Successful endothelial monolayer formation on melt electrowritten scaffolds under dynamic conditions to mimic tunica intima
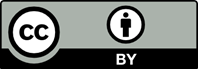
The lack of transplantable tissues and organs as well as the limitations of synthetic implants highlight the need for tissue-engineered constructs to obtain safe, long-lasting, and limitless tissue replacements. Scaffolds for cardiovascular applications, such as for a tissue-engineered vascular graft (TEVG), are thus highly required. For TEVGs, tubular scaffolds should support the formation of confluent endothelial layers in particular under dynamic conditions to prevent thrombosis and maintain hemostasis. For that purpose, a porous and highly diffusible scaffold structure is necessary to allow optimal cell adhesion as well as oxygen and nutrient exchange with the surrounding tissue. Here, we present a three-dimensional-printed scaffold made by a combination of fused deposition modeling (FDM) and melt electrowriting (MEW) out of polycaprolactone that enables monolayer formation and alignment of endothelial cells in the direction of medium flow under a shear stress of up to 10 dyn cm-2. Pore size and coating with human fibrin were optimized to enable confluent endothelial layers on the printed scaffold structures. Cell orientation and shape analysis showed a characteristic alignment and elongation of the tested endothelial cells with the direction of flow after dynamic cultivation. In contrast, melt electrospun scaffolds based on the same CAD design under comparable printing and cultivation conditions were not sufficient to form gapless cell layers. Thus, the new scaffold fabricated by MEW/FDM approach appears most suitable for TEVGs as a template for the innermost vascular wall layer, the tunica intima.
- GBD 2015 Mortality and Causes of Death Collaborators. Global, regional, and national life expectancy, all-cause mortality, and cause-specific mortality for 249 causes of death, 1980-2015: A systematic analysis for the Global Burden of Disease Study 2015. Lancet. 2016;388(1):1459-1544. doi: 10.1016/S0140-6736(16)31012-1
- Cai Q, Liao W, Xue F, et al. Selection of different endothelialization modes and different seed cells for tissue-engineered vascular graft. Bioact Mater. 2021;6(8): 2557-2568. doi: 10.1016/j.bioactmat.2020.12.021
- Eltom A, Zhong G, Muhammad A. Scaffold techniques and designs in tissue engineering functions and purposes: A review. Adv Mater Sci Eng. 2019;2019(2019):1-13. doi: 10.1155/2019/3429527
- Wang C, Xu Y, Xia J, et al. 2021;Multi-scale hierarchical scaffolds with aligned micro-fibers for promoting cell alignment. Biomed Mater. 16(4):045047. doi: 10.1088/1748-605X/ac0a90
- Liu RH, Ong CS, Fukunishi T, Ong K, Hibino N. Review of vascular graft studies in large animal models. Tissue Eng Part B Rev. 2018;24(2):133-143. doi: 10.1089/ten.teb.2017.0350
- Ainsworth MJ, Chirico N, Ruijter M de, et al. Convergence of melt electrowriting and extrusion-based bioprinting for vascular patterning of a myocardial construct. Biofabrication. 2023;15(3):035025. doi: 10.1088/1758-5090/ace07f
- Galarraga JH, Locke RC, Witherel CE, et al. Fabrication of MSC-laden composites of hyaluronic acid hydrogels reinforced with MEW scaffolds for cartilage repair. Biofabrication. 2021;14(1):014106. doi: 10.1088/1758-5090/ac3acb
- Loewner S, Heene S, Baroth T, et al. Recent advances in melt electro writing for tissue engineering for 3D printing of microporous scaffolds for tissue engineering. Front Bioeng Biotechnol. 2022;10(10):896719. doi: 10.3389/fbioe.2022.896719
- Song H-HG, Rumma RT, Ozaki CK, Edelman ER, Chen CS. Vascular tissue engineering: Progress, challenges, and clinical promise. Cell Stem Cell. 2018;22(3):340-354. doi: 10.1016/j.stem.2018.02.009
- Radke D, Jia W, Sharma D, et al. Tissue engineering at the blood-contacting surface: A review of challenges and strategies in vascular graft development. Adv Healthc Mater. 2018;7(15):e1701461. doi: 10.1002/adhm.201701461
- Yau JW, Teoh H, Verma S. Endothelial cell control of thrombosis. BMC Cardiovasc Disord. 2015;15(15):130. doi: 10.1186/s12872-015-0124-z
- Skovrind I, Harvald EB, Juul Belling H, Jørgensen CD, Lindholt JS, Andersen DC. Concise review: Patency of small-diameter tissue-engineered vascular grafts: A meta-analysis of preclinical trials. Stem Cells Transl Med. 2019;8(7): 671-680. doi: 10.1002/sctm.18-0287
- Davies PF, Civelek M, Fang Y, Fleming I. The atherosusceptible endothelium: endothelial phenotypes in complex haemodynamic shear stress regions in vivo. Cardiovasc Res. 2013;99(2):315-327. doi: 10.1093/cvr/cvt101
- Turpaev KT. Transcription factor KLF2 and its role in the regulation of inflammatory processes. Biochemistry (Mosc). 2020;85(1):54-67. doi: 10.1134/S0006297920010058
- Li H, Zhou W-Y, Xia Y-Y, Zhang J-X. Endothelial mechanosensors for atheroprone and atheroprotective shear stress signals. J Inflamm Res. 2022;15(15):1771-1783. doi: 10.2147/JIR.S355158
- Mosesson MW. Fibrinogen and fibrin structure and functions. J Thromb Haemost. 2005;3(8):1894-1904. doi: 10.1111/j.1538-7836.2005.01365.x
- Roux E, Bougaran P, Dufourcq P, Couffinhal T. Fluid shear stress sensing by the endothelial layer. Front Physiol. 2020;11(11):861. doi: 10.3389/fphys.2020.00861
- Johnston A, Callanan A. Recent methods for modifying mechanical properties of tissue-engineered scaffolds for clinical applications. Biomimetics (Basel). 2023;8(2):205. doi: 10.3390/biomimetics8020205
- Kraus X, van de Flierdt E, Renzelmann J, et al. Peripheral blood derived endothelial colony forming cells as suitable cell source for pre-endothelialization of arterial vascular grafts under dynamic flow conditions. Microvasc Res. 2022;143(143):104402. doi: 10.1016/j.mvr.2022.104402
- Kraus X, Pflaum M, Thoms S, et al. A pre-conditioning protocol of peripheral blood derived endothelial colony forming cells for endothelialization of tissue engineered constructs. Microvasc Res. 2021;134(134):104107. doi: 10.1016/j.mvr.2020.104107
- Brown MA, Wallace CS, Angelos M, Truskey GA. Characterization of umbilical cord blood-derived late outgrowth endothelial progenitor cells exposed to laminar shear stress. Tissue Eng Part A. 2009;15(11):3575-3587. doi: 10.1089/ten.tea.2008.0444
- Chu H-R, Sun Y-C, Gao Y, et al. Function of Krüppel‑like factor 2 in the shear stress‑induced cell differentiation of endothelial progenitor cells to endothelial cells. Mol Med Rep. 2019;19(3):1739-1746. doi: 10.3892/mmr.2019.9819
- Kim J, Bakirci E, O’Neill KL, Hrynevich A, Dalton PD. Fiber Bridging during melt electrowriting of poly(ε‐caprolactone) and the influence of fiber diameter and wall height. Macromol Mater Eng. 2021;306(3):2000685. doi: 10.1002/mame.202000685
- Whited BM, Rylander MN. The influence of electrospun scaffold topography on endothelial cell morphology, alignment, and adhesion in response to fluid flow. Biotechnol Bioeng. 2014;111(1):184-195. doi: 10.1002/bit.24995
- Hu Q, Su C, Zeng Z, et al. Fabrication of multilayer tubular scaffolds with aligned nanofibers to guide the growth of endothelial cells. J Biomater Appl. 2020;35(4-5):553-566. doi: 10.1177/0885328220935090
- Li X, Wang X, Yao D, et al. Effects of aligned and random fibers with different diameter on cell behaviors. Colloids Surf B Biointerfaces. 2018;171(171):461-467. doi: 10.1016/j.colsurfb.2018.07.045
- Hochleitner G, Jüngst T, Brown TD, et al. Additive manufacturing of scaffolds with sub-micron filaments via melt electrospinning writing. Biofabrication. 2015;7(3):35002. doi: 10.1088/1758-5090/7/3/035002
- Mani MP, Sadia M, Jaganathan SK, et al. A review on 3D printing in tissue engineering applications. J Polym Eng. 2022;42(3):243-265. doi: 10.1515/polyeng-2021-0059
- Fang Y, Wang C, Liu Z, et al. 3D printed conductive multiscale nerve guidance conduit with hierarchical fibers for peripheral nerve regeneration. Adv Sci (Weinh). 2023;10(12):e2205744. doi: 10.1002/advs.202205744
- Niu Y, Stadler FJ, Fang J, Galluzzi M. Hyaluronic acid-functionalized poly-lactic acid (PLA) microfibers regulate vascular endothelial cell proliferation and phenotypic shape expression. Colloids Surf B Biointerfaces. 2021;206(206):111970. doi: 10.1016/j.colsurfb.2021.111970
- Ahmed FE, Lalia BS, Hashaikeh R. A review on electrospinning for membrane fabrication: Challenges and applications. Desalination. 2015;356(356):15-30. doi: 10.1016/j.desal.2014.09.033
- Xu H, Li H, Ke Q, Chang J. An anisotropically and heterogeneously aligned patterned electrospun scaffold with tailored mechanical property and improved bioactivity for vascular tissue engineering. ACS Appl Mater Interfaces. 2015;7(16):8706-8718. doi: 10.1021/acsami.5b00996
- Pang JH, Farhatnia Y, Godarzi F, et al. In situ endothelialization: Bioengineering considerations to translation. Small. 2015;11(47):6248-6264. doi: 10.1002/smll.201402579
- Jia W, Li M, Kang L, Gu G. Fabrication and comprehensive characterization of biomimetic extracellular matrix electrospun scaffold for vascular tissue engineering applications. J Mater Sci. 2019;54(15):10871-10883. doi: 10.1007/s10853-019-03667-6
- Ju YM, Choi JS, Atala A, Yoo JJ, Lee SJ. Bilayered scaffold for engineering cellularized blood vessels. Biomaterials. 2010;31(15):4313-4321. doi: 10.1016/j.biomaterials.2010.02.002
- Liu Y, Zhang Y, An Z, et al. Slide-ring structure-based double-network hydrogel with enhanced stretchability and toughness for 3D-bio-printing and its potential application as artificial small-diameter blood vessels. ACS Appl Bio Mater. 2021;4(12):8597-8606. doi: 10.1021/acsabm.1c01052
- Baroth T, Loewner S, Heymann H, Cholewa F, Blume H, Blume C. An intelligent and efficient workflow for path-oriented 3D bioprinting of tubular scaffolds. 3D Print Addit Manuf. 10. 2023; doi: 10.1089/3dp.2022.0201
- Stanislawski N, Cholewa F, Heymann H, et al. Automated bioreactor system for the cultivation of autologous tissue-engineered vascular grafts. Annu Int Conf IEEE Eng Med Biol Soc. 2020;2020(2020):2257-2261. doi: 10.1109/EMBC44109.2020.9175340
- Bartnikowski M, Dargaville TR, Ivanovski S, Hutmacher DW. Degradation mechanisms of polycaprolactone in the context of chemistry, geometry and environment. Prog Polym Sci. 2019;96(96):1-20. doi: 10.1016/j.progpolymsci.2019.05.004
- Peña J, Corrales T, Izquierdo-Barba I, et al. Alkaline-treated poly(epsilon-caprolactone) films: Degradation in the presence or absence of fibroblasts. J Biomed Mater Res A. 2006;76(4):788-797. doi: 10.1002/jbm.a.30547
- Sung H-J, Meredith C, Johnson C, Galis ZS. The effect of scaffold degradation rate on three-dimensional cell growth and angiogenesis. Biomaterials. 2004;25(26):5735-5742. doi: 10.1016/j.biomaterials.2004.01.066
- Kamenskiy AV, Pipinos II, MacTaggart JN, Jaffar Kazmi SA, Dzenis YA. Comparative analysis of the biaxial mechanical behavior of carotid wall tissue and biological and synthetic materials used for carotid patch angioplasty. J Biomech Eng. 2011;133(11):111008. doi: 10.1115/1.4005434
- Monson KL, Goldsmith W, Barbaro NM, Manley GT. Axial mechanical properties of fresh human cerebral blood vessels. J Biomech Eng. 2003;125(2):288-294. doi: 10.1115/1.1554412
- Sánchez-Molina D, García-Vilana S, Llumà J, et al. Mechanical behavior of blood vessels: Elastic and viscoelastic contributions. Biology (Basel). 2021;10(9):831. doi: 10.3390/biology10090831
- Camasão DB, Mantovani D. The mechanical characterization of blood vessels and their substitutes in the continuous quest for physiological-relevant performances. A critical review. Mater Today Bio. 2021;10(10):100106. doi: 10.1016/j.mtbio.2021.100106