Increased stiffness of extracellular matrix enhanced chemoresistance in 3D-bioprinted ovarian cancer model
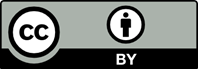
Ovarian cancer is a gynecological malignancy with a high mortality rate. The ovarian cancer microenvironment is a crucial factor affecting the overall and progression-free survival rates of patients with ovarian cancer. The biophysical factors of the tumor microenvironment, such as stiffness, can affect the gene expression and behavior of tumor cells. In this study, we utilized 3D bioprinting technology to construct ovarian cancer tumor models with varying levels of stiffness in vitro to investigate the effect of extracellular matrix stiffness on drug resistance of tumor cells. Our findings indicate that increasing the stiffness of extracellular matrix can attenuate the sensitivity of tumor cells to chemotherapeutic agents. Additionally, the increased stiffness of 3D tumor model may promote malignant phenotypes, such as tumor stemness and tumor progression.
- Alkmin, S., Patankar, M. S. & Campagnola, P. J., 2022, Assessing the roles of collagen fiber morphology and matrix stiffness on ovarian cancer cell migration dynamics using multiphoton fabricated orthogonal image-based models. Acta Biomater, 153:342-354. doi: 10.1016/j.actbio.2022.09.037
- Lheureux, S., Braunstein, M. & Oza, A. M., 2019, Epithelial ovarian cancer: Evolution of management in the era of precision medicine. CA Cancer J Clin, 69(4):280-304. doi: 10.3322/caac.21559
- Vescarelli, E., Gerini, G., Megiorni, F. et al., 2020, MiR- 200c sensitizes Olaparib-resistant ovarian cancer cells by targeting Neuropilin 1. J Exp Clin Cancer Res, 39(1):3. doi: 10.1186/s13046-019-1490-7
- Wang, M., Zhou, J., Zhang, L. et al., 2019, Surgical treatment of ovarian cancer liver metastasis. Hepatobiliary Surg Nutr, 8(2):129-137. doi: 10.21037/hbsn.2018.12.06
- Herrera, F. G., Irving, M., Kandalaft, L. E. et al., 2019, Rational combinations of immunotherapy with radiotherapy in ovarian cancer. Lancet Oncol, 20(8):e417-e433. doi: 10.1016/s1470-2045(19)30401-2
- Raghavan, S., Mehta, P., Ward, M. R. et al., 2017, Personalized Medicine-Based Approach to Model Patterns of Chemoresistance and Tumor Recurrence Using Ovarian Cancer Stem Cell Spheroids. Clin Cancer Res, 23(22): 6934-6945. doi: 10.1158/1078-0432.CCR-17-0133
- Lee, A., Hudson, A. R., Shiwarski, D. J. et al., 2019, 3D bioprinting of collagen to rebuild components of the human heart. SCIENCE, 365(6452):482-487. doi: 10.1126/science.aav9051
- Lee, S., Sani, E. S., Spencer, A. R. et al., 2020, Human- Recombinant-Elastin-Based Bioinks for 3D Bioprinting of Vascularized Soft Tissues. Adv Mater, 32(45):e2003915. doi: 10.1002/adma.202003915
- Li, C., Jiang, Z. & Yang, H., 2022, Advances in 3D bioprinting technology for liver regeneration. Hepatobiliary Surg Nutr, 11(6):917-919. doi: 10.21037/hbsn-22-531
- Zhang, J., Yang, H. & Yang, H., 2022, Highlights of constructing liver-relevant in vitro models with 3D bioprinting. Hepatobiliary Surg Nutr, 11(6):896-898. doi: 10.21037/hbsn-22-486
- Neufeld, L., Yeini, E., Pozzi, S. et al., 2022, 3D bioprinted cancer models: from basic biology to drug development. Nat Rev Cancer, 22(12):679-692. doi: 10.1038/s41568-022-00514-w
- Tang, M., Xie, Q., Gimple, R. C. et al., 2020, Three-dimensional bioprinted glioblastoma microenvironments model cellular dependencies and immune interactions. Cell Res, 30(10):833-853. doi: 10.1038/s41422-020-0338-1
- Park, W., Bae, M., Hwang, M. et al., 2021, 3D Cell-Printed Hypoxic Cancer-on-a-Chip for Recapitulating Pathologic Progression of Solid Cancer. J Vis Exp, (167). doi: 10.3791/61945
- Hong, S. & Song, J. M., 2022, 3D bioprinted drug-resistant breast cancer spheroids for quantitative in situ evaluation of drug resistance. Acta Biomater, 138:228-239. doi: 10.1016/j.actbio.2021.10.031
- Mei, Y., He, C., Gao, C. et al., 2021, 3D-Printed Degradable Anti-Tumor Scaffolds for Controllable Drug Delivery. Int J Bioprint, 7(4):418. doi: 10.18063/ijb.v7i4.418
- Yi, H. G., Jeong, Y. H., Kim, Y. et al., 2019, A bioprinted human-glioblastoma-on-a-chip for the identification of patient-specific responses to chemoradiotherapy. Nat Biomed Eng, 3(7):509-519. doi: 10.1038/s41551-019-0363-x
- Shim, I. K., Yi, H. J., Yi, H. G. et al., 2017, Locally-applied 5-fluorouracil-loaded slow-release patch prevents pancreatic cancer growth in an orthotopic mouse model. Oncotarget, 8(25):40140-40151. doi: 10.18632/oncotarget.17370
- Hirschhaeuser, F., Menne, H., Dittfeld, C. et al., 2010, Multicellular tumor spheroids: an underestimated tool is catching up again. J Biotechnol, 148(1):3-15. doi: 10.1016/j.jbiotec.2010.01.012
- Abhinand, C. S., Raju, R., Soumya, S. J. et al., 2016, VEGF-A/ VEGFR2 signaling network in endothelial cells relevant to angiogenesis. J Cell Commun Signal, 10(4):347-354. doi: 10.1007/s12079-016-0352-8
- Sun, L., Yang, H., Wang, Y. et al., 2020, Application of a 3D Bioprinted Hepatocellular Carcinoma Cell Model in Antitumor Drug Research. Front Oncol, 10:878. doi: 10.3389/fonc.2020.00878
- Mao, S., He, J., Zhao, Y. et al., 2020, Bioprinting of patient-derived in vitro intrahepatic cholangiocarcinoma tumor model: establishment, evaluation and anti-cancer drug testing. Biofabrication, 12(4):045014. doi: 10.1088/1758-5090/aba0c3
- Xie, F., Sun, L., Pang, Y. et al., 2021, Three-dimensional bio-printing of primary human hepatocellular carcinoma for personalized medicine. BIOMATERIALS, 265:120416. doi: 10.1016/j.biomaterials.2020.120416
- Yang, H., Sun, L., Pang, Y. et al., 2021, Three-dimensional bioprinted hepatorganoids prolong survival of mice with liver failure. GUT, 70(3):567-574. doi: 10.1136/gutjnl-2019-319960
- Tebon, P. J., Wang, B., Markowitz, A. L. et al., 2023, Drug screening at single-organoid resolution via bioprinting and interferometry. Nat Commun, 14(1):3168. doi: 10.1038/s41467-023-38832-8
- Mazzocchi, A., Soker, S. & Skardal, A., 2019, 3D bioprinting for high-throughput screening: Drug screening, disease modeling, and precision medicine applications. Appl Phys Rev, 6(1). doi: 10.1063/1.5056188
- Wang, M., Zhao, J., Zhang, L. et al., 2017, Role of tumor microenvironment in tumorigenesis. J Cancer, 8(5):761-773. doi: 10.7150/jca.17648
- Yang, M., Lu, J., Zhang, G. et al., 2021, CXCL13 shapes immunoactive tumor microenvironment and enhances the efficacy of PD-1 checkpoint blockade in high-grade serous ovarian cancer. J Immunother Cancer, 9(1). doi: 10.1136/jitc-2020-001136
- Ding, Q., Dong, S., Wang, R. et al., 2020, A nine-gene signature related to tumor microenvironment predicts overall survival with ovarian cancer. Aging (Albany NY), 12(6):4879-4895. doi: 10.18632/aging.102914
- Di Modugno, F., Colosi, C., Trono, P. et al., 2019, 3D models in the new era of immune oncology: focus on T cells, CAF and ECM. J Exp Clin Cancer Res, 38(1):117. doi: 10.1186/s13046-019-1086-2
- Hinshaw, D. C. & Shevde, L. A., 2019, The Tumor Microenvironment Innately Modulates Cancer Progression. Cancer Res, 79(18):4557-4566. doi: 10.1158/0008-5472.CAN-18-3962
- Elhanani, O., Ben-Uri, R. & Keren, L., 2023, Spatial profiling technologies illuminate the tumor microenvironment. CANCER CELL, 41(3):404-420. doi: 10.1016/j.ccell.2023.01.010
- Wei, X., Lou, H., Zhou, D. et al., 2021, TAGLN mediated stiffness-regulated ovarian cancer progression via RhoA/ ROCK pathway. J Exp Clin Cancer Res, 40(1):292. doi: 10.1186/s13046-021-02091-6
- Kim, J., Jang, J. & Cho, D. W., 2021, Controlling Cancer Cell Behavior by Improving the Stiffness of Gastric Tissue- Decellularized ECM Bioink With Cellulose Nanoparticles. Front Bioeng Biotechnol, 9:605819. doi: 10.3389/fbioe.2021.605819
- Tang, M., Tiwari, S. K., Agrawal, K. et al., 2021, Rapid 3D Bioprinting of Glioblastoma Model Mimicking Native Biophysical Heterogeneity. Small, 17(15):e2006050. doi: 10.1002/smll.202006050
- Yang, X., Wang, G., Huang, X. et al., 2020, RNA-seq reveals the diverse effects of substrate stiffness on epidermal ovarian cancer cells. Aging (Albany NY), 12(20):20493-20511. doi: 10.18632/aging.103906
- Pietilä, E. A., Gonzalez-Molina, J., Moyano-Galceran, L. et al., 2021, Co-evolution of matrisome and adaptive adhesion dynamics drives ovarian cancer chemoresistance. Nat Commun, 12(1):3904. doi: 10.1038/s41467-021-24009-8
- Ouyang, L., Yao, R., Zhao, Y. et al., 2016, Effect of bioink properties on printability and cell viability for 3D bioplotting of embryonic stem cells. Biofabrication, 8(3):035020. doi: 10.1088/1758-5090/8/3/035020
- Li, C., Jin, B., Sun, H. et al., 2022, Exploring the function of stromal cells in cholangiocarcinoma by three-dimensional bioprinting immune microenvironment model. Front Immunol, 13:941289. doi: 10.3389/fimmu.2022.941289
- Singha, B., Gatla, H. R., Manna, S. et al., 2014, Proteasome inhibition increases recruitment of IκB kinase β (IKKβ), S536P-p65, and transcription factor EGR1 to interleukin-8 (IL-8) promoter, resulting in increased IL-8 production in ovarian cancer cells. J Biol Chem, 289(5):2687-2700. doi: 10.1074/jbc.M113.502641
- Javellana, M., Eckert, M. A., Heide, J. et al., 2022, Neoadjuvant Chemotherapy Induces Genomic and Transcriptomic Changes in Ovarian Cancer. Cancer Res, 82(1):169-176. doi: 10.1158/0008-5472.CAN-21-1467
- du Manoir, S., Delpech, H., Orsetti, B. et al., 2022, In high-grade ovarian carcinoma, platinum-sensitive tumor recurrence and acquired-resistance derive from quiescent residual cancer cells that overexpress CRYAB, CEACAM6, and SOX2. J Pathol, 257(3):367-378. doi: 10.1002/path.5896
- Yamawaki, K., Mori, Y., Sakai, H. et al., 2021, Integrative analyses of gene expression and chemosensitivity of patient-derived ovarian cancer spheroids link G6PD-driven redox metabolism to cisplatin chemoresistance. Cancer Lett, 521:29-38. doi: 10.1016/j.canlet.2021.08.018
- Kang, Y., Nagaraja, A. S., Armaiz-Pena, G. N. et al., 2016, Adrenergic Stimulation of DUSP1 Impairs Chemotherapy Response in Ovarian Cancer. Clin Cancer Res, 22(7):1713- 1724. doi: 10.1158/1078-0432.Ccr-15-1275
- Andreoli, M., Persico, M., Kumar, A. et al., 2014, Identification of the first inhibitor of the GBP1:PIM1 interaction. Implications for the development of a new class of anticancer agents against paclitaxel resistant cancer cells. J Med Chem, 57(19):7916-7932. doi: 10.1021/jm5009902
- Xiao, Y., Lai, Y., Yu, Y. et al., 2021, The Exocrine Differentiation and Proliferation Factor (EXDPF) Gene Promotes Ovarian Cancer Tumorigenesis by Up-Regulating DNA Replication Pathway. Front Oncol, 11:669603. doi: 10.3389/fonc.2021.669603
- Zhang, Y., Qiu, J. G., Jia, X. Y. et al., 2023, METTL3- mediated N6-methyladenosine modification and HDAC5/ YY1 promote IFFO1 downregulation in tumor development and chemo-resistance. Cancer Lett, 553:215971. doi: 10.1016/j.canlet.2022.215971
- Zaid, T. M., Yeung, T. L., Thompson, M. S. et al., 2013, Identification of FGFR4 as a potential therapeutic target for advanced-stage, high-grade serous ovarian cancer. Clin Cancer Res, 19(4):809-820. doi: 10.1158/1078-0432.Ccr-12-2736
- Brancato, V., Oliveira, J. M., Correlo, V. M. et al., 2020, Could 3D models of cancer enhance drug screening? BIOMATERIALS, 232:119744. doi: 10.1016/j.biomaterials.2019.119744
- Zanoni, M., Cortesi, M., Zamagni, A. et al., 2020, Modeling neoplastic disease with spheroids and organoids. J Hematol Oncol, 13(1): 97. doi: 10.1186/s13045-020-00931-0
- Fan, Y., Sun, Q., Li, X. et al., 2021, Substrate Stiffness Modulates the Growth, Phenotype, and Chemoresistance of Ovarian Cancer Cells. Front Cell Dev Biol, 9:718834. doi: 10.3389/fcell.2021.718834
- Paradiso, F., Lenna, S., Gazze, S. A. et al., 2022, Mechanomimetic 3D Scaffolds as a Humanized In Vitro Model for Ovarian Cancer. Cells, 11(5). doi: 10.3390/cells11050824
- Barroso, M., Chheda, M. G., Clevers, H. et al., 2022, A path to translation: How 3D patient tumor avatars enable next generation precision oncology. CANCER CELL, 40(12):1448-1453. doi: 10.1016/j.ccell.2022.09.017
- Kim, J., Koo, B. K. & Knoblich, J. A., 2020, Human organoids: model systems for human biology and medicine. Nat Rev Mol Cell Biol, 21(10):571-584. doi: 10.1038/s41580-020-0259-3
- Zanella, E. R., Grassi, E. & Trusolino, L., 2022, Towards precision oncology with patient-derived xenografts. Nat Rev Clin Oncol, 19(11):719-732. doi: 10.1038/s41571-022-00682-6
- Zhuo, J., Su, R., Tan, W. et al., 2020, The ongoing trends of patient-derived xenograft models in oncology. Cancer Commun (Lond), 40(11):559-563. doi: 10.1002/cac2.12096
- Zong, X., Wang, W., Ozes, A. et al., 2020, EZH2-Mediated Downregulation of the Tumor Suppressor DAB2IP Maintains Ovarian Cancer Stem Cells. Cancer Res, 80(20):4371-4385. doi: 10.1158/0008-5472.Can-20-0458
- Sacks Suarez, J., Gurler Main, H., Muralidhar, G. G. et al., 2019, CD44 Regulates Formation of Spheroids and Controls Organ-Specific Metastatic Colonization in Epithelial Ovarian Carcinoma. Mol Cancer Res, 17(9):1801-1814. doi: 10.1158/1541-7786.Mcr-18-1205
- Zhao, Y., He, M., Cui, L. et al., 2020, Chemotherapy exacerbates ovarian cancer cell migration and cancer stem cell-like characteristics through GLI1. Br J Cancer, 122(11):1638-1648. doi: 10.1038/s41416-020-0825-7
- Wang, Y., Niu, X. L., Qu, Y. et al., 2010, Autocrine production of interleukin-6 confers cisplatin and paclitaxel resistance in ovarian cancer cells. Cancer Lett, 295(1):110-123. doi: 10.1016/j.canlet.2010.02.019
- Klinghammer, K., Walther, W. & Hoffmann, J., 2017, Choosing wisely - Preclinical test models in the era of precision medicine. Cancer Treat Rev, 55:36-45. doi: 10.1016/j.ctrv.2017.02.009