Low-temperature deposition 3D printing biotin-doped PLGA/β-TCP scaffold for repair of bone defects in osteonecrosis of femoral head
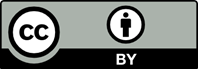
The removal of necrotic bone and implantation of bone repair materials is an effective treatment for osteonecrosis of the femoral head (ONFH)-type bone defects, but there are currently no clinically applicable bone repair materials. In this study, a biotin-doped bone repair scaffold was created using low-temperature deposition (LTD) three-dimensional (3D) printing technology, and its ability to repair bone defects in ONFH was evaluated. The scaffold was characterized in vitro, and its cytotoxicity and osteogenic capacity were assessed by co-culturing the scaffold with rat bone marrow mesenchymal stem cells. The scaffolds were implanted in an animal model of ONFH-type bone defects, and the effect of scaffolds on promoting bone repair was evaluated by means of radiology and histopathology. LTD 3D-printed biotin-doped scaffolds showed cancellous bone-like structures without inducing cytotoxicity, whereas high-biotin β-TCP scaffolds (HBPT; containing 2% biotin) promoted osteogenic differentiation more effectively. Experiments on animals revealed that the effect of HBPT on bone repair was significantly superior to that of other groups. The in vivo biocompatibility of HBPT was confirmed by blood analysis and hematoxylin and eosin staining of the main organs. In conclusion, biotin-doped scaffolds can be used to treat ONFH-type bone defects by virtue of their ability in promoting bone regeneration.
- Zhao D, Zhang F, Wang B, et al. Guidelines for clinical diagnosis and treatment of osteonecrosis of the femoral head in adults (2019 version). J Orthop Translat. 2020;21(2020):100–110. doi: 10.1016/j.jot.2019.12.004
- Cui Q, Jo WL, Koo KH, et al. ARCO consensus on the pathogenesis of non-traumatic osteonecrosis of the femoral head. J Korean Med Sci. 2021;36(10):e65. doi: 10.3346/jkms.2021.36.e65
- Chen K, Liu Y, He J, et al. Steroid-induced osteonecrosis of the femoral head reveals enhanced reactive oxygen species and hyperactive osteoclasts. Int J Biol Sci. 2020;16(11):1888–1900. doi: 10.7150/ijbs.40917
- Xue P, Chen H, Xi H, et al. Magnesium dopped calcium-fluoride/icaritin composite multi-layer coating functionalized 3D printed β-TCP scaffold inducessustained bone regeneration in a rabbit model. Mater Des. 2022;223(111156):111156. doi: 10.1016/j.matdes.2022.111156
- Yuan P, Liu X, Du B, Sun GQ, Wang X, Lin XY. Mid- to long-term results of modified avascular fibular grafting for ONFH. J Hip Preserv Surg. 2021;8(3):274–281. doi: 10.1093/jhps/hnab046
- Bavya DK, Lalzawmliana V, Saidivya M, Kumar V, Roy M, Nandi SK. Magnesium phosphate bioceramics for bone tissue engineering. Chem Rec. 2022;22(11):e202200136. doi: 10.1002/tcr.202200136
- Nabiyouni M, Brückner T, Zhou H, Gbureck U, Bhaduri SB. Magnesium-based bioceramics in orthopedic applications. Acta Biomater. 2018;66(2018):23–43. doi: 10.1016/j.actbio.2017.11.033
- Baldwin P, Li DJ, Auston DA, Mir HS, Yoon RS, Koval KJ. Autograft, allograft, and bone graft substitutes: Clinical evidence and indications for use in the setting of orthopaedic trauma surgery. J Orthop Trauma. 2019;33(4):203–213. doi: 10.1097/BOT.0000000000001420
- Zhou Q, Su X, Wu J, et al. Additive manufacturing of bioceramic implants for restoration bone engineering: Technologies, advances, and future perspectives. ACS Biomater Sci Eng. 2023;9(3):1164–1189. doi: 10.1021/acsbiomaterials.2c01164
- Chen X, Tan B, Bao Z, et al. Enhanced bone regeneration via spatiotemporal and controlled delivery of a genetically engineered BMP-2 in a composite hydrogel. Biomaterials. 2021;277(2021):121117. doi: 10.1016/j.biomaterials.2021.121117
- Hong KS, Kim EC, Bang SH, et al. Bone regeneration by bioactive hybrid membrane containing FGF2 within rat calvarium. J Biomed Mater Res A. 2010;94(4): 1187–1194. doi: 10.1002/jbm.a.32799
- León-Del-Río A. Biotin in metabolism, gene expression, and human disease. J Inherit Metab Dis. 2019;42(4):647–654. doi: 10.1002/jimd.12073
- Bain SD, Newbrey JW, Watkins BA. Biotin deficiency may alter tibiotarsal bone growth and modeling in broiler chicks. Poult Sci. 1988;67(4):590–595. doi: 10.3382/ps.0670590
- Mock DM. Biotin: From nutrition to therapeutics. J Nutr. 2017;147(8):1487–1492. doi: 10.3945/jn.116.238956
- Dai Z, Koh WP. B-vitamins and bone health--a review of the current evidence. Nutrients. 2015;7(5):3322–3346. doi: 10.3390/nu7053322
- Cao J, Yang B, Yarmolenka MA, et al. Osteogenic potential evaluation of biotin combined with magnesium-doped hydroxyapatite sustained-release film. Mater Sci Eng C Mater Biol Appl. 2022;135(2022):112679. doi: 10.1016/j.msec.2022.112679
- Cheng T, Cao J, Wu T, et al. Study on osteoinductive activity of biotin film by low-energy electron beam deposition. Biomater Adv. 2022;135(2022):212730. doi: 10.1016/j.bioadv.2022.212730
- Nobles KP, Janorkar AV, Williamson RS. Surface modifications to enhance osseointegration-Resulting material properties and biological responses. J Biomed Mater Res B Appl Biomater. 2021;109(11):1909–1923. doi: 10.1002/jbm.b.34835
- Liu W, Wang D, Huang J, et al. Low-temperature deposition manufacturing: A novel and promising rapid prototyping technology for the fabrication of tissue-engineered scaffold. Mater Sci Eng C Mater Biol Appl. 2017;70(Pt 2):976–982. doi: 10.1016/j.msec.2016.04.014
- Su X, Wang T, Guo S. Applications of 3D printed bone tissue engineering scaffolds in the stem cell field. Regen Ther. 2021;16(2021):63–72. doi: 10.1016/j.reth.2021.01.007
- Parulski C, Jennotte O, Lechanteur A, Evrard B. Challenges of fused deposition modeling 3D printing in pharmaceutical applications: Where are we now. Adv Drug Deliv Rev. 2021;175(2021):113810. doi: 10.1016/j.addr.2021.05.020
- Xu M, Li Y, Suo H, et al. Fabricating a pearl/PLGA composite scaffold by the low-temperature deposition manufacturing technique for bone tissue engineering. Biofabrication. 2010;2(2):025002. doi: 10.1088/1758-5082/2/2/025002
- Zhang T, Zhang H, Zhang L, et al. Biomimetic design and fabrication of multilayered osteochondral scaffolds by low-temperature deposition manufacturing and thermal-induced phase-separation techniques. Biofabrication. 2017;9(2):025021. doi: 10.1088/1758-5090/aa7078
- Gao X, Wang H, Luan S, Zhou G. Low-temperature printed hierarchically porous induced-biomineralization polyaryletherketone scaffold for bone tissue engineering. Adv Healthc Mater. 2022;11(18): e2200977. doi: 10.1002/adhm.202200977
- Lian M, Sun B, Han Y, et al. A low-temperature-printed hierarchical porous sponge-like scaffold that promotes cell-material interaction and modulates paracrine activity of MSCs for vascularized bone regeneration. Biomaterials. 2021;274 (2021):120841. doi: 10.1016/j.biomaterials.2021.120841
- Liu Z, Feng X, Wang H, et al. Carbon nanotubes as VEGF carriers to improve the early vascularization of porcine small intestinal submucosa in abdominal wall defect repair. Int J Nanomedicine. 2014;9(2014):1275–1286. doi: 10.2147/IJN.S58626
- Ma J, Sun Y, Zhou H, et al. Animal models of femur head necrosis for tissue engineering and biomaterials research. Tissue Eng Part C Methods. 2022;28(5):214–227. doi: 10.1089/ten.TEC.2022.0043
- Wang H, Zhang Y, Ren C, et al. Biomechanical properties and clinical significance of cancellous bone in proximal femur: A review. Injury. 2023;54(6):1432–1438. doi: 10.1016/j.injury.2023.03.010
- Kang D, Lee YB, Yang GH, et al. FeS(2)-incorporated 3D PCL scaffold improves new bone formation and neovascularization in a rat calvarial defect model. Int J Bioprint. 2023;9(1): 636. doi: 10.18063/ijb.v9i1.636
- Guo L, Liang Z, Yang L, et al. The role of natural polymers in bone tissue engineering. J Control Release. 2021;338(2021):571–582. doi: 10.1016/j.jconrel.2021.08.055
- Yoon BH, Mont MA, Koo KH, et al. The 2019 Revised Version of Association Research Circulation Osseous Staging System of Osteonecrosis of the Femoral Head. J Arthroplasty. 2020;35(4):933–940. doi: 10.1016/j.arth.2019.11.029
- Liu LH, Zhang QY, Sun W, Li ZR, Gao FQ. Corticosteroid-induced osteonecrosis of the femoral head: Detection, diagnosis, and treatment in earlier stages. Chin Med J (Engl). 2017;130(21):2601–2607. doi: 10.4103/0366-6999.217094