Application of biomaterial-based three-dimensional bioprinting for organ-on-a-chip fabrication
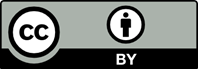
An organ-on-a-chip is a microfluidic device that simulates the microenvironment of organs, facilitating the study of human physiology and disease mechanisms. Through the integration of tissue engineering and micromachining technologies, it effectively manages the cellular microenvironment and implements tissue-specific functions and physiological responses with high fidelity. Several factors must be appropriately considered in the fabrication of an organ-on-a-chip, including the choice of biomaterials to simulate the extracellular matrix (ECM), selection of cells constituting the target organ, incorporation of humanized design to realize the primary function and structure of the organ, and the use of appropriate biofabrication methods to build a tissue-specific environment. Notably, three-dimensional (3D) bioprinting has emerged as a promising method for biofabricating organ-on-a-chip. Three-dimensional bioprinting offers versatility in adapting to various biomaterials with different physical properties, allowing precise control of 3D cell arrays and facilitating cyclic movements of fluidic flow within microfluidic platforms. These capabilities enable the precise fabrication of organ-on-a-chip that reflects tissue-specific functions and microenvironments. Additionally, 3D-bioprinted organ-on-a-chip can serve as a disease-on-a-chip platform, achieved through the implementation of pathophysiological environments and integration with devices such as bioreactors. Their significance in pharmacology research lies in their exceptional resemblance to the 3D microenvironment structure of actual organs, which are conducive for the validation of sequential mechanism of drug action. This review describes recent examples of organ-on-a-chip applications for various organs and state-of-the-art 3D bioprinting techniques employed in organ-on-a-chip fabrication. The discussion extends to the future prospects of this technology, encompassing aspects such as commercialization through mass production and its potential application in personalized medicine or drug-screening platforms. Serving as a relevant guide, this review offers insights for future research and developments in in vitro micromodel fabrication.
- Ingber DE. Human organs-on-chips for disease modelling, drug development and personalized medicine. Nat Rev Genet. 2022;23(8): 467-491. doi: 10.1038/s41576-022-00466-9
- Golding H, Khurana S, Zaitseva M. What is the predictive value of animal models for vaccine efficacy in humans? The importance of bridging studies and species-independent correlates of protection. Cold Spring Harb Perspect Biol. 2018;10(4): a028902. doi: 10.1101/cshperspect.a028902
- Seok J, Warren HS, Cuenca AG, et al. Genomic responses in mouse models poorly mimic human inflammatory diseases. Proc Natl Acad Sci U S A. 2013;110(9): 3507-3512. doi: 10.1073/pnas.1222878110
- Franco R, Cedazo-Minguez A. Successful therapies for Alzheimer’s disease: why so many in animal models and none in humans? Front Pharmacol. 2014;5: 146. doi: 10.3389/fphar.2014.00146
- Kostewicz ES, Abrahamsson B, Brewster M, et al. In vitro models for the prediction of in vivo performance of oral dosage forms. Eur J Pharm Sci. 2014;57: 342-366. doi: 10.1016/j.ejps.2013.08.024
- Low LA, Mummery C, Berridge BR, Austin CP, Tagle DA. Organs-on-chips: into the next decade. Nat Rev Drug Discov. 2021;20(5): 345-361. doi: 10.1038/s41573-020-0079-3
- Zervantonakis IK, Kothapalli CR, Chung S, Sudo R, Kamm RD. Microfluidic devices for studying heterotypic cell-cell interactions and tissue specimen cultures under controlled microenvironments. Biomicrofluidics. 2011;5(1): 013406. doi: 10.1063/1.3553237
- Huh D, Hamilton GA, Ingber DE. From 3D cell culture to organs-on-chips. Trends Cell Biol. 2011;21(12): 745-754. doi: 10.1016/j.tcb.2011.09.005
- Shamir ER, Ewald AJ. Three-dimensional organotypic culture: experimental models of mammalian biology and disease. Nat Rev Mol Cell Biol. 2014;15(10): 647-664. doi: 10.1038/nrm3873
- Fatehullah A, Tan SH, Barker N. Organoids as an in vitro model of human development and disease. Nat Cell Biol. 2016;18(3): 246-254. doi: 10.1038/ncb3312
- Chinta MS, desJardins-Park HE, Wan DC, Longaker MT. “Tissues in a dish”: a review of organoids in plastic surgery. Plast Reconstr Surg Glob Open. 2020;8(4): e2787. doi: 10.1097/GOX.0000000000002787
- Vazin T, Freed WJ. Human embryonic stem cells: derivation, culture, and differentiation: a review. Restor Neurol Neurosci. 2010;28(4): 589-603. doi: 10.3233/RNN-2010-0543
- Klicks J, von Molitor E, Ertongur-Fauth T, Rudolf R, Hafner M. In vitro skin three-dimensional models and their applications. J Cell Biotechnol. 2017;3(1): 21-39. doi: 10.3233/JCB-179004
- Leung CM, De Haan P, Ronaldson-Bouchard K, et al. A guide to the organ-on-a-chip. Nat Rev Methods Primers. 2022;2(1): 33. doi: 10.1038/s43586-022-00118-6
- Vanaei S, Parizi M, Salemizadehparizi F, Vanaei HR. An overview on materials and techniques in 3D bioprinting toward biomedical application. Eng Regen. 2021;2: 1-18. doi: 10.1016/j.engreg.2020.12.001
- Gungor-Ozkerim PS, Inci I, Zhang YS, Khademhosseini A, Dokmeci MR. Bioinks for 3D bioprinting: an overview. Biomater Sci. 2018;6(5): 915-946. doi: 10.1039/c7bm00765e
- Lee H, Cho D-W. One-step fabrication of an organ-on-a-chip with spatial heterogeneity using a 3D bioprinting technology. Lab Chip. 2016;16(14): 2618-2625. doi: 10.1039/C6LC00450D
- Reid JA, Mollica PA, Johnson GD, Ogle RC, Bruno RD, Sachs PC. Accessible bioprinting: adaptation of a low-cost 3D-printer for precise cell placement and stem cell differentiation. Biofabrication. 2016;8(2): 025017. doi: 10.1088/1758-5090/8/2/025017
- Ozbolat IT, Moncal KK, Gudapati H. Evaluation of bioprinter technologies. Addit Manuf. 2017;13: 179-200. doi: 10.1016/j.addma.2016.10.003
- Carvalho V, Gonçalves I, Lage T, et al. 3D printing techniques and their applications to organ-on-a-chip platforms: a systematic review. Sensors. 2021;21(9): 3304. doi: 10.3390/s21093304
- Ambhorkar P, Rakin RH, Wang Z, Kumar H, Kim K. Biofabrication strategies for engineering heterogeneous artificial tissues. Addit Manuf. 2020;36: 101459. doi: 10.1016/j.addma.2020.101459
- Matai I, Kaur G, Seyedsalehi A, McClinton A, Laurencin CT. Progress in 3D bioprinting technology for tissue/organ regenerative engineering. Biomaterials. 2020;226: 119536. doi: 10.1016/j.biomaterials.2019.119536
- Jung JW, Lee J-S, Cho D-W. Computer-aided multiple-head 3D printing system for printing of heterogeneous organ/ tissue constructs. Sci Rep. 2016;6(1): 21685. doi: 10.1038/srep21685
- Hockaday L, Kang K, Colangelo N, et al. Rapid 3D printing of anatomically accurate and mechanically heterogeneous aortic valve hydrogel scaffolds. Biofabrication. 2012;4(3): 035005. doi: 10.1088/1758-5082/4/3/035005
- Au AK, Lee W, Folch A. Mail-order microfluidics: evaluation of stereolithography for the production of microfluidic devices. Lab Chip. 2014;14(7): 1294-1301. doi: 10.1039/c3lc51360b
- Bhattacharjee N, Urrios A, Kang S, Folch A. The upcoming 3D-printing revolution in microfluidics. Lab Chip. 2016;16(10): 1720-1742. doi: 10.1039/c6lc00163g
- Lee H, Kim J, Choi Y, Cho D-W. Application of gelatin bioinks and cell-printing technology to enhance cell delivery capability for 3D liver fibrosis-on-a-chip development. ACS Biomater Sci Eng. 2020;6(4): 2469-2477. doi: 10.1021/acsbiomaterials.9b01735
- Skardal A, Atala A. Biomaterials for integration with 3-D bioprinting. Ann Biomed Eng. 2015;43(3): 730-746. doi: 10.1007/s10439-014-1207-1
- Unagolla JM, Jayasuriya AC. Hydrogel-based 3D bioprinting: a comprehensive review on cell-laden hydrogels, bioink formulations, and future perspectives. Appl Mater Today. 2020;18: 100479. doi: 10.1016/j.apmt.2019.100479
- Murphy SV, Skardal A, Atala A. Evaluation of hydrogels for bio‐printing applications. J Biomed Mater Res Part A. 2013;101(1): 272-284. doi: 10.1002/jbm.a.34326
- Lee KY, Mooney DJ. Hydrogels for tissue engineering. Chem Rev. 2001;101(7): 1869-1880. doi: 10.1021/cr000108x
- Khademhosseini A, Langer R. Microengineered hydrogels for tissue engineering. Biomaterials. 2007;28(34): 5087-5092. doi: 10.1016/j.biomaterials.2007.07.021
- Jang J, Yi H-G, Cho D-W. 3D printed tissue models: present and future. ACS Biomater Sci Eng. 2016;2(10): 1722-1731. doi: 10.1021/acsbiomaterials.6b00129
- Peng W, Unutmaz D, Ozbolat IT. Bioprinting towards physiologically relevant tissue models for pharmaceutics. Trends Biotechnol. 2016;34(9): 722-732. doi: 10.1016/j.tibtech.2016.05.013
- Malda J, Visser J, Melchels FP, et al. 25th anniversary article: engineering hydrogels for biofabrication. Adv Mater. 2013;25(36): 5011-5028. doi: 10.1002/adma.201302042
- Cen L, Liu W, Cui L, Zhang W, Cao Y. Collagen tissue engineering: development of novel biomaterials and applications. Pediatr Res. 2008;63(5): 492-496. doi: 10.1203/PDR.0b013e31816c5bc3
- Parenteau-Bareil R, Gauvin R, Berthod F. Collagen-based biomaterials for tissue engineering applications. Materials. 2010;3(3): 1863-1887. doi: 10.3390/ma3031863
- Persaud A, Maus A, Strait L, Zhu D. 3D bioprinting with live cells. Eng Regen. 2022;3(3): 292-309. doi: 10.1016/j.engreg.2022.07.002
- Gaudet ID, Shreiber DI. Characterization of methacrylated type-I collagen as a dynamic, photoactive hydrogel. Biointerphases. 2012;7(1): 25. doi: 10.1007/s13758-012-0025-y
- Shi H, Li Y, Xu K, Yin J. Advantages of photo-curable collagen-based cell-laden bioinks compared to methacrylated gelatin (GelMA) in digital light processing (DLP) and extrusion bioprinting. Mater Today Bio. 2023;23: 100799. doi: 10.1016/j.mtbio.2023.100799
- Khoeini R, Nosrati H, Akbarzadeh A, et al. Natural and synthetic bioinks for 3D bioprinting. Adv NanoBiomed Res. 2021;1(8): 2000097. doi: 10.1002/anbr.202000097
- Shin JH, Kang H-W. The development of gelatin-based bio-ink for use in 3D hybrid bioprinting. Int J Prec Eng Manuf. 2018;19: 767-771. doi: 10.1007/s12541-018-0092-1
- Chimene D, Kaunas R, Gaharwar AK. Hydrogel bioink reinforcement for additive manufacturing: a focused review of emerging strategies. Adv Mater. 2020;32(1): 1902026. doi: 10.1002/adma.201902026
- Nichol JW, Koshy ST, Bae H, Hwang CM, Yamanlar S, Khademhosseini A. Cell-laden microengineered gelatin methacrylate hydrogels. Biomaterials. 2010;31(21): 5536-5544. doi: 10.1016/j.biomaterials.2010.03.064
- Lee KY, Mooney DJ. Alginate: properties and biomedical applications. Prog Polym Sci. 2012;37(1): 106-126. doi: 10.1016/j.progpolymsci.2011.06.003
- Axpe E, Oyen ML. Applications of alginate-based bioinks in 3D bioprinting. Int J Mol Sci. 2016;17(12): 1976. doi: 10.3390/ijms17121976
- Jia J, Richards DJ, Pollard S, et al. Engineering alginate as bioink for bioprinting. Acta Biomater. 2014;10(10): 4323-4331. doi: 10.1016/j.actbio.2014.06.034
- Yang X, Lu Z, Wu H, Li W, Zheng L, Zhao J. Collagen-alginate as bioink for three-dimensional (3D) cell printing based cartilage tissue engineering. Mater Sci Eng C. 2018;83: 195-201. doi: 10.1016/j.msec.2017.09.002
- Othman SA, Soon CF, Ma NL, et al. Alginate-gelatin bioink for bioprinting of hela spheroids in alginate-gelatin hexagon shaped scaffolds. Polym Bull. 2021;78: 6115-6135. doi: 10.1007/s00289-020-03421-y
- Compaan AM, Christensen K, Huang Y. Inkjet bioprinting of 3D silk fibroin cellular constructs using sacrificial alginate. ACS Biomater Sci Eng. 2017;3(8): 1519-1526. doi: 10.1021/acsbiomaterials.6b00432
- Gao Q, Kim B-S, Gao G. Advanced strategies for 3D bioprinting of tissue and organ analogs using alginate hydrogel bioinks. Mar Drugs. 2021;19(12): 708. doi: 10.3390/md19120708
- Choi Y-J, Cho D-W, Lee H. Development of silk fibroin scaffolds by using indirect 3D-bioprinting technology. Micromachines. 2022;13(1): 43. doi: 10.3390/mi13010043
- Zheng Z, Wu J, Liu M, et al. 3D bioprinting of self‐standing silk‐based bioink. Adv Healthc Mater. 2018;7(6): 1701026. doi: 10.1002/adhm.201701026
- Rodriguez MJ, Brown J, Giordano J, Lin SJ, Omenetto FG, Kaplan DL. Silk based bioinks for soft tissue reconstruction using 3-dimensional (3D) printing with in vitro and in vivo assessments. Biomaterials. 2017;117: 105-115. doi: 10.1016/j.biomaterials.2016.11.046
- Pati F, Jang J, Ha D-H, et al. Printing three-dimensional tissue analogues with decellularized extracellular matrix bioink. Nat Commun. 2014;5(1): 3935. doi: 10.1038/ncomms4935
- Kim BS, Das S, Jang J, Cho D-W. Decellularized extracellular matrix-based bioinks for engineering tissue-and organ-specific microenvironments. Chem Rev. 2020;120(19): 10608-10661. doi: 10.1021/acs.chemrev.9b00808
- Choudhury D, Tun HW, Wang T, Naing MW. Organ-derived decellularized extracellular matrix: a game changer for bioink manufacturing? Trends Biotechnol. 2018;36(8):787-805. doi: 10.1016/j.tibtech.2018.03.003
- Choudhury D, Yee M, Sheng ZLJ, Amirul A, Naing MW. Decellularization systems and devices: state-of-the-art. Acta Biomater. 2020;115: 51-59. doi: 10.1016/j.actbio.2020.07.060
- Kim H, Kang B, Cui X, et al. Light‐activated decellularized extracellular matrix‐based bioinks for volumetric tissue analogs at the centimeter scale. Adv Funct Mater. 2021;31(32): 2011252. doi: 10.1002/adfm.202011252
- Asti A, Gioglio L. Natural and synthetic biodegradable polymers: different scaffolds for cell expansion and tissue formation. Int J Artif Organs. 2014;37(3): 187-205. doi: 10.530/ijao.5000307
- Griffin M, Castro N, Bas O, Saifzadeh S, Butler P, Hutmacher DW. The current versatility of polyurethane three-dimensional printing for biomedical applications. Tissue Eng Part B Rev. 2020;26(3): 272-283. doi: 10.1089/ten.TEB.2019.0224
- Woodruff MA, Hutmacher DW. The return of a forgotten polymer—polycaprolactone in the 21st century. Prog Polym Sci. 2010;35(10): 1217-1256. doi: 10.1016/j.progpolymsci.2010.04.002
- Kim BS, Jang J, Chae S, et al. Three-dimensional bioprinting of cell-laden constructs with polycaprolactone protective layers for using various thermoplastic polymers. Biofabrication. 2016;8(3): 035013. doi: 10.1088/1758-5090/8/3/035013
- Wu W, DeConinck A, Lewis JA. Omnidirectional printing of 3D microvascular networks. Adv Mater. 2011;23(24): H178-H183. doi: 10.1002/adma.201004625
- Homan KA, Kolesky DB, Skylar-Scott MA, et al. Bioprinting of 3D convoluted renal proximal tubules on perfusable chips. Sci Rep. 2016;6(1): 34845. doi: 10.1038/srep34845
- Campbell SB, Wu Q, Yazbeck J, Liu C, Okhovatian S, Radisic M. Beyond polydimethylsiloxane: alternative materials for fabrication of organ-on-a-chip devices and microphysiological systems. ACS Biomater Sci Eng. 2020;7(7): 2880-2899. doi: 10.1021/acsbiomaterials.0c00640
- Duffy DC, McDonald JC, Schueller OJ, Whitesides GM. Rapid prototyping of microfluidic systems in poly (dimethylsiloxane). Anal Chem. 1998;70(23): 4974-4984. doi: 10.1021/ac980656z
- Miranda I, Souza A, Sousa P, et al. Properties and applications of PDMS for biomedical engineering: a review. J Funct Biomater. 2021;13(1): 2. doi: 10.3390/jfb13010002
- Carneiro J, Lima R, Campos J, Miranda JM. A microparticle blood analogue suspension matching blood rheology. Soft Matter. 2021;17(14): 3963-3974. doi: 10.1039/D1SM00106J
- Wu X, Kim S-H, Ji C-H, Allen MG. A solid hydraulically amplified piezoelectric microvalve. J Micromech Microeng. 2011;21(9): 095003. doi: 10.1088/0960-1317/21/9/095003
- Rao H-X, Liu F-N, Zhang Z-Y. Preparation and oxygen/ nitrogen permeability of PDMS crosslinked membrane and PDMS/tetraethoxysilicone hybrid membrane. J Membr Sci. 2007;303(1-2): 132-139. doi: 10.1016/j.memsci.2007.07.002
- Shrestha J, Ghadiri M, Shanmugavel M, et al. A rapidly prototyped lung-on-a-chip model using 3D-printed molds. Organs Chip. 2019;1: 100001. doi: 10.1016/j.ooc.2020.100001
- Jung JP, Bhuiyan DB, Ogle BM. Solid organ fabrication: comparison of decellularization to 3D bioprinting. Biomater Res. 2016;20: 1-11. doi: 10.1186/s40824-016-0074-2
- Mandrycky C, Wang Z, Kim K, Kim D-H. 3D bioprinting for engineering complex tissues. Biotechnol Adv. 2016;34(4): 422-434. doi: 10.1016/j.biotechadv.2015.12.011
- Lee J-S, Hong JM, Jung JW, Shim J-H, Oh J-H, Cho D-W. 3D printing of composite tissue with complex shape applied to ear regeneration. Biofabrication. 2014;6(2): 024103. doi: 10.1088/1758-5082/6/2/024103
- Lee JW, Choi Y-J, Yong W-J, et al. Development of a 3D cell printed construct considering angiogenesis for liver tissue engineering. Biofabrication. 2016;8(1): 015007. doi: 10.1088/1758-5090/8/1/015007
- Lee H, Yoo JJ, Kang H-W, Cho D-W. Investigation of thermal degradation with extrusion-based dispensing modules for 3D bioprinting technology. Biofabrication. 2016;8(1): 015011. doi: 10.1088/1758-5090/8/1/015011
- Tan Y, Richards DJ, Trusk TC, et al. 3D printing facilitated scaffold-free tissue unit fabrication. Biofabrication. 2014;6(2): 024111. doi: 10.1088/1758-5082/6/2/024111
- Cheng E, Yu H, Ahmadi A, Cheung KC. Investigation of the hydrodynamic response of cells in drop on demand piezoelectric inkjet nozzles. Biofabrication. 2016;8(1):015008. doi: 10.1088/1758-5090/8/1/015008
- Cui X, Dean D, Ruggeri ZM, Boland T. Cell damage evaluation of thermal inkjet printed Chinese hamster ovary cells. Biotechnol Bioeng. 2010;106(6): 963-969. doi: 10.1002/bit.22762
- Xu T, Gregory CA, Molnar P, et al. Viability and electrophysiology of neural cell structures generated by the inkjet printing method. Biomaterials. 2006;27(19): 3580-3588. doi: 10.1016/j.biomaterials.2006.01.048
- Saunders RE, Gough JE, Derby B. Delivery of human fibroblast cells by piezoelectric drop-on-demand inkjet printing. Biomaterials. 2008;29(2): 193-203. doi: 10.1016/j.biomaterials.2007.09.032
- Tasoglu S, Demirci U. Bioprinting for stem cell research. Trends Biotechnol. 2013;31(1): 10-19. doi: 10.1016/j.tibtech.2012.10.005
- Singh M, Haverinen HM, Dhagat P, Jabbour GE. Inkjet printing—process and its applications. Adv Mater. 22(6): 673-685. doi: 10.1002/adma.200901141
- Koch L, Deiwick A, Schlie S, et al. Skin tissue generation by laser cell printing. Biotechnol Bioeng. 2012;109(7):1855-1863. doi: 10.1002/bit.24455
- Yan J, Huang Y, Chrisey DB. Laser-assisted printing of alginate long tubes and annular constructs. Biofabrication. 2012;5(1): 015002. doi: 10.1088/1758-5082/5/1/015002
- Pages E, Rémy M, Keriquel V, Correa MM, Guillotin B, Guillemot F. Creation of highly defined mesenchymal stem cell patterns in three dimensions by laser-assisted bioprinting. J Nanotechnol Eng Med. 2015;6(2): 021006. doi: 10.1115/1.4031217
- Park JH, Jang J, Lee J-S, Cho D-W. Three-dimensional printing of tissue/organ analogues containing living cells. Ann Biomed Eng. 2017;45: 180-194. doi: 10.1007/s10439-016-1611-9
- Mironov V, Visconti RP, Kasyanov V, Forgacs G, Drake CJ, Markwald RR. Organ printing: tissue spheroids as building blocks. Biomaterials. 2009;30(12): 2164-2174. doi: 10.1016/j.biomaterials.2008.12.084
- Kim BS, Ahn M, Cho W-W, Gao G, Jang J, Cho D-W. Engineering of diseased human skin equivalent using 3D cell printing for representing pathophysiological hallmarks of type 2 diabetes in vitro. Biomaterials. 2021;272: 120776. doi: 10.1016/j.biomaterials.2021.120776
- Anada T, Pan C-C, Stahl AM, et al. Vascularized bone-mimetic hydrogel constructs by 3D bioprinting to promote osteogenesis and angiogenesis. Int J Mol Sci. 2019;20(5): 1096. doi: 10.3390/ijms20051096
- Gao G, Park W, Kim BS, et al. Construction of a novel in vitro atherosclerotic model from geometry‐tunable artery equivalents engineered via in‐bath coaxial cell printing. Adv Funct Mater. 2021;31(10): 2008878. doi: 10.1002/adfm.202008878
- Lin NY, Homan KA, Robinson SS, et al. Renal reabsorption in 3D vascularized proximal tubule models. Proc Natl Acad Sci U S A. 2019;116(12): 5399-5404. doi: 10.1073/pnas.1815208116
- Singh NK, Han W, Nam SA, et al. Three-dimensional cell-printing of advanced renal tubular tissue analogue. Biomaterials. 2020;232: 119734. doi: 10.1016/j.biomaterials.2019.119734
- Menon GK. New insights into skin structure: scratching the surface. Adv Drug Deliv Rev. 2002;54: S3-S17. doi: 10.1016/s0169-409x(02)00121-7
- Van Gele M, Geusens B, Brochez L, Speeckaert R, Lambert J. Three-dimensional skin models as tools for transdermal drug delivery: challenges and limitations. Expert Opin Drug Deliv. 2011;8(6): 705-720. doi: 10.1517/17425247.2011.568937
- Lumpkin EA, Caterina MJ. Mechanisms of sensory transduction in the skin. Nature. 2007;445(7130): 858-865. doi: 10.1038/nature05662
- Huang S, Xu Y, Wu C, Sha D, Fu X. In vitro constitution and in vivo implantation of engineered skin constructs with sweat glands. Biomaterials. 2010;31(21): 5520-5525. doi: 10.1016/j.biomaterials.2010.03.060
- Bell E, Ehrlich HP, Buttle DJ, Nakatsuji T. Living tissue formed in vitro and accepted as skin-equivalent tissue of full thickness. Science. 1981;211(4486): 1052-1054. doi: 10.1126/science.7008197
- Groeber F, Holeiter M, Hampel M, Hinderer S, Schenke- Layland K. Skin tissue engineering—in vivo and in vitro applications. Adv Drug Deliv Rev. 2011;63(4-5): 352-366. doi: 10.1016/j.addr.2011.01.005
- Clement AL, Moutinho Jr TJ, Pins GD. Micropatterned dermal–epidermal regeneration matrices create functional niches that enhance epidermal morphogenesis. Acta Biomater. 2013;9(12): 9474-9484. doi: 10.1016/j.actbio.2013.08.017
- Lammers G, Roth G, Heck M, et al. Construction of a microstructured collagen membrane mimicking the papillary dermis architecture and guiding keratinocyte morphology and gene expression. Macromol Biosci. 2012;12(5): 675-691. doi: 10.1002/mabi.201100443
- Hudon V, Berthod F, Black A, Damour O, Germain L, Auger FA. A tissue‐engineered endothelialized dermis to study the modulation of angiogenic and angiostatic molecules on capillary‐like tube formation in vitro. Br J Dermatol. 2003;148(6): 1094-1104. doi: 10.1046/j.1365-2133.2003.05298.x
- Kim BS, Gao G, Kim JY, Cho D-W. 3D cell printing of perfusable vascularized human skin equivalent composed of epidermis, dermis, and hypodermis for better structural recapitulation of native skin. Adv Healthc Mater. 2019;8(7): 1801019. doi: 10.1002/adhm.201801019
- Brem H, Tomic-Canic M. Cellular and molecular basis of wound healing in diabetes. J Clin Invest. 2007;117(5): 1219-1222. doi: 10.1172/JCI32169
- Ramasamy S, Davoodi P, Vijayavenkataraman S, et al. Optimized construction of a full thickness human skin equivalent using 3D bioprinting and a PCL/collagen dermal scaffold. Bioprinting. 2021;21: e00123. doi: 10.1016/j.bprint.2020.e00123
- Ng WL, Qi JTZ, Yeong WY, Naing MW. Proof-of-concept: 3D bioprinting of pigmented human skin constructs. Biofabrication. 2018;10(2): 025005. doi: 10.1088/1758-5090/aa9e1e
- Saunders J, Elbestawi M, Fang Q. Ultrafast laser additive manufacturing: a review. J Manuf Mater Process. 2023; 7(3): 89. doi: 10.3390/jmmp7030089
- Niehues H, Bouwstra JA, El Ghalbzouri A, Brandner JM, Zeeuwen PLJM, van den Bogaard EH. 3D skin models for 3R research: the potential of 3D reconstructed skin models to study skin barrier function. Exp Dermatol. 2018;27(5): 501-511. doi: 10.1111/exd.13531
- Sun W, Liu Z, Xu J, et al. 3D skin models along with skin-on-a-chip systems: a critical review. Chin Chem Lett. 2023; 34(5): 107819. doi: 10.1016/j.cclet.2022.107819
- Folkman J, D’Amore PA. Blood vessel formation: what is its molecular basis? Cell. 1996;87(7): 1153-1155. doi: 10.1016/s0092-8674(00)81810-3
- Carmeliet P, Tessier-Lavigne M. Common mechanisms of nerve and blood vessel wiring. Nature. 2005;436(7048): 193-200. doi: 10.1038/nature03875
- L’heureux N, Pâquet S, Labbé R, Germain L, Auger FA. A completely biological tissue‐engineered human blood vessel. FASEB J. 1998;12(1): 47-56. doi: 10.1096/fasebj.12.1.47
- Jones EA, le Noble F, Eichmann A. What determines blood vessel structure? Genetic prespecification vs. hemodynamics. Physiology. 2006;21(6): 388-395. doi: 10.1152/physiol.00020.2006
- Dornhof J, Kieninger J, Muralidharan H, Maurer J, Urban GA, Weltin A. Microfluidic organ-on-chip system for multi-analyte monitoring of metabolites in 3D cell cultures. Lab Chip. 2022;22(2): 225-239. doi: 10.1039/D1LC00689D
- Skardal A, Zhang J, McCoard L, Xu X, Oottamasathien S, Prestwich GD. Photocrosslinkable hyaluronan-gelatin hydrogels for two-step bioprinting. Tissue Eng Part A. 2010;16(8): 2675-2685. doi: 10.1089/ten.TEA.2009.0798
- Gao G, Lee JH, Jang J, et al. Tissue engineered bio‐blood‐vessels constructed using a tissue‐specific bioink and 3D coaxial cell printing technique: a novel therapy for ischemic disease. Adv Funct Mater. 2017;27(33): 1700798. doi: 10.1002/adfm.201700798
- Hansson GK. Inflammation, atherosclerosis, and coronary artery disease. N Engl J Med. 2005;352(16): 1685-1695. doi: 10.1056/NEJMra043430
- Cui H, Zhu W, Huang Y, et al. In vitro and in vivo evaluation of 3D bioprinted small-diameter vasculature with smooth muscle and endothelium. Biofabrication. 2019;12(1): 015004. doi: 10.1088/1758-5090/ab402c
- Zhang Y, Kumar P, Lv S, et al. Recent advances in 3D bioprinting of vascularized tissues. Mater Des. 2021;199: 109398. doi: 10.1016/j.matdes.2020.109398
- Murugesan K, Anandapandian PA, Sharma SK, Kumar MV. Comparative evaluation of dimension and surface detail accuracy of models produced by three different rapid prototype techniques. J Indian Prosthodont Soc. 2012;12:16-20. doi: 10.1007/s13191-011-0103-8
- Mota C, Camarero-Espinosa S, Baker MB, Wieringa P, Moroni L. Bioprinting: from tissue and organ development to in vitro models. Chem Rev. 2020;120(19): 10547-10607. doi: 10.1021/acs.chemrev.9b00789
- Reint G, Rak-Raszewska A, Vainio SJ. Kidney development and perspectives for organ engineering. Cell Tissue Res. 2017;369: 171-183. doi: 10.1007/s00441-017-2616-x
- Finco DR. Kidney Function. London, United Kingdom: Elsevier; 1997. doi: 10.1016/B978-012396305-5/50018-X
- Singh NK, Kim JY, Lee JY, et al. Coaxial cell printing of a human glomerular model: an in vitro glomerular filtration barrier and its pathophysiology. Biofabrication. 2023;15(2): 024101. doi: 10.1088/1758-5090/acad2c
- King SM, Higgins JW, Nino CR, et al. 3D proximal tubule tissues recapitulate key aspects of renal physiology to enable nephrotoxicity testing. Front Physiol. 2017;8: 123. doi: 10.3389/fphys.2017.00123
- Homan KA, Gupta N, Kroll KT, et al. Flow-enhanced vascularization and maturation of kidney organoids in vitro. Nat Methods. 2019;16(3): 255-262. doi: 10.1038/s41592-019-0325-y
- Lawlor KT, Vanslambrouck JM, Higgins JW, et al. Cellular extrusion bioprinting improves kidney organoid reproducibility and conformation. Nat Mater. 2021;20(2): 260-271. doi: 10.1038/s41563-020-00853-9
- Wang D, Gust M, Ferrell N. Kidney-on-a-chip: mechanical stimulation and sensor integration. Sensors. 2022; 22(18): 6889. doi: 10.3390/s22186889
- Kim S, Takayama S. Organ-on-a-chip and the kidney. Kidney Res Clin Pract. 2015;34(3): 165-169.
doi: 10.1016/j.krcp.2015.08.001
- Cheung KL, Lafayette RA. Renal physiology of pregnancy. Adv Chronic Kidney Dis. 2013;20(3): 209-214. doi: 10.1053/j.ackd.2013.01.012
- Fransen MF, Addario G, Bouten CV, Halary F, Moroni L, Mota C. Bioprinting of kidney in vitro models: cells, biomaterials, and manufacturing techniques. Essays Biochem. 2021;65(3): 587-602. doi: 10.1042/EBC20200158
- Lasli S, Kim H J, Lee K, et al. A human liver‐on‐a‐chip platform for modeling nonalcoholic fatty liver disease. Adv Biosyst. 2019;3(8): 1900104. doi: 10.1002/adbi.201900104
- Tajiri K, Shimizu Y. Liver physiology and liver diseases in the elderly. World J Gastroenterol. 2013;19(46): 8459. doi: 10.3748/wjg.v19.i46.8459
- Bellentani S. The epidemiology of non‐alcoholic fatty liver disease. Liver Int. 2017;37: 81-84. doi: 10.1111/liv.13299
- Huang D, Gibeley SB, Xu C, et al. Engineering liver microtissues for disease modeling and regenerative medicine. Adv Funct Mater. 2020;30(44): 1909553. doi: 10.1002/adfm.201909553
- Du Y, Li N, Yang H, et al. Mimicking liver sinusoidal structures and functions using a 3D-configured microfluidic chip. Lab Chip. 2017;17(5): 782-794. doi: 10.1039/C6LC01374K
- Wisse E, De Zanger R, Charels K, Van Der Smissen P, McCuskey RS. The liver sieve: considerations concerning the structure and function of endothelial fenestrae, the sinusoidal wall and the space of Disse. Hepatology. 1985;5(4): 683-692. doi: 10.1002/hep.1840050427
- Berger DR, Ware BR, Davidson MD, Allsup SR, Khetani SR. Enhancing the functional maturity of induced pluripotent stem cell–derived human hepatocytes by controlled presentation of cell–cell interactions in vitro. Hepatology. 2015;61(4): 1370-1381. doi: 10.1002/hep.27621
- Rennert K, Steinborn S, Gröger M, et al. A microfluidically perfused three dimensional human liver model. Biomaterials. 2015;71: 119-131. doi: 10.1016/j.biomaterials.2015.08.043
- Taymour R, Kilian D, Ahlfeld T, Gelinsky M, Lode A. 3D bioprinting of hepatocytes: core–shell structured co- cultures with fibroblasts for enhanced functionality. Sci Rep. 2021;11(1): 5130. doi: 10.1038/s41598-021-84384-6
- Kang HK, Sarsenova M, Kim D-H, et al. Establishing a 3D in vitro hepatic model mimicking physiologically relevant to in vivo state. Cells. 2021;10(5): 1268. doi: 10.3390/cells10051268
- Wang T, Du Z, Zhu F, et al. Comorbidities and multi-organ injuries in the treatment of COVID-19. Lancet. 2020;395(10228): e52. doi: 10.1016/S0140-6736(20)30558-4
- Deng J, Wei W, Chen Z, et al. Engineered liver-on-a-chip platform to mimic liver functions and its biomedical applications: a review. Micromachines. 2019;10(10): 676. doi: 10.3390/mi10100676
- Jin B, Liu Y, Du S, et al. Current trends and research topics regarding liver 3D bioprinting: a bibliometric analysis research. Front Cell Dev Biol. 2022;10: 1047524. doi: 10.3389/fcell.2022.1047524
- Sahlman P, Nissinen M, Puukka P, et al. Genetic and lifestyle risk factors for advanced liver disease among men and women. J Gastroenterol Hepatol. 2020;35(2): 291-298. doi: 10.1111/jgh.14770
- Yi H-G, Lee H, Cho D-W. 3D printing of organs-on-chips. Bioengineering. 2017;4(1): 10. doi: 10.3390/bioengineering4010010
- Miri AK, Mirzaee I, Hassan S, et al. Effective bioprinting resolution in tissue model fabrication. Lab Chip. 2019;19(11): 2019-2037. doi: 10.1039/C8LC01037D
- Brodal P. The Central Nervous System: Structure and Function. New York, United States: Oxford University Press; 2004. doi: 10.1007/s00415-004-0459-3
- LináKong Y. 3D printed nervous system on a chip. Lab Chip. 2016;16(8): 1393-1400. doi: 10.1039/C5LC01270H
- Zhang WJ, Liu W, Cui L, Cao Y. Tissue engineering of blood vessel. J Cell Mol Med. 2007;11(5): 945-957. doi: 10.1111/j.1582-4934.2007.00099.x
- Abramson DI. Blood Vessels and Lymphatics. London, United Kingdom: Elsevier; 2013.
- Nabel EG. Cardiovascular disease. N Engl J Med. 2003;349(1): 60-72. doi: 10.1056/NEJMra035098
- Zhang YS, Arneri A, Bersini S, et al. Bioprinting 3D microfibrous scaffolds for engineering endothelialized myocardium and heart-on-a-chip. Biomaterials. 2016;110: 45-59. doi: 10.1016/j.biomaterials.2016.09.003
- Sharp AN, Heazell AE, Crocker IP, Mor G. Placental apoptosis in health and disease. Am J Reprod Immunol. 2010;64(3): 159-169. doi: 10.1111/j.1600-0897.2010.00837.x
- Kuo CY, Shevchuk M, Opfermann J, et al. Trophoblast– endothelium signaling involves angiogenesis and apoptosis in a dynamic bioprinted placenta model. Biotechnol Bioeng. 2019;116(1): 181-192. doi: 10.1002/bit.26850
- Kuo C-Y, Eranki A, Placone JK, et al. Development of a 3D printed, bioengineered placenta model to evaluate the role of trophoblast migration in preeclampsia. ACS Biomater Sci Eng. 2016;2(10): 1817-1826. doi: 10.1021/acsbiomaterials.6b00031
- Kim W, Kim G. Intestinal villi model with blood capillaries fabricated using collagen-based bioink and dual-cell-printing process. ACS Appl Mater Interfaces. 2018;10(48): 41185-41196. doi: 10.1021/acsami.8b17410
- Park JY, Ryu H, Lee B, et al. Development of a functional airway-on-a-chip by 3D cell printing. Biofabrication. 2018;11(1): 015002. doi: 10.1088/1758-5090/aae545
- Huh D, Matthews BD, Mammoto A, Montoya-Zavala M, Hsin HY, Ingber DE. Reconstituting organ-level lung functions on a chip. Science. 2010;328(5986): 1662-1668. doi: 10.1126/science.1188302
- O’Bryan CS, Bhattacharjee T, Niemi SR, et al. Three-dimensional printing with sacrificial materials for soft matter manufacturing. Mrs Bull. 2017;42(8): 571-577. doi: 10.1557/mrs.2017.167
- Jalili-Firoozinezhad S, Miranda CC, Cabral JM. Modeling the human body on microfluidic chips. Trends Biotechnol. 2021;39(8): 838-852. doi: 10.1016/j.tibtech.2021.01.004
- Sosa-Hernández JE, Villalba-Rodríguez AM, Romero- Castillo KD, et al. Organs-on-a-chip module: a review from the development and applications perspective. Micromachines. 2018;9(10): 536. doi: 10.3390/mi9100536
- Choi Y-J, Park H, Ha D-H, Yun H-S, Yi H-G, Lee H. 3D bioprinting of in vitro models using hydrogel-based bioinks. Polymers. 2021;13(3): 366. doi: 10.3390/polym13030366
- Yi H-G, Kim H, Kwon J, Choi Y-J, Jang J, Cho D-W. Application of 3D bioprinting in the prevention and the therapy for human diseases. Signal Transduct Target Ther. 2021;6(1): 177. doi: 10.1038/s41392-021-00566-8
- Chang R, Nam J, Sun W. Effects of dispensing pressure and nozzle diameter on cell survival from solid freeform fabrication–based direct cell writing. Tissue Eng Part A. 2008;14(1): 41-48. doi: 10.1089/ten.a.2007.0004
- Derby B. Printing and prototyping of tissues and scaffolds. Science. 2012;338(6109): 921-926. doi: 10.1126/science.1226340
- Amorim P, d’Ávila M, Anand R, Moldenaers P, Van Puyvelde P, Bloemen V. Insights on shear rheology of inks for extrusion-based 3D bioprinting. Bioprinting. 2021;22: e00129. doi: 10.1016/j.bprint.2021.e00129
- Ning L, Betancourt N, Schreyer DJ, Chen X. Characterization of cell damage and proliferative ability during and after bioprinting. ACS Biomater Sci Eng. 2018;4(11): 3906-3918. doi: 10.1021/acsbiomaterials.8b00714
- Li J, He L, Zhou C, et al. 3D printing for regenerative medicine: from bench to bedside. Mrs Bull. 2015;40(2): 145-154. doi: 10.1557/mrs.2015.5
- Bajaj P, Schweller RM, Khademhosseini A, West JL, Bashir R. 3D biofabrication strategies for tissue engineering and regenerative medicine. Annu Rev Biomed Eng. 2014;16: 247-276. doi: 10.1146/annurev-bioeng-071813-105155
- Brassard J A, Nikolaev M, Hübscher T, Hofer M, Lutolf MP. Recapitulating macro-scale tissue self-organization through organoid bioprinting. Nat Mater. 2021;20(1): 22-29. doi: 10.1038/s41563-020-00803-5
- Gao B, Yang Q, Zhao X, Jin G, Ma Y, Xu F. 4D bioprinting for biomedical applications. Trends Biotechnol. 2016;34(9): 746-756. doi: 10.1016/j.tibtech.2016.03.004
- Kirillova A, Maxson R, Stoychev G, Gomillion CT, Ionov L. 4D biofabrication using shape‐morphing hydrogels. Adv Mater. 2017;29(46): 1703443. doi: 10.1002/adma.201703443
- Lee VK, Dai G. Three-dimensional bioprinting and tissue fabrication: prospects for drug discovery and regenerative medicine. Adv Healthc Technol. 2015;1: 23. doi: 10.2147/AHCT.S69191
- Hölzl K, Lin S, Tytgat L, Van Vlierberghe S, Gu L, Ovsianikov A. Bioink properties before, during and after 3D bioprinting. Biofabrication. 2016;8(3): 032002. doi: 10.1088/1758-5090/8/3/032002
- Chen EP, Toksoy Z, Davis BA, Geibel JP. 3D bioprinting of vascularized tissues for in vitro and in vivo applications. Front Bioeng Biotechnol. 2021;9: 664188. doi: 10.3389/fbioe.2021.664188
- Ke D, Niu C, Yang X. Evolution of 3D bioprinting-from the perspectives of bioprinting companies. Bioprinting. 2022;25: e00193. doi: 10.1016/j.bprint.2022.e00193
- Lee J, Kim KE, Bang S, Noh I, Lee C. A desktop multi-material 3D bio-printing system with open-source hardware and software. Int J Prec Eng Manuf. 2017;18: 605-612. doi: 10.1007/s12541-017-0072-x
- Fateri M, Gebhardt A. Introduction to additive manufacturing. In: Heinrich A, ed. 3D Printing of Optical Components. Cham: Springer; 2021: 1-22. doi: 10.1007/978-3-030-58960-8_1
- Wu Q, Liu J, Wang X, et al. Organ-on-a-chip: recent breakthroughs and future prospects. Biomed Eng Online. 2020;19: 1-19. doi: 10.1186/s12938-020-0752-0
- Kim JJ, Park JY, Nguyen VVT, et al. Pathophysiological reconstruction of a tissue‐specific multiple‐organ on‐a‐chip for type 2 diabetes emulation using 3D cell printing. Adv Funct Mater. 2023;33(22): 2213649. doi: 10.1002/adfm.202213649
- Craighead H. Future lab-on-a-chip technologies for interrogating individual molecules. Nature. 2006;442(7101):387-393. doi: 10.1038/nature05061
- Sakai S, Yoshii A, Sakurai S, Horii K, Nagasuna O. Silk fibroin nanofibers: a promising ink additive for extrusion three-dimensional bioprinting. Mater Today Bio. 2020;8: 100078. doi: 10.1016/j.mtbio.2020.100078
- Li X, Liu B, Pei B, et al. Inkjet bioprinting of biomaterials. Chem Rev. 2020;120(19): 10793-10833. doi: 10.1021/acs.chemrev.0c00008
- Yang H, Yang K-H, Narayan RJ, Ma S. Laser-based bioprinting for multilayer cell patterning in tissue engineering and cancer research. Essays Biochem. 2021;65(3): 409-416. doi: 10.1042/EBC20200093