3D-printed poly(p-dioxanone)/graphene oxide composite bioresorbable stents for congenital heart disease treatment
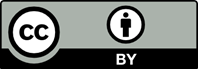
Bioresorbable stents (BRSs) offer significant advantages in treating congenital heart disease (CHD)-related vascular stenoses, especially for pediatric patients. However, the insufficient mechanical performance of polymeric BRSs remains a critical challenge. In this study, poly(p-dioxanone) (PPDO) was incorporated with graphene oxide (GO) for the first time to improve both mechanical properties and biocompatibility. PPDO/GO composites with varying GO content were fabricated via solution mixing and solvent casting, and tensile tests revealed that lower GO content levels (0.2% and 0.5%) significantly improved Young’s modulus, tensile strength, and elongation at break of PPDO due to hydrogen bonding and increased degree of crystallinity. 3D-printed PPDO/GO sliding-lock stents with optimal GO contents were fabricated by fused deposition modeling and demonstrated superior compression force compared to pristine PPDO stents. In vitro hemocompatibility and cytocompatibility assessments indicated that 3D-printed PPDO/GO stents exhibited a low hemolysis rate, reduced platelet adhesion, and enhanced adhesion and proliferation of endothelial cells. In vivo evaluation further demonstrated improved endothelialization in rat abdominal aortas implanted with 3D-printed PPDO/GO filaments for four weeks. Overall, PPDO/0.5%GO exhibited superior performance in compression force, hemocompatibility, cytocompatibility, and in vivo endothelialization. This study, for the first time, combines PPDO with GO and elucidates the mechanism behind the enhanced mechanical properties of PPDO/GO composite material. Using 3D printing, PPDO/GO BRSs with improved compression performance and biocompatibility were developed, highlighting their potential for treating pediatric patients with CHD-related vascular stenoses.

- Su Z, Zou Z, Hay SI, et al. Global, regional, and national time trends in mortality for congenital heart disease, 1990–2019: an age-period-cohort analysis for the Global Burden of Disease 2019 study. eClinicalMedicine. 2022;43:101249. doi: 10.1016/j.eclinm.2021.101249
- Liu Y, Chen S, Zühlke L, et al. Global birth prevalence of congenital heart defects 1970–2017: updated systematic review and meta-analysis of 260 studies. Int J Epidemiol. 2019;48(2):455-463. doi: 10.1093/ije/dyz009
- Baerlocher L, Kretschmar O, Harpes P, Arbenz U, Berger F, Knirsch W. Stent implantation and balloon angioplasty for treatment of branch pulmonary artery stenosis in children. Clin Res Cardiol. 2008;97(5):310-317. doi: 10.1007/s00392-007-0631-8
- Balasubramanian S, Marshall AC, Gauvreau K, et al. Outcomes after stent implantation for the treatment of congenital and postoperative pulmonary vein stenosis in children. Circ Cardiovasc Interv. 2012;5(1):109-117. doi: 10.1161/CIRCINTERVENTIONS.111.964189
- Forbes TJ, Kim DW, Du W, et al. Comparison of surgical, stent, and balloon angioplasty treatment of native coarctation of the aorta. J Am Coll Cardiol. 2011;58(25):2664-2674. doi: 10.1016/j.jacc.2011.08.053
- Lezo JSd, Romero M, Pan M, et al. Stent repair for complex coarctation of aorta. J Am Coll Cardiol Intv. 2015;8(10): 1368-1379. doi: 10.1016/j.jcin.2015.05.018
- Moore JW, Spicer RL, Perry JC, et al. Percutaneous use of stents to correct pulmonary artery stenosis in young children after cavopulmonary anastomosis. Am Heart J. 1995;130(6):1245-1249. doi: 10.1016/0002-8703(95)90149-3
- Ing F. Stents: what’s available to the pediatric interventional cardiologist? Catheter Cardiovasc Interv. 2002;57(3): 374-386. doi: 10.1002/ccd.10342
- Wiebe J, Nef HM, Hamm CW. Current status of bioresorbable scaffolds in the treatment of coronary artery disease. J Am Coll Cardiol. 2014;64(23):2541-2551. doi: 10.1016/j.jacc.2014.09.041
- McMahon S, Bertollo N, Cearbhaill EDO, et al. Bio-resorbable polymer stents: a review of material progress and prospects. Prog Polymer Sci. 2018;83:79-96. doi: 10.1016/j.progpolymsci.2018.05.002
- Sotomi Y, Onuma Y, Collet C, et al. Bioresorbable scaffold. Circ Res. 2017;120(8):1341-1352. doi: 10.1161/CIRCRESAHA.117.310275
- Shibbani K, De Lima e Silva Bagno L, Poulin M-F, et al. Preclinical comparative assessment of a dedicated pediatric poly-L-lactic-acid-based bioresorbable scaffold with a low-profile bare metal stent. Catheter Cardiovasc Interv. 2020;96(4):878-888. doi: 10.1002/ccd.28893
- Wright J, Nguyen A, D’Souza N, et al. Bioresorbable stent to manage congenital heart defects in children. Materialia. 2021;16:101078. doi: 10.1016/j.mtla.2021.101078
- Bowen PK, Guillory RJ, Shearier ER, et al. Metallic zinc exhibits optimal biocompatibility for bioabsorbable endovascular stents. Mater Sci Eng C Mater Biol Appl. 2015;56:467-472. doi: 10.1016/j.msec.2015.07.022
- Sun L, Li J-j, Xu Y-k, Xie Y-m, Wang S-s, Zhang Z-w. Initial status and 3-month results relating to the use of biodegradable nitride iron stents in children and the evaluation of right ventricular function. Front Cardiovasc Med. 2022;9:914370. doi: 10.3389/fcvm.2022.914370
- Veeram Reddy SR, Welch TR, Nugent AW. Biodegradable stent use for congenital heart disease. Prog Pediatr Cardiol. 2021;61:101349. doi: 10.1016/j.ppedcard.2021.101349
- Ormiston JA, Serruys PWS. Bioabsorbable coronary stents. Circ Cardiovasc Intervent. 2009;2(3):255-260. doi: 10.1161/CIRCINTERVENTIONS.109.859173
- Ang HY, Huang YY, Lim ST, Wong P, Joner M, Foin N. Mechanical behavior of polymer-based vs. metallic-based bioresorbable stents. J Thorac Dis. 2017;9(Suppl 9):s923-s934. doi: 10.21037/jtd.2017.06.30
- Sousa AM, Amaro AM, Piedade AP. 3D printing of polymeric bioresorbable stents: a strategy to improve both cellular compatibility and mechanical properties. Polymers (Basel). 2022;14(6):1099. doi: 10.3390/polym14061099.
- Bratincsak A, Moore JW, Gulker B, Choules B, Koren L, El- Said HG. Breaking the limit: mechanical characterization of overexpanded balloon expandable stents used in congenital heart disease. Congenit Heart Dis. 2015;10(1):51-63. doi: 10.1111/chd.12175
- Yasmin F, Vafadar A, Tolouei-Rad M. Application of additive manufacturing in the development of polymeric bioresorbable cardiovascular stents: a review. Adv Mater Technol. 2024;2400210. doi: 10.1002/admt.202400210
- Martins JA, Lach AA, Morris HL, Carr AJ, Mouthuy P-A. Polydioxanone implants: a systematic review on safety and performance in patients. J Biomater Appl. 2020;34(7):902-916. doi: 10.1177/0885328219888841
- Zamiri P, Kuang Y, Sharma U, et al. The biocompatibility of rapidly degrading polymeric stents in porcine carotid arteries. Biomaterials. 2010;31(31):7847-7855. doi: 10.1016/j.biomaterials.2010.06.057
- Zhao F, Xue W, Wang F, et al. Braided bioresorbable cardiovascular stents mechanically reinforced by axial runners. J Mech Behav Biomed Mater. 2019;89:19-32. doi: 10.1016/j.jmbbm.2018.09.003
- Sun J, Sun K, Bai K, et al. Oversized composite braided biodegradable stents with post-dilatation for pediatric applications: mid-term results of a porcine study. Biomater Sci. 2020;8(18):5183-5195. doi: 10.1039/d0bm00567c
- Vahabli E, Mann J, Heidari BS, et al. The technological advancement to engineer next-generation stent-grafts: design, material, and fabrication techniques. Adv Healthc Mater. 2022;11(13):2200271. doi: 10.1002/adhm.202200271
- Wang L, Jiao L, Pang S, Yan P, Wang X, Qiu T. The development of design and manufacture techniques for bioresorbable coronary artery stents. Micromachines. 2021;12(8):990. doi: 10.3390/mi12080990
- Rebelo R, Vila N, Fangueiro R, Carvalho S, Rana S. Influence of design parameters on the mechanical behavior and porosity of braided fibrous stents. Mater Design. 2015;86:237-247. doi: 10.1016/j.matdes.2015.07.051
- Khalaj R, Tabriz AG, Okereke MI, Douroumis D. 3D printing advances in the development of stents. Int J Pharm. 2021;609:121153. doi: 10.1016/j.ijpharm.2021.121153
- Ng WL, An J, Chua CK. Process, material, and regulatory considerations for 3D printed medical devices and tissue constructs. Engineering. 2024;36:146-166. doi: 10.1016/j.eng.2024.01.028
- van Lith R, Baker E, Ware H, et al. 3D-printing strong high-resolution antioxidant bioresorbable vascular stents. Adv Mater Technol. 2016;1(9):1600138. doi: 10.1002/admt.201600138
- Ware HOT, Farsheed AC, Van Lith R, Baker E, Ameer G, Sun C. Process development for high-resolution 3D-printing of bioresorbable vascular stents. Paper presented at: Conference on Advanced Fabrication Technologies for Micro/Nano Optics and Photonics X; Jan 29-Feb 01, 2017; San Francisco, CA. doi: 10.1117/12.2252856
- Flege C, Vogt F, Höges S, et al. Development and characterization of a coronary polylactic acid stent prototype generated by selective laser melting. J Mater Sci Mater Med. 2013;24(1):241-255. doi: 10.1007/s10856-012-4779-z
- Li JF, Ye WY, Fan ZY, Lu ZQ. Stereocomplex poly(lactic acid) vascular stents by 3D-printing with long chain branching structures: toward desirable crystallization properties and mechanical performance. Polym Adv Technol. 2021;32(1): 97-110. doi: 10.1002/pat.5064
- Misra SK, Ostadhossein F, Babu R, et al. 3D-printed multidrug-eluting stent from graphene-nanoplatelet-doped biodegradable polymer composite. Adv Healthc Mater. 2017;6(11). doi: 10.1002/adhm.201700008
- Guerra A, Roca A, de Ciurana J. A novel 3D additive manufacturing machine to biodegradable stents. Paper presented at: 7th Manufacturing-Engineering-Society International Conference (MESIC); Jun 28-30, 2017; Vigo, Spain
- Kim TH, Lee JH, Ahn CB, Hong JH, Son KH, Lee JW. Development of a 3D-printed drug-eluting stent for treating obstructive salivary gland disease. ACS Biomater Sci Eng. 2019;5(7):3572-3581. doi: 10.1021/acsbiomaterials.9b00636
- Lu J, Hu X, Yuan T, et al. 3D-printed poly (P-Dioxanone) stent for endovascular application: in vitro evaluations. Polymers. 2022;14(9):1755. doi: 10.3390/polym14091755
- Feng Q, Jiang W, Sun K, et al. Mechanical properties and in vivo performance of a novel sliding-lock bioabsorbable poly-p-dioxanone stent. J Mater Sci: Mater Med. 2011;22(10):2319. doi: 10.1007/s10856-011-4407-3
- Wu S, Qi Y, Shi W, Kuss M, Chen S, Duan B. Electrospun conductive nanofiber yarns for accelerating mesenchymal stem cells differentiation and maturation into Schwann cell-like cells under a combination of electrical stimulation and chemical induction. Acta Biomater. 2022;139:91-104. doi: 10.1016/j.actbio.2020.11.042
- Zhu L, Liang K, Ji Y. Prominent reinforcing effect of chitin nanocrystals on electrospun polydioxanone nanocomposite fiber mats. J Mech Behav Biomed Mater. 2015;44:35-42. doi: 10.1016/j.jmbbm.2014.12.019
- Shiroud Heidari B, Lopez EM, Chen P, et al. Silane-modified hydroxyapatite nanoparticles incorporated into polydioxanone/poly(lactide-co-caprolactone) creates a novel toughened nanocomposite with improved material properties and in vivo inflammatory responses. Mater Today Bio. 2023;22:100778. doi: 10.1016/j.mtbio.2023.100778
- Suk JW, Piner RD, An J, Ruoff RS. Mechanical properties of monolayer graphene oxide. ACS Nano. 2010;4(11): 6557-6564. doi: 10.1021/nn101781v
- Cao C, Daly M, Singh CV, Sun Y, Filleter T. High strength measurement of monolayer graphene oxide. Carbon. 2015;81:497-504. doi: 10.1016/j.carbon.2014.09.082
- Fu X, Lin J, Liang Z, et al. Graphene oxide as a promising nanofiller for polymer composite. Surf Interfaces. 2023;37:102747. doi: 10.1016/j.surfin.2023.102747
- Shuai C, Peng B, Feng P, Yu L, Lai R, Min A. In situ synthesis of hydroxyapatite nanorods on graphene oxide nanosheets and their reinforcement in biopolymer scaffold. J Adv Res. 2022;35:13-24. doi: 10.1016/j.jare.2021.03.009
- Raslan A, Saenz del Burgo L, Ciriza J, Pedraz JL. Graphene oxide and reduced graphene oxide-based scaffolds in regenerative medicine. Int J Pharma. 2020;580:119226. doi: 10.1016/j.ijpharm.2020.119226
- Sahafnejad-Mohammadi I, Rahmati S, Najmoddin N, Bodaghi M. Biomimetic polycaprolactone-graphene oxide composites for 3D printing bone scaffolds. Macromol Mater Eng. 2023;308(5):2200558. doi: 10.1002/mame.202200558
- Jana S. Endothelialization of cardiovascular devices. Acta Biomater. 2019;99:53-71. doi: 10.1016/j.actbio.2019.08.042
- Wang W, Lin S, Ye Z, et al. Electrospun egg white protein/ polyvinyl alcohol/graphene oxide fibrous wound dressing: Fabrication, antibacterial, cytocompatibility and wound healing assay. Colloids Surf A: Physicochem Eng Aspects. 2023;658:130658. doi: 10.1016/j.colsurfa.2022.130658
- Wang Y, Wu Y, Zhang Y, et al. Graphene oxide coated three-dimensional printed biphasic calcium phosphate scaffold for angiogenic and osteogenic synergy in repairing critical-size bone defect. J Mater Sci Technol. 2023;145:25-39. doi: 10.1016/j.jmst.2022.10.016
- Henriques PC, Pereira AT, Pires AL, Pereira AM, Magalhães FD, Gonçalves IC. Graphene surfaces interaction with proteins, bacteria, mammalian cells, and blood constituents: the impact of graphene platelet oxidation and thickness. ACS Appl Mater Interf. 2020;12(18):21020-21035. doi: 10.1021/acsami.9b21841
- Zhao Z, Zong L, Liu C, et al. Dual strengthened corrosion control of biodegradable coating on magnesium alloy for vascular stent application. Prog Organic Coat. 2023;174:107297. doi: 10.1016/j.porgcoat.2022.107297
- Chen E, Xiong Z, Cai X, et al. Bioresorbable PPDO sliding-lock stents with optimized FDM parameters for congenital heart disease treatment. J Mech Behav Biomed Mater. 2023;138:105609. doi: 10.1016/j.jmbbm.2022.105609
- Davis CS, Hillgartner KE, Han SH, Seppala JE. Mechanical strength of welding zones produced by polymer extrusion additive manufacturing. Addit Manuf. 2017;16:162-166. doi: 10.1016/j.addma.2017.06.006
- Sofińska K, Barbasz J, Witko T, et al. Structural, topographical, and mechanical characteristics of purified polyhydroxyoctanoate polymer. J Appl Polymer Sci. 2019;136(4):47192. doi: 10.1002/app.47192
- Niu R, Gong J, Xu D, Tang T, Sun Z-Y. Influence of molecular weight of polymer matrix on the structure and rheological properties of graphene oxide/polydimethylsiloxane composites. Polymer. 2014;55(21):5445-5453. doi: 10.1016/j.polymer.2014.08.056
- Yang W, Zhong Y, He C, et al. Electrostatic self-assembly of pFe3O4 nanoparticles on graphene oxide: a co-dispersed nanosystem reinforces PLLA scaffolds. J Adv Res. 2020;24:191-203. doi: 10.1016/j.jare.2020.04.009
- Li Z, Deng L, Kinloch IA, Young RJ. Raman spectroscopy of carbon materials and their composites: graphene, nanotubes and fibres. Prog Mater Sci. 2023; 135:101089. doi: 10.1016/j.pmatsci.2023.101089
- Loskot J, Jezbera D, Bezrouk A, et al. Raman spectroscopy as a novel method for the characterization of polydioxanone medical stents biodegradation. Materials (Basel). 2021;14(18):5462. doi: 10.3390/ma14185462
- Zainy M, Huang NM, Vijay Kumar S, Lim HN, Chia CH, Harrison I. Simple and scalable preparation of reduced graphene oxide–silver nanocomposites via rapid thermal treatment. Mater Lett. 2012;89:180-183. doi: 10.1016/j.matlet.2012.08.101
- Zheng Y, Zhou J, Du F, et al. Formation of mesomorphic polymorph, thermal-induced phase transition, and crystalline structure-dependent degradable and mechanical properties of poly(p-dioxanone). Crystal Growth Design. 2019;19(1):166-176. doi: 10.1021/acs.cgd.8b01246
- Ren P-G, Yan D-X, Ji X, Chen T, Li Z-M. Temperature dependence of graphene oxide reduced by hydrazine hydrate. Nanotechnology. 2011;22(5):055705. doi: 10.1088/0957-4484/22/5/055705
- Joy A, Unnikrishnan G, Megha M, et al. Hybrid gold/ graphene oxide reinforced polycaprolactone nanocomposite for biomedical applications. Surf Interfaces. 2023;40:103000. doi: 10.1016/j.surfin.2023.103000
- Du Y, Xing L, Hou P, et al. Dual stimulus response mechanical properties tunable biodegradable and biocompatible PLCL/ PPDO based shape memory composites. Colloids Surf A: Physicochem Eng Aspects. 2022;648:129244. doi: 10.1016/j.colsurfa.2022.129244
- Qian Y, Li C, Qi Y, Zhong J. 3D printing of graphene oxide composites with well controlled alignment. Carbon. 2021;171:777-784. doi: 10.1016/j.carbon.2020.08.077
- Zheng Y, Ashizawa M, Zhang S, et al. Tuning the mechanical properties of a polymer semiconductor by modulating hydrogen bonding interactions. Chem Mater. 2020;32(13):5700-5714. doi: 10.1021/acs.chemmater.0c01437
- Wang R, Xu H, Cheng S, et al. Ultrahigh-energy-density dielectric materials from ferroelectric polymer/glucose all-organic composites with a cross-linking network of hydrogen bonds. Energy Storage Mater. 2022;49:339-347. doi: 10.1016/j.ensm.2022.04.028
- Cheng X, Kumar V, Yokozeki T, et al. Highly conductive graphene oxide/polyaniline hybrid polymer nanocomposites with simultaneously improved mechanical properties. Compos Part A: Appl Sci Manuf. 2016;82:100-107. doi: 10.1016/j.compositesa.2015.12.006
- Zhong J, Gao S, Xue G, Wang B. Study on enhancement mechanism of conductivity induced by graphene oxide for polypyrrole nanocomposites. Macromolecules. 2015;48(5):1592-1597. doi: 10.1021/ma502449k
- Huang X, Nakagawa S, Houjou H, Yoshie N. Insights into the role of hydrogen bonds on the mechanical properties of polymer networks. Macromolecules. 2021;54(9):4070-4080. doi: 10.1021/acs.macromol.1c00120
- Chen Q, Mangadlao JD, Wallat J, De Leon A, Pokorski JK, Advincula RC. 3D printing biocompatible polyurethane/ poly(lactic acid)/graphene oxide nanocomposites: anisotropic properties. ACS Appl Mater Interfaces. 2017;9(4):4015-4023. doi: 10.1021/acsami.6b11793
- Sun N, Di M, Liu Y. Lignin-containing polyurethane elastomers with enhanced mechanical properties via hydrogen bond interactions. Int J Biol Macromol. 2021;184:1-8. doi: 10.1016/j.ijbiomac.2021.06.038
- Wang F, Yang Z, Li J, Zhang C, Sun P. Bioinspired polyurethane using multifunctional block modules with synergistic dynamic bonds. ACS Macro Lett. 2021;10(5): 510-517. doi: 10.1021/acsmacrolett.1c00054
- Peng H, Du X, Cheng X, Wang H, Du Z. Room‐temperature self-healable and stretchable waterborne polyurethane film fabricated via multiple hydrogen bonds. Prog Organic Coat. 2021;151:106081. doi: 10.1016/j.porgcoat.2020.106081
- Abdullah SI, Ansari MNM. Mechanical properties of graphene oxide (GO)/epoxy composites. HBRC J. 2015;11(2):151-156. doi: 10.1016/j.hbrcj.2014.06.001
- Forati T, Atai M, Rashidi AM, Imani M, Behnamghader A. Physical and mechanical properties of graphene oxide/ polyethersulfone nanocomposites. Polym Adv Technol. 2014;25(3):322-328. doi: 10.1002/pat.3243
- Mianehrow H, Lo Re G, Carosio F, et al. Strong reinforcement effects in 2D cellulose nanofibril-graphene oxide (CNF-GO) nanocomposites due to GO-induced CNF ordering. J Mater Chem A. 2020;8(34):17608-17620. doi: 10.1039/d0ta04406g
- Wan CY, Chen BQ. Reinforcement and interphase of polymer/graphene oxide nanocomposites. J Mater Chem. 2012;22(8):3637-3646. doi: 10.1039/c2jm15062j
- Wang H, Li Z, Zuo M, et al. Stretchable, freezing-tolerant conductive hydrogel for wearable electronics reinforced by cellulose nanocrystals toward multiple hydrogen bonding. Carbohydr Polym. 2022;280:119018 doi: 10.1016/j.carbpol.2021.119018
- Wang J, Jin X, Li C, Wang W, Wu H, Guo S. Graphene and graphene derivatives toughening polymers: toward high toughness and strength. Chem Eng J. 2019;370:831-854. doi: 10.1016/j.cej.2019.03.229
- Quaresimin M, Schulte K, Zappalorto M, Chandrasekaran S. Toughening mechanisms in polymer nanocomposites: from experiments to modelling. Compos Sci Technol. 2016;123:187-204. doi: 10.1016/j.compscitech.2015.11.027
- Yang F, Lin Y, Shen S, Gu Y, Shuai C, Feng P. Polydopamine chelating strontium on graphene oxide enhances the mechanical and osteogenic induction properties of PLLA/ PGA bone scaffold. IJB. 2024;10(3):1829. doi: 10.36922/ijb.1829
- Singh G, Divakar Botcha V, Sutar DS, Talwar SS, Srinivasa RS, Major SS. Graphite mediated reduction of graphene oxide monolayer sheets. Carbon. 2015;95:843-851. doi: 10.1016/j.carbon.2015.08.067
- Ortiz M, Rosales-Ibáñez R, Pozos-Guillén A, et al. DPSC colonization of functionalized 3D textiles. J Biomed Mater Res B: Appl Biomater. 2017;105(4):785-794. doi: 10.1002/jbm.b.33609
- Zhu Y, Gao C, Liu X, Shen J. Surface modification of polycaprolactone membrane via aminolysis and biomacromolecule immobilization for promoting cytocompatibility of human endothelial cells. Biomacromolecules. 2002;3(6):1312-1319. doi: 10.1021/bm020074y
- An N, Schedle A, Wieland M, Andrukhov O, Matejka M, Rausch-Fan X. Proliferation, behavior, and cytokine gene expression of human umbilical vascular endothelial cells in response to different titanium surfaces. J Biomed Mater Res A. 2010;93A(1):364-372. doi: 10.1002/jbm.a.32539
- Arrigo R, Frache A. FDM printability of PLA based-materials: the key role of the rheological behavior. Polymers (Basel). 2022;14(9):1754. doi: 10.3390/polym14091754
- Tabriz AG, Scoutaris N, Gong Y, Hui H-W, Kumar S, Douroumis D. Investigation on hot melt extrusion and prediction on 3D printability of pharmaceutical grade polymers. Int J Pharma. 2021;604:120755. doi: 10.1016/j.ijpharm.2021.120755
- Scaffaro R, Maio A. Optimization of two-step techniques engineered for the preparation of polyamide 6 graphene oxide nanocomposites. Compos B: Eng. 2019;165:55-64. doi: 10.1016/j.compositesb.2018.11.107
- Garcia-Villen F, López-Zárraga F, Viseras C, et al. Three-dimensional printing as a cutting-edge, versatile and personalizable vascular stent manufacturing procedure: Toward tailor-made medical devices. Int J Bioprint. 2023;9(2):664. doi: 10.18063/ijb.v9i2.664
- Meng X, Cheng Y, Wang P, et al. Enhanced hemocompatibility of a direct chemical vapor deposition-derived graphene film. ACS Appl Mater Interfaces. 2021;13(4):4835-4843. doi: 10.1021/acsami.0c19790
- Jaffer IH, Fredenburgh JC, Hirsh J, Weitz JI. Medical device-induced thrombosis: what causes it and how can we prevent it? J Thromb Haemost. 2015;13(S1):S72-S81. doi: 10.1111/jth.12961
- Breton HL, Plow EF, Topol EJ. Role of platelets in restenosis after percutaneous coronary revascularization. J Am Coll Cardiol. 1996;28(7):1643-1651. doi: 10.1016/S0735-1097(96)00417-2
- Pan C, Xu R, Chen J, Zhang Q, Deng L, Hong Q. A CO-releasing coating based on carboxymethyl chitosan-functionalized graphene oxide for improving the anticorrosion and biocompatibility of magnesium alloy stent materials. Int J Biol Macromol. 2024;271:132487. doi: 10.1016/j.ijbiomac.2024.132487
- Liu W, Wang XY, Feng YK. Restoring endothelial function: shedding light on cardiovascular stent development. Biomater Sci. 2023;11(12):4132-4150. doi: 10.1039/d3bm00390f
- Wang X, Fang F, Ni Y, et al. The combined contribution of vascular endothelial cell migration and adhesion to stent re-endothelialization. Front Cell Dev Biol. 2021;9:641382. doi: 10.3389/fcell.2021.641382
- Yoon HH, Bhang SH, Kim T, Yu T, Hyeon T, Kim B-S. Dual roles of graphene oxide in chondrogenic differentiation of adult stem cells: cell-adhesion substrate and growth factor-delivery carrier. Adv Funct Mater. 2014;24(41):6455-6464. doi: 10.1002/adfm.201400793
- Lee WC, Lim CHYX, Shi H, et al. Origin of enhanced stem cell growth and differentiation on graphene and graphene oxide. ACS Nano. 2011;5(9):7334-7341. doi: 10.1021/nn202190c
- Jing X, Mi H-Y, Salick MR, Cordie TM, Peng X-F, Turng L-S. Electrospinning thermoplastic polyurethane/graphene oxide scaffolds for small diameter vascular graft applications. Mater Sci Eng C. 2015;49:40-50. doi: 10.1016/j.msec.2014.12.060
.