3D bioprinting technologies for the enhancement and application of functional lung organoid models
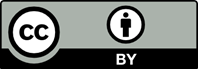
Respiratory diseases, ranging from minor infections to serious chronic diseases and malignancies, negatively affect the respiratory system and are influenced by various environmental factors such as air pollution, occupational hazards, and tobacco smoke, as well as lifestyle, genetic causes, and infectious agents. The prevalence and severity of respiratory diseases require the development of advanced models to better understand their pathophysiology and develop effective treatments. In this context, 3D bioprinting technology emerges as an innovative tool to create functional lung organoid models. The use of induced pluripotent stem cells and extracellular matrix in bioprinting enables the development of organoids that closely mimic human lung tissue. Bioprinting-based organoids can better replicate the dynamic environment of the human lung, facilitating more accurate disease modeling and drug testing. In this review, we highlight the potential of bioprinted lung organoids in understanding the mechanisms of chronic respiratory diseases, testing the efficacy and safety of new drugs, and exploring regenerative medicine approaches. The integration of advanced bioprinting and organoid technologies is a promising field in respiratory disease research and treatment, offering new hope for patients suffering from lung diseases.

- Levine SM, Marciniuk DD. Global impact of respiratory disease: what can we do, together, to make a difference? Chest. 2022;161(5):1153-1154. doi: 10.1016/j.chest.2022.01.014
- Shukla SD, Swaroop Vanka K, Chavelier A, et al. Chronic respiratory diseases: an introduction and need for novel drug delivery approaches. In: Targeting Chronic Inflammatory Lung Diseases Using Advanced Drug Delivery Systems; 2020:1-31. doi: 10.1016/B978-0-12-820658-4.00001-7
- Ayilya BL, Balde A, Ramya M, Benjakul S, Kim SK, Nazeer RA. Insights on the mechanism of bleomycin to induce lung injury and associated in vivo models: a review. Int Immunopharmacol. 2023;121:110493. doi: 10.1016/j.intimp.2023.110493
- Ribble A, Hellmann J, Conklin DJ, Bhatnagar A, Haberzettl P. Fine particulate matter (PM(2.5))-induced pulmonary oxidative stress contributes to increases in glucose intolerance and insulin resistance in a mouse model of circadian dyssynchrony. Sci Total Environ. 2023;877:162934. doi: 10.1016/j.scitotenv.2023.162934
- John G, Kohse K, Orasche J, et al. The composition of cigarette smoke determines inflammatory cell recruitment to the lung in COPD mouse models. Clin Sci (Lond). 2014;126(3):207-221. doi: 10.1042/cs20130117
- Khedoe PP, Wong MC, Wagenaar GT, et al. The effect of PPE-induced emphysema and chronic LPS-induced pulmonary inflammation on atherosclerosis development in APOE*3- LEIDEN mice. PLoS One. 2013;8(11):e80196. doi: 10.1371/journal.pone.0080196
- Ishida Y, Kuninaka Y, Mukaida N, Kondo T. Immune mechanisms of pulmonary fibrosis with bleomycin. Int J Mol Sci. 2023;24(4):3149. doi: 10.3390/ijms24043149
- Su J, Ye Q, Zhang D, et al. Joint association of cigarette smoking and PM(2.5) with COPD among urban and rural adults in regional China. BMC Pulm Med. 2021;21(1):87. doi: 10.1186/s12890-021-01465-y
- Kwon MC, Berns A. Mouse models for lung cancer. Mol Oncol. 2013;7(2):165-177. doi: 10.1016/j.molonc.2013.02.010
- Uhl EW, Warner NJ. Mouse models as predictors of human responses: evolutionary medicine. Curr Pathobiol Rep. 2015;3(3):219-223. doi: 10.1007/s40139-015-0086-y
- Rydell-Törmänen K, Johnson JR. The applicability of mouse models to the study of human disease. Methods Mol Biol. 2019;1940:3-22. doi: 10.1007/978-1-4939-9086-3_1
- Patisaul HB, Fenton SE, Aylor D. Animal models of endocrine disruption. Best Pract Res Clin Endocrinol Metab. 2018;32(3):283-297. doi: 10.1016/j.beem.2018.03.011
- Chae S, Ha DH, Lee H. 3D bioprinting strategy for engineering vascularized tissue models. Int J Bioprint. 2023;9(5):748. doi: 10.18063/ijb.748
- Bosáková V, De Zuani M, Sládková L, et al. Lung organoids-the ultimate tool to dissect pulmonary diseases? Front Cell Dev Biol. 2022;10:899368. doi: 10.3389/fcell.2022.899368
- Shrestha J, Razavi Bazaz S, Aboulkheyr Es H, et al. Lung-on-a-chip: the future of respiratory disease models and pharmacological studies. Crit Rev Biotechnol. 2020;40(2):213-230. doi: 10.1080/07388551.2019.1710458
- Hermanns MI, Unger RE, Kehe K, Peters K, Kirkpatrick CJ. Lung epithelial cell lines in coculture with human pulmonary microvascular endothelial cells: development of an alveolo-capillary barrier in vitro. Lab Invest. 2004;84(6): 736-752. doi: 10.1038/labinvest.3700081
- Birgersdotter A, Sandberg R, Ernberg I. Gene expression perturbation in vitro—a growing case for three-dimensional (3D) culture systems. Semin Cancer Biol. 2005;15(5):405-412. doi: 10.1016/j.semcancer.2005.06.009
- Pampaloni F, Reynaud EG, Stelzer EH. The third dimension bridges the gap between cell culture and live tissue. Nat Rev Mol Cell Biol. 2007;8(10):839-845. doi: 10.1038/nrm2236
- Murphy SV, Atala A. 3D bioprinting of tissues and organs. Nat Biotechnol. 2014;32(8):773-785. doi: 10.1038/nbt.2958
- Zou S, Ye J, Wei Y, Xu J. Characterization of 3D-bioprinted in vitro lung cancer models using RNA-sequencing techniques. Bioengineering (Basel). 2023;10(6):667. doi: 10.3390/bioengineering10060667
- Wang X, Zhang X, Dai X, et al. Tumor-like lung cancer model based on 3D bioprinting. 3 Biotech. 2018;8:501. doi: 10.1007/s13205-018-1519-1
- Maina JN. Fundamental structural aspects and features in the bioengineering of the gas exchangers: comparative perspectives. Adv Anat Embryol Cell Biol. 2002;163:Iii-xii, 1-108. doi: 10.1007/978-3-642-55917-4
- Khan YS, Lynch DT. Histology, Lung. 2023. In: StatPearls [Internet]. Treasure Island (FL): StatPearls Publishing; 2024.
- Brownfield DG, de Arce AD, Ghelfi E, Gillich A, Desai TJ, Krasnow MA. Alveolar cell fate selection and lifelong maintenance of AT2 cells by FGF signaling. Nat Commun. 2022;13(1):7137. doi: 10.1038/s41467-022-34059-1
- Yang J, Hernandez BJ, Martinez Alanis D, et al. The development and plasticity of alveolar type 1 cells. Development. 2016;143(1):54-65. doi: 10.1242/dev.130005
- Han S, Lee M, Shin Y, et al. Mitochondrial integrated stress response controls lung epithelial cell fate. Nature. 2023;620(7975):890-897. doi: 10.1038/s41586-023-06423-8
- Quan R, Shi C, Fang B, et al. Age-dependent inflammatory microenvironment mediates alveolar regeneration. Int J Mol Sci. 2024;25(6):3476. doi: 10.3390/ijms25063476
- Basil MC, Katzen J, Engler AE, et al. The cellular and physiological basis for lung repair and regeneration: past, present, and future. Cell Stem Cell. 2020;26(4):482-502. doi: 10.1016/j.stem.2020.03.009
- Ruaro B, Salton F, Braga L, et al. The history and mystery of alveolar epithelial type II cells: focus on their physiologic and pathologic role in lung. Int J Mol Sci. 2021;22(5):2566. doi: 10.3390/ijms22052566
- Zhang J, Liu Y. Epithelial stem cells and niches in lung alveolar regeneration and diseases. Chin Med J Pulm Crit Care Med. 2024;2(1):17-26. doi: 10.1016/j.pccm.2023.10.007
- Beers MF, Moodley Y. When is an alveolar type 2 cell an alveolar type 2 cell? A conundrum for lung stem cell biology and regenerative medicine. Am J Respir Cell Mol Biol. 2017;57(1):18-27. doi: 10.1165/rcmb.2016-0426PS
- Kendall RT, Feghali-Bostwick CA. Fibroblasts in fibrosis: novel roles and mediators. Front Pharmacol. 2014;5:123. doi: 10.3389/fphar.2014.00123
- Fu Z, Xu YS, Cai CQ. Ginsenoside Rg3 inhibits pulmonary fibrosis by preventing HIF-1α nuclear localisation. BMC Pulm Med. 2021;21(1):70. doi: 10.1186/s12890-021-01426-5
- Wilson MS, Wynn TA. Pulmonary fibrosis: pathogenesis, etiology and regulation. Mucosal Immunol. 2009;2(2):103-121. doi: 10.1038/mi.2008.85
- Li YX, Wang HB, Li J, Jin JB, Hu JB, Yang CL. Targeting pulmonary vascular endothelial cells for the treatment of respiratory diseases. Front Pharmacol. 2022;13:983816. doi: 10.3389/fphar.2022.983816
- Vassiliou AG, Kotanidou A, Dimopoulou I, Orfanos SE. Endothelial damage in acute respiratory distress syndrome. Int J Mol Sci. 2020;21(22):8793. doi: 10.3390/ijms21228793
- Correale M, Chirivi F, Bevere EML, et al. Endothelial function in pulmonary arterial hypertension: from bench to bedside. J Clin Med. 2024;13(8):2444. doi: 10.3390/jcm13082444
- Meng X, Cui G, Peng G. Lung development and regeneration: newly defined cell types and progenitor status. Cell Regen. 2023;12(1):5. doi: 10.1186/s13619-022-00149-0
- Zhao Z, Chen X, Dowbaj AM, et al. Organoids. Nat Rev Methods Primers. 2022;2:94. doi: 10.1038/s43586-022-00174-y
- Arjmand B, Rabbani Z, Soveyzi F, et al. Advancement of organoid technology in regenerative medicine. Regen Eng Transl Med. 2023;9(1):83-96. doi: 10.1007/s40883-022-00271-0
- Parikh P, Wicher S, Khandalavala K, Pabelick CM, Britt RD, Jr., Prakash YS. Cellular senescence in the lung across the age spectrum. Am J Physiol Lung Cell Mol Physiol. 2019;316(5):L826-L842. doi: 10.1152/ajplung.00424.2018
- Koks S, Dogan S, Tuna BG, Gonzalez-Navarro H, Potter P, Vandenbroucke RE. Mouse models of ageing and their relevance to disease. Mech Ageing Dev. 2016;160:41-53. doi: 10.1016/j.mad.2016.10.001
- Fan C, Wu Y, Rui X, et al. Animal models for COVID-19: advances, gaps and perspectives. Signal Transduct Target Ther. 2022;7(1):220. doi: 10.1038/s41392-022-01087-8
- Cox TC. Utility and limitations of animal models for the functional validation of human sequence variants. Mol Genet Genomic Med. 2015;3(5):375-382. doi: 10.1002/mgg3.167
- Duval K, Grover H, Han LH, et al. Modeling physiological events in 2D vs. 3D cell culture. Physiology (Bethesda). 2017;32(4):266-277. doi: 10.1152/physiol.00036.2016
- Jubelin C, Munoz-Garcia J, Griscom L, et al. Three-dimensional in vitro culture models in oncology research. Cell Biosci. 2022;12(1):155. doi: 10.1186/s13578-022-00887-3
- Cacciamali A, Villa R, Dotti S. 3D cell cultures: evolution of an ancient tool for new applications. Front Physiol. 2022;13:836480. doi: 10.3389/fphys.2022.836480
- Miller AJ, Dye BR, Ferrer-Torres D, et al. Generation of lung organoids from human pluripotent stem cells in vitro. Nat Protoc. 2019;14(2):518-540. doi: 10.1038/s41596-018-0104-8
- Kong J, Wen S, Cao W, et al. Lung organoids, useful tools for investigating epithelial repair after lung injury. Stem Cell Res Ther. 2021;12(1):95. doi: 10.1186/s13287-021-02172-5
- Hein RFC, Conchola AS, Fine AS, et al. Stable iPSC-derived NKX2-1+ lung bud tip progenitor organoids give rise to airway and alveolar cell types. Development. 2022;149(20):dev200693. doi: 10.1242/dev.200693
- Chiu MC, Li C, Liu X, et al. A bipotential organoid model of respiratory epithelium recapitulates high infectivity of SARS-CoV-2 Omicron variant. Cell Discov. 2022;8(1):57. doi: 10.1038/s41421-022-00422-1
- Sucre JM, Wilkinson D, Vijayaraj P, et al. A three-dimensional human model of the fibroblast activation that accompanies bronchopulmonary dysplasia identifies Notch-mediated pathophysiology. Am J Physiol Lung Cell Mol Physiol. 2016;310(10):L889-L898. doi: 10.1152/ajplung.00446.2015
- Ng-Blichfeldt JP, de Jong T, Kortekaas RK, et al. TGF-beta activation impairs fibroblast ability to support adult lung epithelial progenitor cell organoid formation. Am J Physiol Lung Cell Mol Physiol. 2019;317(1):L14-L28. doi: 10.1152/ajplung.00400.2018
- Sachs N, Papaspyropoulos A, Zomer-van Ommen DD, et al. Long-term expanding human airway organoids for disease modeling. EMBO J. 2019;38(4):e100300. doi: 10.15252/embj.2018100300
- Chan LLY, Anderson DE, Cheng HS, et al. The establishment of COPD organoids to study host-pathogen interaction reveals enhanced viral fitness of SARS-CoV-2 in bronchi. Nat Commun. 2022;13(1):7635. doi: 10.1038/s41467-022-35253-x
- Laube M, Pietsch S, Pannicke T, Thome UH, Fabian C. Development and functional characterization of fetal lung organoids. Front Med (Lausanne). 2021;8:678438. doi: 10.3389/fmed.2021.678438
- Matkovic Leko I, Schneider RT, Thimraj TA, et al. A distal lung organoid model to study interstitial lung disease, viral infection and human lung development. Nat Protoc. 2023;18(7):2283-2312. doi: 10.1038/s41596-023-00827-6
- Vazquez-Armendariz AI, Herold S. From clones to buds and branches: the use of lung organoids to model branching morphogenesis ex vivo. Front Cell Dev Biol. 2021;9:631579. doi: 10.3389/fcell.2021.631579
- Jain KG, Xi NM, Zhao R, Ahmad W, Ali G, Ji HL. Alveolar type 2 epithelial cell organoids: focus on culture methods. Biomedicines. 2023;11(11):3034. doi: 10.3390/biomedicines11113034
- Mulay A, Konda B, Garcia G, Jr., et al. SARS-CoV-2 infection of primary human lung epithelium for COVID-19 modeling and drug discovery. Cell Rep. 2021;35(5):109055. doi: 10.1016/j.celrep.2021.109055
- Konda B, Mulay A, Yao C, Beil S, Israely E, Stripp BR. Isolation and enrichment of human lung epithelial progenitor cells for organoid culture. J Vis Exp. 2020;(161). doi: 10.3791/61541
- Van Hung T, Emoto N, Vignon-Zellweger N, et al. Inhibition of vascular endothelial growth factor receptor under hypoxia causes severe, human-like pulmonary arterial hypertension in mice: potential roles of interleukin-6 and endothelin. Life Sci. 2014;118(2):313-328. doi: 10.1016/j.lfs.2013.12.215
- Zhang Y, Zhang H, Li S, Huang K, Jiang L, Wang Y. Metformin alleviates LPS-induced acute lung injury by regulating the SIRT1/NF-kappaB/NLRP3 pathway and inhibiting endothelial cell pyroptosis. Front Pharmacol. 2022;13:801337. doi: 10.3389/fphar.2022.801337
- Selimovic N, Bergh CH, Andersson B, Sakiniene E, Carlsten H, Rundqvist B. Growth factors and interleukin-6 across the lung circulation in pulmonary hypertension. Eur Respir J. 2009;34(3):662-668. doi: 10.1183/09031936.00174908
- Finicelli M, Digilio FA, Galderisi U, Peluso G. The emerging role of macrophages in chronic obstructive pulmonary disease: the potential impact of oxidative stress and extracellular vesicle on macrophage polarization and function. Antioxidants (Basel). 2022;11(3):464. doi: 10.3390/antiox11030464
- Osorio-Valencia S, Zhou B. Roles of macrophages and endothelial cells and their crosstalk in acute lung injury. Biomedicines. 2024;12(3):632. doi: 10.3390/biomedicines12030632
- Ramachandran S, Verma AK, Dev K, et al. Role of cytokines and chemokines in NSCLC immune navigation and proliferation. Oxid Med Cell Longev. 2021;2021:5563746. doi: 10.1155/2021/5563746
- Huskin G, Chen J, Davis T, Jun HW. Tissue-engineered 3D in vitro disease models for high-throughput drug screening. Tissue Eng Regen Med. 2023;20(4):523-538. doi: 10.1007/s13770-023-00522-3
- da Rosa NN, Appel JM, Irioda AC, et al. Three-dimensional bioprinting of an in vitro lung model. Int J Mol Sci. 2023;24(6):5852. doi: 10.3390/ijms24065852
- Mu P, Zhou S, Lv T, et al. Newly developed 3D in vitro models to study tumor-immune interaction. J Exp Clin Cancer Res. 2023;42(1):81. doi: 10.1186/s13046-023-02653-w
- Gibson I, Rosen DW, Stucker B, et al. Additive Manufacturing Technologies, vol 17. Springer; 2021. doi: 10.1007/978-1-4419-1120-9
- Shahrubudin N, Lee TC, Ramlan R. An overview on 3D printing technology: technological, materials, and applications. Procedia Manufact. 2019;35:1286-1296. doi: 10.1016/j.promfg.2019.06.089
- Gopinathan J, Noh I. Recent trends in bioinks for 3D printing. Biomater Res. 2018;22(1):11. doi: 10.1186/s40824-018-0122-1
- Jakab K, Norotte C, Marga F, Murphy K, Vunjak-Novakovic G, Forgacs G. Tissue engineering by self-assembly and bio-printing of living cells. Biofabrication. 2010;2(2):022001. doi: 10.1088/1758-5082/2/2/022001
- Mandrycky C, Wang Z, Kim K, Kim D-H. 3D bioprinting for engineering complex tissues. Biotechnol Adv. 2016;34(4):422-434. doi: 10.1016/j.biotechadv.2015.12.011
- Fan P, Jin F, Qin Y, et al. Multiscale 3D bioprinting for the recapitulation of lung tissue. IJB. 2023;9(6):1166. doi: 10.36922/ijb.1166
- Ozbolat IT. Bioprinting scale-up tissue and organ constructs for transplantation. Trends Biotechnol. 2015;33(7):395-400. doi: 10.1016/j.tibtech.2015.04.005
- Groll J, Boland T, Blunk T, et al. Biofabrication: reappraising the definition of an evolving field. Biofabrication. 2016;8(1):013001. doi: 10.1088/1758-5090/8/1/013001
- Miller AJ, Spence JR. In vitro models to study human lung development, disease and homeostasis. Physiology (Bethesda). 2017;32(3):246-260. doi: 10.1152/physiol.00041.2016
- Matai I, Kaur G, Seyedsalehi A, McClinton A, Laurencin CT. Progress in 3D bioprinting technology for tissue/ organ regenerative engineering. Biomaterials. 2020;226: 119536. doi: 10.1016/j.biomaterials.2019.119536
- Ozbolat IT, Hospodiuk M. Current advances and future perspectives in extrusion-based bioprinting. Biomaterials. 2016;76:321-343. doi: 10.1016/j.biomaterials.2015.10.076
- Gungor-Ozkerim PS, Inci I, Zhang YS, Khademhosseini A, Dokmeci MR. Bioinks for 3D bioprinting: an overview. Biomater Sci. 2018;6(5):915-946. doi: 10.1039/c7bm00765e
- Derby B. Printing and prototyping of tissues and scaffolds. Science. 2012;338(6109):921-926. doi: 10.1126/science.1226340
- Ning L, Chen X. A brief review of extrusion‐based tissue scaffold bio‐printing. Biotechnol J. 2017;12(8):1600671. doi: 10.1002/biot.201600671
- Xu T, Jin J, Gregory C, Hickman JJ, Boland T. Inkjet printing of viable mammalian cells. Biomaterials. 2005;26(1):93-99. doi: 10.1016/j.biomaterials.2004.04.011
- Kang D, Park JA, Kim W, et al. All‐inkjet‐printed 3D alveolar barrier model with physiologically relevant microarchitecture. Adv Sci (Weinh). 2021;8(10):2004990. doi: 10.1002/advs.202004990
- Scoutaris N, Ross S, Douroumis D. Current trends on medical and pharmaceutical applications of inkjet printing technology. Pharm Res. 2016;33(8):1799-1816. doi: 10.1007/s11095-016-1931-3
- Yang H, Yang K-H, Narayan RJ, Ma S. Laser-based bioprinting for multilayer cell patterning in tissue engineering and cancer research. Essays Biochem. 2021;65(3):409-416. doi: 10.1042/EBC20200093
- Gruene M, Pflaum M, Hess C, et al. Laser printing of three-dimensional multicellular arrays for studies of cell–cell and cell–environment interactions. Tissue Eng Part C: Methods. 2011;17(10):973-982. doi: 10.1089/ten.TEC.2011.0185
- Catros S, Guillotin B, Bačáková M, Fricain J-C, Guillemot F. Effect of laser energy, substrate film thickness and bioink viscosity on viability of endothelial cells printed by laser-assisted bioprinting. Appl Surf Sci. 2011;257(12): 5142-5147. doi: 10.1016/j.apsusc.2010.11.049
- Guillotin B, Souquet A, Catros S, et al. Laser assisted bioprinting of engineered tissue with high cell density and microscale organization. Biomaterials. 2010;31(28):7250-7256. doi: 10.1016/j.biomaterials.2010.05.055
- Zhu W, Qu X, Zhu J, et al. Direct 3D bioprinting of prevascularized tissue constructs with complex microarchitecture. Biomaterials. 2017;124:106-115. doi: 10.1016/j.biomaterials.2017.01.042
- Li J, Chen M, Fan X, Zhou H. Recent advances in bioprinting techniques: approaches, applications and future prospects. J Transl Med. 2016;14:271. doi: 10.1186/s12967-016-1028-0
- Geckil H, Xu F, Zhang X, Moon S, Demirci U. Engineering hydrogels as extracellular matrix mimics. Nanomedicine (Lond). 2010;5(3):469-484. doi: 10.2217/nnm.10.12
- Hinton TJ, Jallerat Q, Palchesko RN, et al. Three-dimensional printing of complex biological structures by freeform reversible embedding of suspended hydrogels. Sci Adv. 2015;1(9):e1500758. doi: 10.1126/sciadv.1500758
- Kang H-W, Lee SJ, Ko IK, Kengla C, Yoo JJ, Atala A. A 3D bioprinting system to produce human-scale tissue constructs with structural integrity. Nat Biotechnol. 2016;34(3):312-319. doi: 10.1038/nbt.3413
- Wang X, Ao Q, Tian X, et al. 3D bioprinting technologies for hard tissue and organ engineering. Materials (Basel). 2016;9(10):802. doi: 10.3390/ma9110911
- Krakos A, Cieslak A, Hartel E, Labowska MB, Kulbacka J, Detyna J. 3D bio-printed hydrogel inks promoting lung cancer cell growth in a lab-on-chip culturing platform. Mikrochim Acta. 2023;190(9):349. doi: 10.1007/s00604-023-05931-8
- Gerboles AG, Galetti M, Rossi S, et al. Three-dimensional bioprinting of organoid-based scaffolds (OBST) for long-term nanoparticle toxicology investigation. Int J Mol Sci. 2023;24(7):6595. doi: 10.3390/ijms24076595
- Urciuolo A, Giobbe GG, Dong Y, et al. Hydrogel-in-hydrogel live bioprinting for guidance and control of organoids and organotypic cultures. Nat Commun. 2023;14(1):3128. doi: 10.1038/s41467-023-37953-4
- Choi YM, Lee H, Ann M, Song M, Rheey J, Jang J. 3D bioprinted vascularized lung cancer organoid models with underlying disease capable of more precise drug evaluation. Biofabrication. 2023;15(3):4104. doi: 10.1088/1758-5090/acd95f
- Ma X, Liu J, Zhu W, et al. 3D bioprinting of functional tissue models for personalized drug screening and in vitro disease modeling. Adv Drug Deliv Rev. 2018;132:235-251. doi: 10.1016/j.addr.2018.06.011
- Ashammakhi N, Ahadian S, Xu C, et al. Bioinks and bioprinting technologies to make heterogeneous and biomimetic tissue constructs. Mater Today Bio. 2019;1:100008. doi: 10.1016/j.mtbio.2019.100008
- Chen XB, Fazel Anvari-Yazdi A, Duan X, et al. Biomaterials / bioinks and extrusion bioprinting. Bioact Mater. 2023;28:511-536. doi: 10.1016/j.bioactmat.2023.06.006
- Grigoryan B, Paulsen SJ, Corbett DC, et al. Multivascular networks and functional intravascular topologies within biocompatible hydrogels. Science. 2019;364(6439):458-464. doi: 10.1126/science.aav9750
- Bernal PN, Delrot P, Loterie D, et al. Volumetric bioprinting of complex living-tissue constructs within seconds. Adv Mater. 2019;31(42):e1904209. doi: 10.1002/adma.201904209
- Richard C, Neild A, Cadarso VJ. The emerging role of microfluidics in multi-material 3D bioprinting. Lab Chip. 2020;20(12):2044-2056. doi: 10.1039/c9lc01184f
- Mironov V, Kasyanov V, Drake C, Markwald RR. Organ printing: promises and challenges. Regen Med. 2008;3(1):93-103. doi: 10.2217/17460751.3.1.93
- Sekar MP, Budharaju H, Zennifer A, et al. Current standards and ethical landscape of engineered tissues-3D bioprinting perspective. J Tissue Eng. 2021;12:20417314211027677. doi: 10.1177/20417314211027677
- Saini G, Segaran N, Mayer JL, Saini A, Albadawi H, Oklu R. Applications of 3D bioprinting in tissue engineering and regenerative medicine. J Clin Med. 2021;10(21):4966. doi: 10.3390/jcm10214966
- Huang G, Zhao Y, Chen D, et al. Applications, advancements, and challenges of 3D bioprinting in organ transplantation. Biomater Sci. 2024;12(6):1425-1448. doi: 10.1039/d3bm01934a