 2022;121(1):131-141. doi: 10.1016/j.bpj.2021.11.018
- Baek JW, Kim KS, Park H, Kim BS. Marine plankton exoskeletone-derived hydroxyapatite/polycaprolactone composite 3D scaffold for bone tissue engineering. Biomater Sci. 2022;10(24):7055-7066. doi: 10.1039/D2BM00875K
- Lee J, Lee S, Huh SJ, Kang B, Shin H. Directed regeneration of osteochondral tissue by hierarchical assembly of spatially organized composite spheroids. Adv Sci. 2022;9(3): 2103525. doi: 10.1002/advs.202103525
- Qin C, Ma J, Chen L, et al. 3D bioprinting of multicellular scaffolds for osteochondral regeneration. Mater Today. 2021;49:68-84. doi: 10.1016/j.mattod.2021.04.016
- Wang Z, Le H, Wang Y, et al. Instructive cartilage regeneration modalities with advanced therapeutic implantations under abnormal conditions. Bioact Mater. 2022;11:317-338. doi: 10.1016/j.bioactmat.2021.10.002
- Yang T, Tamaddon M, Jiang L, et al. Bilayered scaffold with 3D printed stiff subchondral bony compartment to provide constant mechanical support for long-term cartilage regeneration. J Orthop Transl. 2021;30:112-121. doi: 10.1016/j.jot.2021.09.001