Evaluation of 3D-bioprinted skin scaffolds in mice along with gold nanoparticle exposure
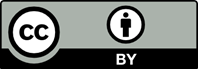
The progress in nanomedicine has sparked increasing concerns regarding its applications with biocompatible materials. Here, we assessed and optimized a three-dimensional (3D) bioprinting technique by testing various printing parameters with multiple cell types. Cell-laden scaffolds were designed, cultivated, imaged, and transplanted onto the dorsal skin of nude mice. The structure of bioprinted scaffolds retained its shape and dimensions with no cell migration between layers. Moreover, gold nanoparticles (GNPs) were intravenously administered to transplanted nude mice and aggregately deposited in the cell-laden scaffolds. Importantly, GNPs exhibited extensive accumulation in bioprinted scaffolds compared to natural skin and other organs in vivo. GNPs accumulated in the dermis of the transplanted scaffolds, while they stayed in the subcutaneous tissue of the natural skin with no permeation to the dermis, indicating a high absorption tendency of GNPs for artificial scaffolds. The results revealed a lack of similarity between the artificial skin scaffolds and natural skin, which may diminish their potential as artificial skin substitutes. Furthermore, the absorption property of 3D-bioprinted scaffolds suggests their potential as (i) a therapeutic method to absorb and excrete GNPs; and (ii) a strategy for targeted drug delivery of GNPs.

- Matai I, Kaur G, Seyedsalehi A, McClinton A, Laurencin CT. Progress in 3D bioprinting technology for tissue/ organ regenerative engineering. Biomaterials. 2020; 226:119536. doi: 10.1016/j.biomaterials.2019.119536
- Cubo N, Garcia M, Del Canizo JF, Velasco D, Jorcano JL. 3D bioprinting of functional human skin: production and in vivo analysis. Biofabrication. 2016;9(1):015006. doi: 10.1088/1758-5090/9/1/015006
- Zhu W, Ma X, Gou M, Mei D, Zhang K, Chen S. 3D printing of functional biomaterials for tissue engineering. Curr Opin Biotechnol. 2016;40:103-112. doi: 10.1016/j.copbio.2016.03.014
- Li X, Liu B, Pei B, et al. Inkjet bioprinting of biomaterials. Chem Rev. 2020;120(19):10793-10833. doi: 10.1021/acs.chemrev.0c00008
- Douillet C, Nicodeme M, Hermant L, et al. From local to global matrix organization by fibroblasts: a 4D laser-assisted bioprinting approach. Biofabrication. 2022;14(2):025006. doi: 10.1088/1758-5090/ac40ed
- Rossi A, Pescara T, Gambelli AM, et al. Biomaterials for extrusion-based bioprinting and biomedical applications. Front Bioeng Biotechnol. 2024;12:1393641. doi: 10.3389/fbioe.2024.1393641
- Li W, Wang M, Ma H, Chapa-Villarreal FA, Lobo AO, Zhang YS. Stereolithography apparatus and digital light processing-based 3D bioprinting for tissue fabrication. iScience. 2023;26(2):106039. doi: 10.1016/j.isci.2023.106039
- Weng T, Zhang W, Xia Y, et al. 3D bioprinting for skin tissue engineering: Current status and perspectives. J Tissue Eng. 2021;12:20417314211028574. doi: 10.1177/20417314211028574
- Rossi G, Manfrin A, Lutolf MP. Progress and potential in organoid research. Nat Rev Genet. 2018;19(11):671-687. doi: 10.1038/s41576-018-0051-9
- Hong ZX, Zhu ST, Li H, et al. Bioengineered skin organoids: from development to applications. Mil Med Res. 2023;10(1):40. doi: 10.1186/s40779-023-00475-7
- Zhao H, Chen Y, Shao L, et al. Airflow-assisted 3D bioprinting of human heterogeneous microspheroidal organoids with microfluidic nozzle. Small. 2018;14(39):e1802630. doi: 10.1002/smll.201802630
- Michael S, Sorg H, Peck CT, et al. Tissue engineered skin substitutes created by laser-assisted bioprinting form skin-like structures in the dorsal skin fold chamber in mice. PloS One. 2013;8(3):e57741. doi: 10.1371/journal.pone.0057741
- Chen Y, Feng X. Gold nanoparticles for skin drug delivery. Int J Pharm. 2022;625:122122. doi: 10.1016/j.ijpharm.2022.122122
- Revia RA, Stephen ZR, Zhang M. Theranostic nanoparticles for RNA-based cancer treatment. Acc Chem Res. 2019;52(6):1496-1506. doi: 10.1021/acs.accounts.9b00101
- de Puig H, Bosch I, Salcedo N, Collins JJ, Hamad-Schifferli K, Gehrke L. Multiplexed rapid antigen tests developed using multicolored nanoparticles and cross-reactive antibody pairs: implications for pandemic preparedness. Nano Today. 2022;47:101669. doi: 10.1016/j.nantod.2022.101669
- Surendran SP, Moon MJ, Park R, Jeong YY. Bioactive nanoparticles for cancer immunotherapy. Int J Mol Sci. 2018;19(12):3877. doi: 10.3390/ijms19123877
- Yang X, Yang J, Wang L, et al. Pharmaceutical intermediate-modified gold nanoparticles: against multidrug-resistant bacteria and wound-healing application via an electrospun scaffold. ACS Nano. 2017;11(6): 5737-5745. doi: 10.1021/acsnano.7b01240
- Randeria PS, Seeger MA, Wang XQ, et al. siRNA-based spherical nucleic acids reverse impaired wound healing in diabetic mice by ganglioside GM3 synthase knockdown. Proc Natl Acad Sci U S A. 2015;112(18):5573-5578. doi: 10.1073/pnas.1505951112
- Koushki K, Varasteh AR, Shahbaz SK, et al. Dc-specific aptamer decorated gold nanoparticles: a new attractive insight into the nanocarriers for allergy epicutaneous immunotherapy. Int J Pharm. 2020;584:119403. doi: 10.1016/j.ijpharm.2020.119403
- Niu J, Chu Y, Huang YF, et al. Transdermal gene delivery by functional peptide-conjugated cationic gold nanoparticle reverses the progression and metastasis of cutaneous melanoma. ACS Appl Mater Interfaces. 2017;9(11):9388-9401. doi: 10.1021/acsami.6b16378
- Kim HS, Sun X, Lee JH, Kim HW, Fu X, Leong KW. Advanced drug delivery systems and artificial skin grafts for skin wound healing. Adv Drug Deliv Rev. 2019;146:209-239. doi: 10.1016/j.addr.2018.12.014
- Wang XM, Wu XW, Zhao XY, Wang CW, Zhou JN. Exposure-time-dependent subcellular staging of gold nanoparticles deposition and vesicle destruction in mice livers. Nanomedicine. 2021;34:102393. doi: 10.1016/j.nano.2021.102393
- Lee V, Singh G, Trasatti JP, et al. Design and fabrication of human skin by three-dimensional bioprinting. Tissue Eng Part C Methods. 2014;20(6):473-484. doi: 10.1089/ten.TEC.2013.0335
- Lee W, Debasitis JC, Lee VK, et al. Multi-layered culture of human skin fibroblasts and keratinocytes through three-dimensional freeform fabrication. Biomaterials. 2009;30(8):1587-1595. doi: 10.1016/j.biomaterials.2008.12.009
- Tiwari R, Pathak K. Local drug delivery strategies towards wound healing. Pharmaceutics. 2023;15(2):634. doi: 10.3390/pharmaceutics15020634
- Osouli-Bostanabad K, Masalehdan T, Kapsa RMI, et al. Traction of 3D and 4D printing in the Healthcare Industry: from drug delivery and analysis to regenerative medicine. ACS Biomater Sci Eng. 2022;8(7):2764-2797. doi: 10.1021/acsbiomaterials.2c00094
- Sufiyan M, Kushwaha P, Ahmad M, Mandal P, Vishwakarma KK. Scaffold-mediated drug delivery for enhanced wound healing: a review. AAPS PharmSciTech. 2024;25(5):137. doi: 10.1208/s12249-024-02855-1
- Mbese Z, Alven S, Aderibigbe BA. Collagen-based nanofibers for skin regeneration and wound dressing applications. Polymers (Basel). 2021;13(24):4368. doi: 10.3390/polym13244368
- Liang Y, He J, Guo B. Functional hydrogels as wound dressing to enhance wound healing. ACS Nano. 2021;15(8):12687-12722. doi: 10.1021/acsnano.1c04206
- An B, Lin YS, Brodsky B. Collagen interactions: drug design and delivery. Adv Drug Deliv Rev. 2016;97:69-84. doi: 10.1016/j.addr.2015.11.013
- Yanez M, Rincon J, Dones A, De Maria C, Gonzales R, Boland T. In vivo assessment of printed microvasculature in a bilayer skin graft to treat full-thickness wounds. Tissue Eng Part A. 2015;21(1-2):224-233. doi: 10.1089/ten.TEA.2013.0561
- Eweida AM, Marei MK. Naturally occurring extracellular matrix scaffolds for dermal regeneration: do they really need cells? Biomed Res Int. 2015;2015:839694. doi: 10.1155/2015/839694
- Lee CH, Singla A, Lee Y. Biomedical applications of collagen. Int J Pharm. 2001;221(1-2):1-22. doi: 10.1016/s0378-5173(01)00691-3
- Kang MS, Lee SY, Kim KS, Han DW. State of the art biocompatible gold nanoparticles for cancer theragnosis. Pharmaceutics. 2020;12(8):701. doi: 10.3390/pharmaceutics12080701
- Akshaya K, Arthi C, Pavithra AJ, et al. Bioconjugated gold nanoparticles as an efficient colorimetric sensor for cancer diagnostics. Photodiagnosis Photodyn Ther. 2020;30:101699. doi: 10.1016/j.pdpdt.2020.101699
- Hahn MA, Singh AK, Sharma P, Brown SC, Moudgil BM. Nanoparticles as contrast agents for in-vivo bioimaging: current status and future perspectives. Anal Bioanal Chem. 2011;399(1):3-27. doi: 10.1007/s00216-010-4207-5
- Oliveira BB, Ferreira D, Fernandes AR, Baptista PV. Engineering gold nanoparticles for molecular diagnostics and biosensing. Wiley Interdiscip Rev Nanomed Nanobiotechnol. 2023;15(1):e1836. doi: 10.1002/wnan.1836
- Comune M, Rai A, Chereddy KK, et al. Antimicrobial peptide-gold nanoscale therapeutic formulation with high skin regenerative potential. J Control Release. 2017;262:58-71. doi: 10.1016/j.jconrel.2017.07.007
- Cao M, Li B, Guo M, et al. In vivo percutaneous permeation of gold nanomaterials in consumer cosmetics: implication in dermal safety assessment of consumer nanoproducts. Nanotoxicology. 2021;15(1):131-144. doi: 10.1080/17435390.2020.1860264
- Huang Y, Yu F, Park YS, et al. Co-administration of protein drugs with gold nanoparticles to enable percutaneous delivery. Biomaterials. 2010;31(34):9086-9091. doi: 10.1016/j.biomaterials.2010.08.046
- Mascarenhas-Melo F, Mathur A, Murugappan S, et al. Inorganic nanoparticles in dermopharmaceutical and cosmetic products: properties, formulation development, toxicity, and regulatory issues. Eur J Pharm Biopharm. 2023;192:25-40. doi: 10.1016/j.ejpb.2023.09.011
- Labouta HI, Liu DC, Lin LL, et al. Gold nanoparticle penetration and reduced metabolism in human skin by toluene. Pharm Res. 2011;28(11):2931-2944. doi: 10.1007/s11095-011-0561-z
- Wilson CG, Sisco PN, Gadala-Maria FA, Murphy CJ, Goldsmith EC. Polyelectrolyte-coated gold nanorods and their interactions with type I collagen. Biomaterials. 2009;30(29):5639-5648. doi: 10.1016/j.biomaterials.2009.07.011
- Slowinska K. Cross-linked collagen gels using gold nanoparticles. Methods Mol Biol. 2018;1798:203-212. doi: 10.1007/978-1-4939-7893-9_16
- Schuetz T, Richmond N, Harmon ME, Schuetz J, Castaneda L, Slowinska K. The microstructure of collagen type I gel cross-linked with gold nanoparticles. Colloids Surf B Biointerfaces. 2013;101:118-125. doi: 10.1016/j.colsurfb.2012.06.006
- Akturk O, Kismet K, Yasti AC, et al. Collagen/gold nanoparticle nanocomposites: a potential skin wound healing biomaterial. J Biomater Appl. 2016;31(2):283-301. doi: 10.1177/0885328216644536
- Sasidharan S, Pottail L. Biodegradable polymers and gold nanoparticle-decorated skin substitutes: synthesis, characterization, and in vitro biological activities. Appl Biochem Biotechnol. 2021;193(10):3232-3252. doi: 10.1007/s12010-021-03600-1
- Khlebtsov N, Dykman L. Biodistribution and toxicity of engineered gold nanoparticles: a review of in vitro and in vivo studies. Chem Soc Rev. 2011;40(3):1647-1671. doi: 10.1039/c0cs00018c
- Sykes EA, Dai Q, Tsoi KM, Hwang DM, Chan WC. Nanoparticle exposure in animals can be visualized in the skin and analysed via skin biopsy. Nat Commun. 2014;5:3796. doi: 10.1038/ncomms4796
- Mortensen LJ, Oberdörster G, Pentland AP, DeLouise LA. In vivo skin penetration of quantum dot nanoparticles in the murine model: the effect of UVR. Nano Lett. 2008;8(9):2779-2787. doi: 10.1021/nl801323y
- Smith LT, Holbrook KA, Byers PH. Structure of the dermal matrix during development and in the adult. J Invest Dermatol. 1982;79(Suppl 1):93s-104s. doi: 10.1111/1523-1747.ep12545877
- Zidek J, Vojtova L, Abdel-Mohsen AM, et al. Accurate micro-computed tomography imaging of pore spaces in collagen-based scaffold. J Mater Sci Mater Med. 2016;27(6):110. doi: 10.1007/s10856-016-5717-2