Optimizing cell deposition for inkjet-based bioprinting
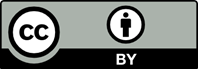
Although inkjet-based bioprinting enables precise drop-on-demand cell deposition within three-dimensional (3D) tissue constructs and facilitates critical cell–cell and cell–matrix interactions, it faces challenges such as poor cell homogeneity and low cell viability. To date, there is a lack of comprehensive review papers addressing the optimization of cell deposition in inkjet-based bioprinting. This review aims to fill that gap by providing an overview of various critical aspects in bioprinting, ranging from bio-ink properties to the impact of printed droplets. The bio-ink section begins by exploring how cells influence the physical properties of bio-inks and emphasizes the significance of achieving cell homogeneity within bio-inks to ensure consistent and reliable printing. The discussion then delves into inkjet-based printing chambers (thermal and piezoelectric), the effect of shear stress on printed cells, droplet formation dynamics, the influence of polymer-based and cell-laden droplets on the underlying substrate surface, and the dynamics of droplet impact. Beyond droplet formation and impact, the review highlights the importance of biophysical and biological cues within 3D hydrogel matrices for cell proliferation and differentiation. Finally, the paper highlights current and potential applications, with a specific focus on skin and lung tissue engineering using inkjet-based bioprinting techniques, and provides insights into the emerging role of machine learning in optimizing the cell deposition process for inkjet-based bioprinting.
- Ng WL, Chua CK, Shen Y-F. Print me an organ! Why we are not there yet. Prog Polym. Sci. 2019;97:101145. doi: 10.1016/j.progpolymsci.2019.101145
- Sun W, Starly B, Daly AC, et al. The bioprinting roadmap. Biofabrication. 2020;12(2):022002. doi: 10.1088/1758-5090/ab5158
- Levato R, Jungst T, Scheuring RG, Blunk T, Groll J, Malda J, et al. From shape to function: The next step in bioprinting. Adv Mater. 2020;32(12):1906423. doi: 10.1002/adma.201906423
- Gudapati H, Dey M, Ozbolat I. A comprehensive review on droplet-based bioprinting: past, present and future. Biomaterials. Biomaterials. 2016;102:20-42. doi: 10.1016/j.biomaterials.2016.06.012
- Ng WL, Yeong WY, Naing MW. Microvalve bioprinting of cellular droplets with high resolution and consistency. Proc Int Conf Prog Addit Manuf. 2016;397-402. doi: 10.3850/2424-8967_V02-236
- Ng WL, Lee JM, Yeong WY, Naing MW. Microvalve-based bioprinting – process, bio-inks and applications. Biomaterials. Sci. 2017;5(4):632-647. doi: 10.1039/C6BM00861E
- Koch L, Deiwick A, Franke A, et al. Laser bioprinting of human induced pluripotent stem cells—the effect of printing and biomaterials on cell survival, pluripotency, and differentiation. Biofabrication. 2018;10(3):035005, 1-21. doi: 10.1088/1758-5090/aab981
- Kotlarz M, Ferreira AM, Gentile P, Russell SJ, Dalgarno K. Droplet-based bioprinting enables the fabrication of cell– hydrogel–microfibre composite tissue precursors. Bio-Des Manuf. 2022;5(3):512-528. doi: 10.1007/s42242-022-00192-5
- Ng WL, Yeong WY, Naing MW. Potential of bioprinted films for skin tissue engineering. Proceedings of the 1st International Conference on Progress in Additive Manufacturing. 2014;441- 446. doi: 10.3850/978-981-09-0446-3_065
- Ozbolat IT, Hospodiuk M. Current advances and future perspectives in extrusion-based bioprinting. Biomaterials. 2016;76:321-343. doi: 10.1016/j.biomaterials.2015.10.076
- Ng WL, Yeong WY, Naing MW. Development of polyelectrolyte chitosan-gelatin hydrogels for skin bioprinting. Procedia CIRP. 2016; 49:105-112. doi: 10.1016/j.procir.2015.09.002
- Zhuang P, Ng WL, An J, Kai Chua C, Tan LP. Layer-by-layer ultraviolet assisted extrusion-based (UAE) bioprinting of hydrogel constructs with high aspect ratio for soft tissue engineering applications. PLoS One. 2019;14(6):e0216776. doi: 10.1371/journal.pone.0216776
- Liu S, Zhang H, Ahlfeld T, et al. Evaluation of different crosslinking methods in altering the properties of extrusion-printed chitosan-based multi-material hydrogel composites. Bio-Des Manuf. 2023;6(2):150-173. doi: 10.1007/s42242-022-00194-3
- WL Ng, Yeong WY, Naing MW. Polyelectrolyte gelatin-chitosan hydrogel optimized for 3D bioprinting in skin tissue engineering. Int J Bioprint. 2016;2(1):53-62. doi: 10.18063/IJB.2016.01.009
- Ng WL, Lee JM, Zhou M, et al. Vat polymerization-based bioprinting–process, materials, applications and regulatory challenges. Biofabrication. 2020;12(2):022001. doi: 10.1088/1758-5090/ab6034
- Li W, Mille LS, Robledo JA, Uribe T, Huerta V, Zhang YS. Recent advances in formulating and processing biomaterial inks for vat polymerization‐vased 3D printing. Adv Healthc Mater. 2020;9(15):2000156. doi: 10.1002/adhm.202000156
- Klebe RJ. Cytoscribing: A method for micropositioning cells and the construction of two-and three-dimensional synthetic tissues. Exp Cell Res. 1988;179(2):362-373. doi: 10.1016/0014-4827(88)90275-3
- Roth EA, Xu T, Das M, Hickman JJ, Boland T. Inkjet printing for high-throughput cell patterning. Biomaterials. 2004;25(17):3707-3715. doi: 10.1016/j.biomaterials.2003.10.052
- Xu T, Jin J, Gregory C, Hickman JJ, Boland T. Inkjet printing of viable mammalian cells. Biomaterials. 2005;26(1):93-99. doi: 10.1016/j.biomaterials.2004.04.011
- Nakamura M, Kobayashi A, Takagi F, et al. Biocompatible inkjet printing technique for designed seeding of individual living cells. Tissue Eng. 2005;11(11-12):1658-1666. doi: 10.1089/ten.2005.11.1658
- Xu T, Rohozinski J, Zhao W, Moorefield EC, Atala A, Yoo JJ. Inkjet-mediated gene transfection into living cells combined with targeted delivery. Tissue Eng Part A. 2009;15(1):95-101. doi: 10.1089/ten.tea.2008.0095
- Parsa S, Gupta M, Loizeau F, Cheung KC. Effects of surfactant and gentle agitation on inkjet dispensing of living cells, Biofabrication. 2010;2(2):025003.
- Xu C, Zhang M, Huang Y, Ogale A, Fu J, Markwald RR. Study of droplet formation process during drop-on-demand inkjetting of living cell-laden bioink. Langmuir. 2014;30(30):9130-9138. doi: 10.1021/la501430x
- Schoendube J, Wright D, Zengerle R, Koltay P. Single-cell printing based on impedance detection. Biomicrofluidics. 2015;9(1).
- Ng WL, Huang X, Shkolnikov V, et al. Controlling droplet impact velocity and droplet volume: Key factors to achieving high cell viability in sub-nanoliter droplet-based bioprinting. Int J Bioprint. 2022;8(1):424. doi: 10.18063/ijb.v8i1.424
- Huang X, Ng WL, Yeong WY. Predicting the number of printed cells during inkjet-based bioprinting process based on droplet velocity profile using machine learning approaches. J Intell Manuf. 2023;1-16. doi: 10.1007/s10845-023-02167-4
- Rutgers IR. Relative viscosity of suspensions of rigid spheres in newtonian liquids. Rheol Acta. 1962;2(3):202-210. doi: 10.1007/BF01983952
- Hsueh C, Wei W. Analyses of effective viscosity of suspensions with deformable polydispersed spheres. J Phys D: Appl Phys. 2009;42(7):075503. doi: 10.1088/0022-3727/42/7/075503
- A. Einstein. Eine neue bestimmung der Moleküldimensionen. Annalen der Physik. 1906;324(2):289-306. doi: 10.1002/andp.19063240204
- Taylor GI. The viscosity of a fluid containing small drops of another fluid, proceedings of the royal society of london. Series A. Containing Pap of a Math & Phys Character. 1932;138(834):41-48. doi: 10.1098/rspa.1932.0169
- Wang K, Sun X, Zhang Y, et al. Characterization of cytoplasmic viscosity of hundreds of single tumour cells based on micropipette aspiration. R Soc Open Sci. 2019;6(3):181707. doi: 10.1098/rsos.181707
- Phan-Thien N, Pham D. Differential multiphase models for polydispersed suspensions and particulate solids. J Nonnewton Fluid Mech. 1997;72(2-3):305-318. doi: 10.1016/S0377-0257(97)90002-1
- Shi Y, Ryu DD, Ballica R. Rheological properties of mammalian cell culture suspensions: Hybridoma and HeLa cell lines. Biotechnol Bioeng. 1993;41(7):745-754. doi: 10.1002/bit.260410709
- Pepper ME, Seshadri V, Burg TC, Burg KJL, Groff RE. Characterizing the effects of cell settling on bioprinter output. Biofabrication. 2012;4(1):011001. doi: 10.1088/1758-5082/4/1/011001
- Wang Z, Belovich JM. A simple apparatus for measuring cell settling velocity. Biotechnol Progr. 2010;26(5):1361-1366. doi: 10.1002/btpr.432
- Sendekie ZB, Bacchin P. Colloidal jamming dynamics in microchannel bottlenecks. Langmuir. 2016;32(6):1478-1488. doi: 10.1021/acs.langmuir.5b04218
- Dersoir B, de Saint Vincent MR, Abkarian M, Tabuteau H. Clogging of a single pore by colloidal particles. Microfluid Nanofluid. 2015;19(4):953-961. doi: 10.1007/s10404-015-1624-y
- Ng WL, Yeong WY, Naing MW. Polyvinylpyrrolidone-based bio-ink improves cell viability and homogeneity during drop-on-demand printing. Materials. 2017;10(2):190, 1-12. doi: 10.3390/ma10020190
- Xu H, Liu J, Zhang Z, et al. Cell sedimentation during 3D bioprinting: a mini review. Bio-Des Manuf. 2022;5(3): 617-626. doi: 10.1007/s42242-022-00183-6
- Liu J, Shahriar M, Xu H, Xu C. Cell-laden bioink circulation-assisted inkjet-based bioprinting to mitigate cell sedimentation and aggregation. Biofabrication. 2022;14(4):045020. doi: 10.1088/1758-5090/ac8fb7
- Allen RR, Meyer JD, Knight WR. Thermodynamics and hydrodynamics of thermal ink jets. Hewlett-Packard J. 1985;36(5):21-27 doi: hparchive.com/Journals/HPJ-1985-05.pdf
- Morita N, Hiratsuka M, Hamazaki T, et al. Pulse and temperature control of thermal ink jet printheads without a heater passivation layer. J Imaging Sci Technol. 2008;52(2):20503-1-20503-5. doi: 10.2352/J.ImagingSci.Technol.(2008)52:2(020503)
- Skripov VP. Metastable Liquids. New York: Wiley;1974.
- Okuyama K, Tsukahara S, Morita N, Iida Y. Transient behavior of boiling bubbles generated on the small heater of a thermal ink jet printhead. Exp Therm Fluid Sci. 2004;28(8):825-834. doi: 10.1016/j.expthermflusci.2003.12.018
- Meyer J. Bubble Growth and Nucleation Properties in Thermal Ink-jet Printing Technology. Digest of Technical Papers - SID International Symposium. 1986; (17) 101-104.
- Chang L. Effects of kogation on the operation and lifetime of bubble jet thin-film devices. Denshi Shashin Gakkaishi(Electrophotography). 1989;28(1):2-8. doi: 10.11370/isjepj.28.2
- Wijshoff H. The dynamics of the piezo inkjet printhead operation. Phys Rep. 2010;491(4-5):77-177. doi: 10.1016/j.physrep.2010.03.003
- Sharp MK, Mohammad SF. Scaling of hemolysis in needles and catheters, Ann Biomed Eng. 1998;26:788-797. doi: 10.1114/1.65
- Grigioni M, Daniele C, Morbiducci U, D’Avenio Giuseppe, Di Benedetto G, Barbaro V. The power‐law mathematical model for blood damage prediction: analytical developments and physical inconsistencies. Artif Organs. 2004;28(5):467-475. doi: 10.1111/j.1525-1594.2004.00015.x
- Grigioni M, Morbiducci U, D’Avenio G, Di Benedetto G, Del Gaudio C. A novel formulation for blood trauma prediction by a modified power-law mathematical model. Biomech Model Mechanobio. 2005;4:249-260. doi: 10.1007/s10237-005-0005-y
- Faghih MM, Sharp MK. Modeling and prediction of flow-induced hemolysis: A review. Biomech Model Mechanobio. 2019;18:845-881. doi: 10.1007/s10237-019-01137-1
- Stolberg S, McCloskey KE. Can shear stress direct stem cell fate? Biotechnol Progr. 2009;25(1):10-19. doi: 10.1002/btpr.124
- Smith C, Greenfield P, Randerson D. Shear sensitivity of three hybridoma cell lines in suspension culture. Mod Apr Anim cell tech. 1987;316-327. doi: 10.1016/B978-0-408-02732-8.50027-4
- Malek AM, Alper SL, Izumo S. Hemodynamic shear stress and its role in atherosclerosis. Jama. 1999;282(21): 2035-2042. doi: 10.1001/jama.282.21.2035
- Reneman RS, Hoeks AP. Wall shear stress as measured in vivo: consequences for the design of the arterial system. Med Biol Eng Comput. 2008;46:499-507. doi: 10.1007/s11517-008-0330-2
- Williams A, Hughes D, Nyborg W. Hemolysis near a transversely oscillating wire. Sci. 1970;169(3948):871-873. doi: 10.1126/science.169.3948.871
- Rooney JA. Hemolysis near an ultrasonically pulsating gas bubble. Sci. 1970;169(3948):869-871. doi: 10.1126/science.169.3948.869
- Forstrom RJ. A New Measure of Erythrocyte Membrane Strength: The Jet Fragility Test. [PhD thesis]. Minnesota: University of Minnesota; 1969
- Blackshear PL. Hemolysis at prosthetic surfaces. In: Hair ML, ed. Chemistry of Biosurfaces. New York: Marcel Dekker; 1972: 523-561.
- Kretzmer G, Schügerl K. Response of mammalian cells to shear stress. Appl Microbiol Biotechnol. 1991;34:613-616. doi: 10.1007/BF00167909
- Barnes JM, Nauseef JT, Henry MD. Resistance to fluid shear stress is a conserved biophysical property of malignant cells. PloS one. 2012;7(12):e50973. doi: 10.1371/journal.pone.0050973
- Lohse D. Fundamental fluid dynamics challenges in inkjet printing. Annu Rev Fluid Mech. 2022;54:349-382. doi: 10.1146/annurev-fluid-022321-114001
- Xu T, Gregory CA, Molnar P, Cui X. Viability and electrophysiology of neural cell structures generated by the inkjet printing method. Biomaterials. 2006;27(19):3580- 3588. doi: 10.1016/j.biomaterials.2006.01.048
- Yumoto M, Hemmi N, Sato N, et al. Evaluation of the effects of cell-dispensing using an inkjet-based bioprinter on cell integrity by RNA-seq analysis. Sci Rep. 2020;10(1):7158. doi: 10.1038/s41598-020-64193-z
- Furbank RJ, Morris JF. An experimental study of particle effects on drop formation. Phys Fluids. 2004;16(5): 1777-1790. doi: 10.1063/1.1691034
- Furbank RJ, Morris JF. Pendant drop thread dynamics of particle-laden liquids. Int J Multiphase Flow. 2007;33(4): 448-468. doi: 10.1016/j.ijmultiphaseflow.2006.02.021
- Rioboo R, Tropea C, Marengo M. Outcomes from a drop impact on solid surfaces. Atomization Sprays. 2001;11(2). doi: 10.1615/AtomizSpr.v11.i2.40
- Liu Y, Yan X, Wang Z. Droplet dynamics on slippery surfaces: small droplet, big impact. Biosurface and Biotribology. 2019;5(2):35-45. doi: 10.1049/bsbt.2019.0004
- Rein M. Phenomena of liquid drop impact on solid and liquid surfaces. Fluid Dyn Res. 1993;12(2):61-93. doi: 10.1016/0169-5983(93)90106-K
- Fedorchenko AI, Wang A-B. On some common features of drop impact on liquid surfaces. Phys Fluids. 2004;16(5): 1349-1365. doi: 10.1063/1.1652061
- Zou J, Wang PF, Zhang TR, Fu Xin, Ruan X. Experimental study of a drop bouncing on a liquid surface. Phys Fluids. 2011;23(4). doi: 10.1063/1.3575298
- Leng LJ. Splash formation by spherical drops, J Fluid Mech. 2001;427:73-105. doi: 10.1017/S0022112000002500
- Bach GA, Koch DL, Gopinath A. Coalescence and bouncing of small aerosol droplets. J Fluid Mech. 2004;518:157-185. doi: 10.1017/S0022112004000928
- Frith WJ, d’Haene P, Buscall R, Mewis J. Shear thickening in model suspensions of sterically stabilized particles. J Rheol. 1996;40(4):531-548. doi: 10.1122/1.550791
- German G, Bertola V. Impact of shear-thinning and yield-stress drops on solid substrates. J Phys Condens Matter 2009;21(37):375111. doi: 10.1088/0953-8984/21/37/375111
- Bertola V, Marengo M. Single drop impacts of complex fluids: a review. In: Ferrari M, Liggieri L, Miller R, eds. Drops and Bubbles in Contact with Solid Surfaces. Florida, United States: Taylor & Francis(CRC Press); 2012: 267-298.
- Bergeron V, Bonn D, Martin JY, Vovelle L. Controlling droplet deposition with polymer additives. Nature. 2000;405(6788):772-775. doi: 10.1038/35015525
- Bergeron V. Designing intelligent fluids for controlling spray applications. C R Phys. 2003;4(2):211-219. doi: 10.1016/S1631-0705(03)00043-4
- Vega E, Castrejón-Pita A. Suppressing prompt splash with polymer additives. Exp Fluids. 2017;58(5):57. doi: 10.1007/s00348-017-2341-y
- Ng WL, Huang X, Shkolnikov V, Suntornnond R. Polyvinylpyrrolidone-based bioink: Influence of bioink properties on printing performance and cell proliferation during inkjet-based bioprinting. Bio-Des Manuf. 2023;6: 676-690. doi: 10.1007/s42242-023-00245-3
- Guémas M, Marín ÁG, Lohse D. Drop impact experiments of non-Newtonian liquids on micro-structured surfaces. Soft Matter. 2012;8(41):10725-10731. doi: 10.1039/C2SM26230D
- An SM, Lee SY. Maximum spreading of a shear-thinning liquid drop impacting on dry solid surfaces. Exp Therm Fluid Sci. 2012;38:140-148. doi: 10.1016/j.expthermflusci.2011.12.003
- Nicolas M. Spreading of a drop of neutrally buoyant suspension. J Fluid Mech. 2005;545:271-280. doi: 10.1017/S0022112005006944
- Chen X, O’Mahony AP, Barber T. Spreading behavior of cell-laden droplets in 3D bioprinting process. J Appl Phys 2023;133(1). doi: 10.1063/5.0130063
- He P, Liu Y, Qiao R. Fluid dynamics of the droplet impact processes in cell printing. Microfluid Nanofluid. 2015;18: 569-585. doi: 10.1007/s10404-014-1470-3
- Suntornnond R, Ng WL, Huang X, Ethan Yeowa CH, Yee Yeong Wai. Improving printability of hydrogel-based bio-inks for thermal inkjet bioprinting applications via saponification and heat treatment process. J Mater Chem B. 2022;10(31):5989-6000. doi: 10.1039/D2TB00442A
- Ng WL, Lee JM, Zhou M, Yeong WY. Hydrogels for 3-D bioprinting-based tissue engineering. In: Narayan R, ed. Rapid Prototyping of Biomaterials. Chapel Hill, NC: Elsevier; 2020: 183-204.
- Tibbitt MW, Anseth KS. Hydrogels as extracellular matrix mimics for 3D cell culture. Biotechnol Bioeng. 2009;103(4):655-663. doi: 10.1002/bit.22361
- Blache U, Ford EM, Ha B, et al. Engineered hydrogels for mechanobiology. Nat Rev Methods Primers. 2022;2(1):98. doi: 10.1038/s43586-022-00179-7
- Loh QL, Choong C. Three-dimensional scaffolds for tissue engineering applications: role of porosity and pore size, Tissue Eng Part B: Reviews. 2013;19(6):485-502. doi: 10.1089/ten.teb.2012.0437
- Ng WL, Goh MH, Yeong WY, Naing MW. Applying Macromolecular Crowding to 3D Bioprinting: Fabrication of 3D Hierarchical Porous Collagen-based Hydrogel Constructs. Biomater Sci. 2018;6(3):562-574. doi: 10.1039/C7BM01015J
- Kumbar SG, Nukavarapu SP, James R, Nair LS, Laurencin CT. Electrospun poly(lactic acid-co-glycolic acid) scaffolds for skin tissue engineering. Biomaterials. 2008;29(30): 4100-4107. doi: 10.1016/j.biomaterials.2008.06.028
- Lien S-M, Ko L-Y, Huang T-J. Effect of pore size on ECM secretion and cell growth in gelatin scaffold for articular cartilage tissue engineering. Acta Biomater. 2009;5(2): 670-679. doi: 10.1016/j.actbio.2008.09.020
- Ben Messaoud G, Aveic S, Wachendoerfer M, et al. 3D printable gelatin methacryloyl (GelMA)‐dextran aqueous two‐phase system with tunable pores atructure and aize enables physiological behavior of embedded cells In Vitro. Small. 2023;2208089. doi: 10.1002/smll.202208089
- Duarte Campos DF, Blaeser A, Buellesbach K, et al. Bioprinting organotypic hydrogels with improved mesenchymal stem cell remodeling and mineralization properties for bone tissue engineering. Adv Healthc Mater. 2016;5(11):1336-1345. doi: 10.1002/adhm.201501033
- Cooper GM, Miller ED, DeCesare GE, et al. Inkjet-based biopatterning of bone morphogenetic protein-2 to spatially control calvarial bone formation. Tissue Eng Part A. 2010;16(5):1749-1759. doi: 10.1089/ten.tea.2009.0650
- Sun Z, Yue X, Liu L, et al. Bioprinted Notch ligand to function as stem cell niche improves muscle regeneration in dystrophic muscle. Int J Bioprint. 2023;9(3):711. doi: 10.18063/ijb.711
- Zimmermann R, Hentschel C, Schrön F, et al. High resolution bioprinting of multi-component hydrogels. Biofabrication. 2019;11(4):045008. doi: 10.1088/1758-5090/ab2aa1
- Ng WL, Wang S, Yeong WY, Naing MW. Skin bioprinting: Impending reality or fantasy? Trends Biotechnol. 2016;34 (9):689 - 699. doi: 10.1016/j.tibtech.2016.04.006
- Lee W, Debasitis JC, Lee VK, et al. Multi-layered culture of human skin fibroblasts and keratinocytes through three-dimensional freeform fabrication. Biomaterials. 2009;30(8):1587-1595. doi: 10.1016/j.biomaterials.2008.12.009
- Lee V, Singh G, Trasatti JP, et al. Design and fabrication of human skin by three-dimensional bioprinting. Tissue Eng Part C: Methods. 2013;20(6):473-484. doi: 10.1089/ten.tec.2013.0335
- Yanez M, Rincon J, Dones A, De Maria C, Gonzales R, Boland T. In vivo assessment of printed microvasculature in a bilayer skin graft to treat full-thickness wounds. Tissue Eng Part A. 2015;21(1-2):224-233. doi: 10.1089/ten.tea.2013.0561
- Ng WL, Tan ZQ, Yeong WY, Win Naing M. Proof-of-concept: 3D bioprinting of pigmented human skin constructs. Biofabrication. 2018;10(2):025005, 1-13. doi: 10.1088/1758-5090/aa9e1e
- Min D, Lee W, Bae IH, Lee TR, Croce P, Yoo S-S. Bioprinting of biomimetic skin containing melanocytes. Exp Dermatol. 2018;27(5):453-459. doi: 10.1111/exd.13376
- Nanmo A, Yan L, Asaba T, Wan L, Kageyama T, Fukuda J. Bioprinting of hair follicle germs for hair regenerative medicine. Acta Biomater. 2023;165:50-59. doi: 10.1016/j.actbio.2022.06.021
- Horváth L, Umehara Y, Jud C, Blank F, Petri-Fink A, Rothen- Rutishauser B. Engineering an in vitro air-blood barrier by 3D bioprinting. Sci Rep. 2015;5:7974, 1-8. doi: 10.1038/srep07974
- Ng WL, Ayi TC, Liu Y-C, Sing SL, Yeong WY, Tan B-H. Fabrication and characterization of 3D bioprinted triple-layered human alveolar lung models. Int J Bioprint. 2021;7(2):332. doi: 10.18063/ijb.v7i2.332
- Kang D, Park JA, Kim W, et al. All‐inkjet‐printed 3D alveolar barrier model with physiologically relevant microarchitecture. Adv Sci. 2021;8(10):2004990. doi: 10.1002/advs.202004990
- Ng WL, Yeong WY. The Future of Skin Toxicology Testing - 3D bioprinting meets microfluidics. Int J Bioprint. 2019;5(2.1):237. doi: 10.18063/ijb.v5i2.1.237
- El‐Ghalbzouri A, Gibbs S, Lamme E, Van Blitterswijk CA, Ponec M. Effect of fibroblasts on epidermal regeneration. Br J Dermatol. 2002;147(2):230-243. doi: j.1365-2133.2002.04871.x
- Hoet PHM, Brüske-Hohlfeld Irene, Salata OV Nanoparticles–known and unknown health risks. J Nanobiotechnol. 2004;2(1):12, 1-15. doi: 10.1186/1477-3155-2-12
- Habib RH, Chalker RB, Suki B, Jackson AC. Airway geometry and wall mechanical properties estimated from subglottal input impedance in humans. J Appl Physiol. 1994;77(1):441-451. doi: 10.1152/jappl.1994.77.1.441
- Crandall ED, Matthay MA. Alveolar epithelial transport: basic science to clinical medicine. Am J Respir Crit Care Med. 2001;163(4):1021-1029. doi: 10.1164/ajrccm.163.4.2006116
- Fehrenbach H. Alveolar epithelial type II cell: defender of the alveolus revisited. Respir Res. 2001;2(1):33-46. doi: 10.1186/rr36
- Hussell T, Bell TJ. Alveolar macrophages: plasticity in a tissue-specific context. Nat Rev Immunol. 2014;14(2):81-93. doi: 10.1038/nri3600
- Comhair SA, Xu W, Mavrakis L, Aldred MA, Asosingh K, Erzurum SC. Human primary lung endothelial cells in culture. Am J Respir Cell Mol Biol. 2012;46(6):723-730. doi: 10.1165/rcmb.2011-0416TE
- White ES. Lung extracellular matrix and fibroblast function. Ann Am Thorac Soc. 2015;12(Suppl 1):S30-S33. doi: 10.1513/AnnalsATS.201406-240MG
- LeCun Y, Bengio Y, Hinton G. Deep learning. Nature. 2015;521(7553):436-444. doi: 10.1038/nature14539
- Ng WL, Chan A, Ong YS, Chua CK. Deep learning for fabrication and maturation of 3D bioprinted tissues and organs. Virtual Phys Prototyp. 2020;15(3):340-358. doi: 10.1080/17452759.2020.1771741
- Shi J, Song J, Song B, Lu WF. Multi-objective optimization design through machine learning for drop-on-demand bioprinting. Engr. 2019;5(3):586-593. doi: 10.1016/j.eng.2018.12.009
- Wu D, Xu C. Predictive modeling of droplet formation processes in inkjet-based bioprinting. J Manuf Sci Eng. 2018;140(10):101007. doi: 10.1115/1.4040619
- Ogunsanya M, Isichei J, Parupelli SK, Desai SS, Cai Yi. In-situ droplet monitoring of inkjet 3D printing process using image analysis and machine learning models. Procedia Manuf. 2021;53:427-434. doi: 10.1016/j.promfg.2021.06.045
- Xu T, Binder KW, Albanna MZ, et al. Hybrid printing of mechanically and biologically improved constructs for cartilage tissue engineering applications. Biofabrication. 2012;5(1):015001, 1-10. doi: 10.1088/1758-5082/5/1/015001
- Daly AC, Kelly DJ. Biofabrication of spatially organised tissues by directing the growth of cellular spheroids within 3D printed polymeric microchambers. Biomaterials. 2019;197:194-206. doi: 10.1016/j.biomaterials.2018.12.028
- Dufour A, Gallostra XB, O’keeffe C, et al. Integrating melt electrowriting and inkjet bioprinting for engineering structurally organized articular cartilage. Biomaterials. 2022;283:121405. doi: 10.1016/j.biomaterials.2022.121405