Mechanism study of structural functional dual biomimetic composite bone scaffold for repair of mandibular defects in rabbits
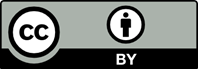
The present study aims to explore the potential mechanisms of bone tissue-engineered triply periodic minimal surfaces (TPMS) scaffolds containing injectable platelet-rich fibrin and stromal cell-derived factor-1 (in short, SIT scaffolds) in promoting angiogenesis in mandibular defects. Surface structures with a 70% porosity were created using the Matlab R2020a program. SIT scaffolds were fabricated using digital laser processing. Histological structures on SIT scaffolds inoculated with rabbit bone marrow mesenchymal stem cells (BMSCs) were observed using alkaline phosphatase (ALP) immunohistochemical staining, Alcian blue staining, and Masson-Goldner staining. Double-end sequencing in PE150 mode was performed using the Illumina NovaSeq™ 6000. Analyses such as Gene Ontology (GO), Kyoto Encyclopedia of Genes and Genomes (KEGG), protein-protein interactions, and gene set enrichment analysis (GSEA) were conducted to delineate the gene functions. RT-qPCR was performed to confirm the expression of genes of interest. Finally, cells on different samples were examined by confocal laser scanning microscopy to observe c-Jun expression. Confocal imaging and quantitative analysis showed that BMSCs supported by the SIT scaffold had a greater tendency to differentiate into osteoblasts than those supported by the TPMS scaffold or the control group. The SIT group exhibited the most intense ALP staining. The expression of angiogenesis-related factors VEGFA was significantly upregulated in T and SIT groups. Expression of osteogenic gene RUNX2 and c-Fos/c-Jun pathway genes FOS/JUN was significantly upregulated in the SIT group. GSEA revealed that the WNT signaling pathway and MAPK signaling pathway were more active in the SIT group. Immunofluorescence showed that the c-Jun is highly expressed in newly formed capillaries in the SIT group. In conclusion, both TPMS and SIT scaffolds promote angiogenesis in mandibular defects.

- Brown JS, Barry C, Ho M, Shaw R. A new classification for mandibular defects after oncological resection. Lancet Oncol. 2016;17(1):e23-30. doi: 10.1016/S1470-2045(15)00310-1
- Miljanovic D, Seyedmahmoudian M, Stojcevski A, Horan B. Design and fabrication of implants for mandibular and craniofacial defects using different medical-additive manufacturing technologies: a review. Ann Biomed Eng. 2020;48(9):2285-2300. doi: 10.1007/s10439-020-02567-0
- Sivaraman K, Chopra A, Venkatesh SB. Clinical importance of median mandibular flexure in oral rehabilitation: a review. J Oral Rehabil. 2016;43(3):215-225. doi: 10.1111/joor.12361
- Pickrell BB, Serebrakian AT, Maricevich RS. Mandible fractures. Semin Plast Surg. 2017;31(2):100-107. doi: 10.1055/s-0037-1601374
- García-Lamas L, Lozano D, Jiménez-Díaz V, et al. Enriched mesoporous bioactive glass scaffolds as bone substitutes in critical diaphyseal bone defects in rabbits. Acta Biomater. 2024;180:104-114. doi: 10.1016/j.actbio.2024.04.005
- Stahl A, Yang YP. Regenerative approaches for the treatment of large bone defects. Tissue Eng Part B Rev. 2021;27(6):539-547. doi: 10.1089/ten.TEB.2020.0281
- Zheng X, Fu Z, Du K, Wang C, Yi Y. Minimal surface designs for porous materials: from microstructures to mechanical properties. J Mater Sci. 2018;53(14):10194-10208. doi: 10.1007/s10853-018-2285-5
- Shen M, Li Y, Lu F, et al. Bioceramic scaffolds with triply periodic minimal surface architectures guide early-stage bone regeneration. Bioact Mater. 2023;25:374-386. doi: 10.1016/j.bioactmat.2023.02.012
- Wang C, Lai J, Li K, et al. Cryogenic 3D printing of dual-delivery scaffolds for improved bone regeneration with enhanced vascularization. Bioact Mater. 2021;6(1):137-145. doi: 10.1016/j.bioactmat.2020.07.007
- Zhu H, Wang J, Wang S, et al. Additively manufactured bioceramic scaffolds based on triply periodic minimal surfaces for bone regeneration. J Tissue Eng. 2024;15:20417314241244997. doi: 10.1177/20417314241244997
- Ahn J-H, Kim J, Han G, et al. 3D-printed biodegradable composite scaffolds with significantly enhanced mechanical properties via the combination of binder jetting and capillary rise infiltration process. Addit Manufactur. 2021;41: 101988. doi: 10.1016/j.addma.2021.101988
- LeGeros RZ, Lin S, Rohanizadeh R, Mijares D, LeGeros JP. Biphasic calcium phosphate bioceramics: preparation, properties and applications. J Mater Sci Mater Med. 2003;14(3):201-209. doi: 10.1023/A:1022872421333
- Ishack S, Mediero A, Wilder T, Ricci JL, Cronstein BN. Bone regeneration in critical bone defects using three-dimensionally printed β-tricalcium phosphate/ hydroxyapatite scaffolds is enhanced by coating scaffolds with either dipyridamole or BMP-2. J Biomed Mater Res B Appl Biomater. 2017;105(2):366-375. doi: 10.1002/jbm.b.33561
- Witek L, Shi Y, Smay J. Controlling calcium and phosphate ion release of 3D printed bioactive ceramic scaffolds: an in vitro study. J Adv Ceramics. 2017;6(2):157-164. doi: 10.1007/s40145-017-0228-2
- Liu B, Lun DX. Current application of β-tricalcium phosphate composites in orthopaedics. Orthop Surg. 2012;4(3):139-144. doi: 10.1111/j.1757-7861.2012.00189.x
- Fariña NM, Guzón FM, Peña ML, Cantalapiedra AG. In vivo behaviour of two different biphasic ceramic implanted in mandibular bone of dogs. J Mater Sci Mater Med. 2008;19(4):1565-1573. doi: 10.1007/s10856-008-3400-y
- Wang Y, Chen S, Liang H, Liu Y, Bai J, Wang M. Digital light processing (DLP) of nano biphasic calcium phosphate bioceramic for making bone tissue engineering scaffolds. Ceram Int. 2022;48(19, Part A):27681-27692. doi: 10.1016/j.ceramint.2022.06.067
- Chen Z, Li Z, Li J, et al. 3D printing of ceramics: a review. J Euro Ceram Soc. 2019;39(4):661-687. doi: 10.1016/j.jeurceramsoc.2018.11.013
- Melchels FPW, Feijen J, Grijpma DW. A review on stereolithography and its applications in biomedical engineering. Biomaterials 2010;31(24):6121-6130. doi: 10.1016/j.biomaterials.2010.04.050
- Zhang J, Hu Q, Wang S, Tao J, Gou M. Digital light processing based three-dimensional printing for medical applications. Int J Bioprint. 2020;6(1):242. doi: 10.18063/ijb.v6i1.242
- Monfared MH, Nemati A, Loghman F, et al. A deep insight into the preparation of ceramic bone scaffolds utilizing robocasting technique. Ceram Int. 2022;48(5):5939-5954. doi: 10.1016/j.ceramint.2021.11.268
- Dong Z, Zhao X. Application of TPMS structure in bone regeneration. Eng Regeneration. 2021;2:154-162. doi: 10.1016/j.engreg.2021.09.004
- Shi J, Zhu L, Li L, Li Z, Yang J, Wang X. A TPMS-based method for modeling porous scaffolds for bionic bone tissue engineering. Sci Rep. 2018;8(1):7395. doi: 10.1038/s41598-018-25750-9
- Toosi S, Javid-Naderi MJ, Tamayol A, Ebrahimzadeh MH, Yaghoubian S, Mousavi Shaegh SA. Additively manufactured porous scaffolds by design for treatment of bone defects. Front Bioeng Biotechnol. 2023;11:1252636. doi: 10.3389/fbioe.2023.1252636
- Zhu H, Lin Z, Luan Q, et al. Angiogenesis-promoting composite TPMS bone tissue engineering scaffold for mandibular defect regeneration. Int J Bioprint. 2023;10(1):0153. doi: 10.36922/ijb.0153
- Thanasrisuebwong P, Kiattavorncharoen S, Deeb GR, Bencharit S. Implant site preparation application of injectable platelet-rich fibrin for vertical and horizontal bone regeneration: a clinical report. J Oral Implantol. 2022;48(1):43-50. doi: 10.1563/aaid-joi-D-20-00031
- Chenchev IL, Ivanova VV, Neychev DZ, Cholakova RB. Application of platelet-rich fibrin and injectable platelet-rich fibrin in combination of bone substitute material for alveolar ridge augmentation – a case report. Folia Medica. 2017;59(3):362-366. doi: 10.1515/folmed-2017-0044
- Lorenz J, Al-Maawi S, Sader R, Ghanaati S. Individualized titanium mesh combined with platelet-rich fibrin and deproteinized bovine bone: a new approach for challenging augmentation. J Oral Implantol. 2018;44(5): 345-351. doi: 10.1563/aaid-joi-D-18-00049
- Dong Y, Zhou X, Zhang N. CCN1 inhibition affects the function of endothelial progenitor cells under high-glucose condition. Adv Clin Exp Med. 2024;33(6):619-631. doi: 10.17219/acem/170998
- Pan C, Hu T, Liu P, et al. BM-MSCs display altered gene expression profiles in B-cell acute lymphoblastic leukemia niches and exert pro-proliferative effects via overexpression of IFI6. J Transl Med. 2023;21(1):593. doi: 10.1186/s12967-023-04464-1
- Gonçalves TL, de Araújo LP, Pereira Ferrer V. Tamoxifen as a modulator of CXCL12-CXCR4-CXCR7 chemokine axis: A breast cancer and glioblastoma view. Cytokine. 2023;170:156344. doi: 10.1016/j.cyto.2023.156344
- Olmo N, Martín AI, Salinas AJ, Turnay J, Vallet-Regí M, Lizarbe MA. Bioactive sol–gel glasses with and without a hydroxycarbonate apatite layer as substrates for osteoblast cell adhesion and proliferation. Biomaterials 2003;24(20):3383-3393. doi: 10.1016/S0142-9612(03)00200-X
- Li Y, Dai X, Bai Y, et al. Electroactive BaTiO3 nanoparticle-functionalized fibrous scaffolds enhance osteogenic differentiation of mesenchymal stem cells. Int J Nanomedicine. 2017;12:4007-4018. doi: 10.2147/IJN.S135605
- Shi W, Sun M, Hu X, et al. Structurally and functionally optimized silk-fibroin-gelatin scaffold using 3d printing to repair cartilage injury in vitro and in vivo. Adv Mater (Deerfield Beach, Fla). 2017;29(29):10.1002/adma.201701089. doi: 10.1002/adma.201701089
- Li Y, Li J, Jiang S, et al. The design of strut/TPMS-based pore geometries in bioceramic scaffolds guiding osteogenesis and angiogenesis in bone regeneration. Mater Today Bio. 2023;20:100667. doi: 10.1016/j.mtbio.2023.100667
- Li M, Jiang J, Liu W, et al. Bioadaptable bioactive glass-β- tricalcium phosphate scaffolds with TPMS-gyroid structure by stereolithography for bone regeneration. J Mater Sci Technol. 2023;155:54-65. doi: 10.1016/j.jmst.2023.01.025
- Wang J, Li W, He X, Li S, Pan H, Yin L. Injectable platelet-rich fibrin positively regulates osteogenic differentiation of stem cells from implant hole via the ERK1/2 pathway. Platelets. 2023;34(1):2159020. doi: 10.1080/09537104.2022.2159020
- Majidinia M, Sadeghpour A, Yousefi B. The roles of signaling pathways in bone repair and regeneration. J Cell Physiol. 2018;233(4):2937-2948. doi: 10.1002/jcp.26042
- Daigang L, Jining Q, Jinlai L, et al. LPS-stimulated inflammation inhibits BMP-9-induced osteoblastic differentiation through crosstalk between BMP/MAPK and Smad signaling. Exp Cell Res. 2016;341(1):54-60. doi: 10.1016/j.yexcr.2016.01.009
- Liu C, Weng Y, Yuan T, et al. CXCL12/CXCR4 signal axis plays an important role in mediating bone morphogenetic protein 9-induced osteogenic differentiation of mesenchymal stem cells. Int J Med Sci.2013;10(9):1181-1192. doi: 10.7150/ijms.6657
- Mousavi A. CXCL12/CXCR4 signal transduction in diseases and its molecular approaches in targeted-therapy. Immunol Lett. 2020;217:91-115. doi: 10.1016/j.imlet.2019.11.007
- Xiao G, Jiang D, Thomas P, et al. MAPK pathways activate and phosphorylate the osteoblast-specific transcription factor, Cbfa1. J Biological Chem. 2000;275(6): 4453-4459. doi: 10.1074/jbc.275.6.4453
- Ge C, Xiao G, Jiang D, et al. Identification and functional characterization of ERK/MAPK phosphorylation sites in the Runx2 transcription factor. J Biological Chem. 2009;284(47):32533-32543. doi: 10.1074/jbc.M109.040980
- Fu L, Peng S, Wu W, Ouyang Y, Tan D, Fu X. LncRNA HOTAIRM1 promotes osteogenesis by controlling JNK/ AP-1 signalling-mediated RUNX2 expression. J Cell Mol Med. 2019;23(11):7517-7524. doi: 10.1111/jcmm.14620
- Zenz R, Wagner EF. Jun signalling in the epidermis: from developmental defects to psoriasis and skin tumors. Int J Biochem Cell Biol. 2006;38(7):1043-1049. doi: 10.1016/j.biocel.2005.11.011
- Zenz R, Eferl R, Scheinecker C, et al. Activator protein 1 (Fos/Jun) functions in inflammatory bone and skin disease. Arthritis Res Ther. 2008;10(1):201. doi: 10.1186/ar2338
- Vleugel MM, Greijer AE, Bos R, van der Wall E, van Diest PJ. c-Jun activation is associated with proliferation and angiogenesis in invasive breast cancer. Hum Pathol. 2006;37(6):668-674. doi: 10.1016/j.humpath.2006.01.022
- Folkman J. Angiogenesis and c-Jun. J Natl Cancer Inst. 2004;96(9):644. doi: 10.1093/jnci/djh148
- Liekens S, Schols D, Hatse S. CXCL12-CXCR4 axis in angiogenesis, metastasis and stem cell mobilization. Curr Pharm Des. 2010;16(35):3903-3920. doi: 10.2174/138161210794455003
- Mirshahi F, Pourtau J, Li H, et al. SDF-1 activity on microvascular endothelial cells: consequences on angiogenesis in in vitro and in vivo models. Thromb Res. 2000;99(6):587-594. doi: 10.1016/s0049-3848(00)00292-9
- He C, Zhang H, Wang B, He J, Ge G. SDF-1/CXCR4 axis promotes the growth and sphere formation of hypoxic breast cancer SP cells by c-Jun/ABCG2 pathway. Biochem Biophys Res Commun. 2018;505(2):593-599. doi: 10.1016/j.bbrc.2018.09.130
- Lue H, Dewor M, Leng L, Bucala R, Bernhagen J. Activation of the JNK signalling pathway by macrophage migration inhibitory factor (MIF) and dependence on CXCR4 and CD74. Cell Signal. 2011;23(1): 135-144. doi: 10.1016/j.cellsig.2010.08.013
- Strassheim D, Karoor V, Nijmeh H, et al. c-Jun, Foxo3a, and c-Myc transcription factors are key regulators of ATP-mediated angiogenic responses in pulmonary artery vasa vasorum endothelial cells. Cells. 2020;9(2):416. doi: 10.3390/cells9020416
- Ma J, Zhang L, Han W, et al. Activation of JNK/c-Jun is required for the proliferation, survival, and angiogenesis induced by EET in pulmonary artery endothelial cells. J Lipid Res. 2012;53(6):1093-1105. doi: 10.1194/jlr.M024398
- Huang J, Han Q, Cai M, et al. Effect of angiogenesis in bone tissue engineering. Ann Biomed Eng. 2022;50(8):898-913. doi: 10.1007/s10439-022-02970-9
- Chia HN, Wu BM. Recent advances in 3D printing of biomaterials. J Biol Eng. 2015;9:4. doi: 10.1186/s13036-015-0001-4
- Eftekhari H, Jahandideh A, Asghari A, Akbarzadeh A, Hesaraki S. Assessment of polycaprolacton (PCL) nanocomposite scaffold compared with hydroxyapatite (HA) on healing of segmental femur bone defect in rabbits. Artif Cells Nanomed Biotechnol. 2017;45(5):961-968. doi: 10.1080/21691401.2016.1198360
- Wang S, Lu J, You Q, Huang H, Chen Y, Liu K. The mTOR/ AP-1/VEGF signaling pathway regulates vascular endothelial cell growth. Oncotarget. 2016;7(33):53269-53276. doi: 10.18632/oncotarget.10756
- Lee C-C, Chen S-C, Tsai S-C, et al. Hyperbaric oxygen induces VEGF expression through ERK, JNK and c-Jun/ AP-1 activation in human umbilical vein endothelial cells. J Biomed Sci. 2006;13(1):143-156. doi: 10.1007/s11373-005-9037-7
- Shin M, Pandya M, Espinosa K, et al. Istradefylline mitigates age-related hearing loss in C57BL/6J mice. Int J Mol Sci. 2021;22(15):8000. doi: 10.3390/ijms22158000
- Mercado-Pagán ÁE, Stahl AM, Shanjani Y, Yang Y. Vascularization in bone tissue engineering constructs. Ann Biomed Eng. 2015;43(3):718-729. doi: 10.1007/s10439-015-1253-3