Development of P(3HB-co-3HHx) nanohydroxyapatite (nHA) composites for scaffolds manufacturing by means of fused deposition modeling
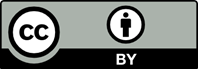
This work reports on the development of nanocomposites based on poly(3- hydroxybutyrate-co-3-hydroxyhexanoate) [P(3HB-co-3HHx)] and nanohydroxyapatite (nHA) for the development of scaffolds by means of a two-stage extrusion process followed by a 3D printing process. Tensile test samples were produced for the characterization of the materials. Each processing thermal cycle promoted a slight thermal degradation, identified by means of differential scanning calorimetry (DSC) and thermogravimetric analysis (TGA). Also, a viscosity reduction was observed in the rheological measurements. The 3D-printed tensile test samples exhibited increasing stiffness at increasing nHA content (with elastic modulus values close to 1000 MPa), while tensile strength and strain at break were reduced. Nonetheless, the deposition direction oriented with the tensile direction (raster angle of 0°C) exhibited the highest tensile strength (18 MPa) but lower elongation at break than the 45°/−45°C deposition, which resulted in the highest strain (up to 17%). Regarding the scaffolds, they were degraded in phosphate-buffered saline at 37°C for 8 weeks. This degradation was identified by a reduction of their weight (between 1.5% and 3.0%) and reduced mechanical behavior measured by means of a compression test. Scaffolds showed a decrease of the compression strength (from values close to 13 MPa to 9 MPa).
- Ngo TD, Kashani A, Imbalzano G, Nguyen KTQ, Hui D. Additive manufacturing (3D printing): A review of materials, methods, applications and challenges. Compos B Eng. 2018;143(1): 172-196. doi: 10.1016/j.compositesb.2018.02.012
- Mohan N, Senthil P, Vinodh S, Jayanth N. A review on composite materials and process parameters optimisation for the fused deposition modelling process. Virtual Phys Prototyp. 2017;12(1):47-59. doi: 10.1080/17452759.2016.1274490
- Martín-Montal J, Pernas-Sánchez J, Varas D. Experimental characterization framework for SLA additive manufacturing materials. Polymers. 2021;13(7):1-16. doi: 10.3390/polym13071147
- Lima AL, Pires FQ, Hilgert LA, Sá-Barreto LL. Oscillatory shear rheology as an in-process control tool for 3D printing medicines production by fused deposition modeling. J Manuf Process. 2022;76(1):850-862. doi: 10.1016/j.jmapro.2022.03.001
- Tarrés Q, Melbø JK, Delgado-Aguilar M, Espinach FX, Mutjé P, Chinga-Carrasco G. Bio-polyethylene reinforced with thermomechanical pulp fibers: Mechanical and micromechanical characterization and its application in 3D-printing by fused deposition modelling. Compos B Eng. 2018;153(1):70-77. doi: 10.1016/j.compositesb.2018.07.009
- Domínguez-Robles J, Utomo E, Cornelius VA, et al. TPU-based antiplatelet cardiovascular prostheses prepared using fused deposition modelling. Mater Design. 2022;220(1):1-18. doi: 10.1016/j.matdes.2022.110837
- Prasad LK, Smyth H. 3D printing technologies for drug delivery: A review. Drug Dev Ind Pharm. 2016;42(7):1019-1031. doi: 10.3109/03639045.2015.1120743
- Roopavath UK, Kalaskar DM. Introduction to 3D printing in medicine, In: 3D Printing in Medicine. 2017;Elsevier Inc., 1-20.
- Yan Q, Dong H, Su J, et al. A review of 3D printing technology for medical applications. Engineering. 2018;4(5): 729-742. doi: 10.1016/j.eng.2018.07.021
- Stansbury JW, Idacavage MJ. 3D printing with polymers: Challenges among expanding options and opportunities. Dent Mater. 2016;32(1):54-64. doi: 10.1016/j.dental.2015.09.018
- Chen X, Gao C, Jiang J, Wu Y, Zhu P, Chen G. 3D printed porous PLA/nHA composite scaffolds with enhanced osteogenesis and osteoconductivity in vivo for bone regeneration. Biomed Mater (Bristol). 2019;14(6):1-15. doi: 10.1088/1748-605X/ab388d
- Dwivedi R, Kumar S, Pandey R, et al. Polycaprolactone as biomaterial for bone scaffolds: Review of literature. J Oral Biol Craniofac Res. 2020;10(1):381-388. doi: 10.1016/j.jobcr.2019.10.003
- Dhandayuthapani B, Yoshida Y, Maekawa T, D. Kumar S. Polymeric scaffolds in tissue engineering application: A review. Int J Polym Sci. 2011;2011(1):1-19. doi: 10.1155/2011/290602
- Billström GH, Blom AW, Larsson S, Beswick AD. Application of scaffolds for bone regeneration strategies: Current trends and future directions. Injury. 2013;44(suppl.1):S28-S33. doi: 10.1016/S0020-1383(13)70007-X
- Betz MW, Yeatts AB, Richbourg WJ, et al. Macroporous hydrogels upregulate osteogenic signal expression and promote bone regeneration. Biomacromolecules. 2010;11(5):1160-1168. doi: 10.1021/bm100061z
- Webster TJ, Ahn ES. Nanostructured biomaterials for tissue engineering bone. Adv Biochem Eng/Biotechnol. 2006;103(1):275-308. doi: 10.1007/10_021
- Javaid M, Haleem A. 3D printing applications towards the required challenge of stem cells printing. Clin Epidemiol Global Health. 2020;8(3):862-867. doi: 10.1016/j.cegh.2020.02.014
- Strong D, Sirichakwal I, Manogharan GP, Wakefield T. Current state and potential of additive: Hybrid manufacturing for metal parts. Rapid Prototyp J. 2017;23(3):577-588. doi: 10.1108/RPJ-04-2016-0065
- Spierings AB, Starr TL, Wegener K. Fatigue performance of additive manufactured metallic parts. Rapid Prototyp J. 2013;19(2):88-94. doi: 10.1108/13552541311302932
- Song J, Gao H, Zhu G, Cao X, Shi X, Wang Y. The preparation and characterization of polycaprolactone/graphene oxide biocomposite nanofiber scaffolds and their application for directing cell behaviors. Carbon. 2015;95(1):1039-1050. doi: 10.1016/j.carbon.2015.09.011
- Nazeer MA, Onder OC, Sevgili I, Yilgor E, Kavakli IH, Yilgor Iskender. 3D printed poly(lactic acid) scaffolds modified with chitosan and hydroxyapatite for bone repair applications. Mater Today Commun. 2020;25(1):1-9.
doi: 10.1016/j.mtcomm.2020.101515
- Kattimani VS, Kondaka S, Lingamaneni KP. Hydroxyapatite: Past, present, and future in bone regeneration. Bone Tissue Regen Insights. 2016;7(1):BTRI.S36138. doi: 10.4137/btri.S36138
- Kim CG, Han KS, Lee S, Kim MC, Kim SY, Nah J. Fabrication of biocompatible polycaprolactone–hydroxyapatite composite filaments for the FDM 3D printing of bone scaffolds. Appl Sci (Switzerland). 2021;11(14):1-9. doi: 10.3390/app11146351
- Fierz FC, Beckmann F, Huser M, et al. The morphology of anisotropic 3D-printed hydroxyapatite scaffolds. Biomaterials. 2008;29(28):3799-3806. doi: 10.1016/j.biomaterials.2008.06.012
- Esposito Corcione C, Gervaso F, Scalera F, et al. Highly loaded hydroxyapatite microsphere/PLA porous scaffolds obtained by fused deposition modelling. Ceram Int. 2019;45(2, Part B):2803-2810. doi: https://doi.org/10.1016/j.ceramint.2018.07.297
- Yang TC. Effect of extrusion temperature on the physico-mechanical properties of unidirectional wood fiber-reinforced polylactic acid composite (WFRPC) components using fused depositionmodeling. Polymers. 2018;10(9):1-11. doi: 10.3390/polym10090976
- Ang SL, Sivashankari R, Shaharuddin B, et al. Potential applications of polyhydroxyalkanoates as a biomaterial for the aging population. Polym Degrad Stab. 2020;181(1):1-18. doi: 10.1016/j.polymdegradstab.2020.109371
- Asghari F, Samiei M, Adibkia K, Akbarzadeh A, Davaran S. Biodegradable and biocompatible polymers for tissue engineering application: A review. Artif Cells Nanomed Biotechnol. 2017;45(2):185-192. doi: 10.3109/21691401.2016.1146731
- Mehrpouya M, Vahabi H, Barletta M, et al. Additive manufacturing of polyhydroxyalkanoates (PHAs) biopolymers: Materials, printing techniques, and applications. Mater Sci Eng C. 2021;127(1):1-13. doi: 10.1016/j.msec.2021.112216
- Ferrer I, Manresa A, Méndez JA, Delgado-Aguilar M, Garcia-Romeu ML. Manufacturing PLA/PCL blends by ultrasonic molding technology. Polymers. 2021;13(15):1-17. doi: 10.3390/polym13152412
- Wu CS. Characterization, functionality and application of siliceous sponge spicules additive-based manufacturing biopolymer composites. Addit Manuf. 2018;22(1):13-20. doi: 10.1016/j.addma.2018.04.034
- Rebia RA, Rozet S, Tamada Y, Tanaka T. Biodegradable PHBH/PVA blend nanofibers: Fabrication, characterization, in vitro degradation, and in vitro biocompatibility. Polym Degrad Stab. 2018;154(1):124-136. doi: 10.1016/j.polymdegradstab.2018.05.018
- Washington MA, Balmert SC, Fedorchak MV, Little SR, Watkins SC, Meyer TY. Monomer sequence in PLGA microparticles: Effects on acidic microclimates and in vivo inflammatory response. Acta Biomater. 2018;65(1):259-271. doi: 10.1016/j.actbio.2017.10.043
- Lim J, You M, Li J, Li Z. Emerging bone tissue engineering via polyhydroxyalkanoate (PHA)-based scaffolds. Mater Sci Eng C. 2017;79(1):917-929. doi: 10.1016/j.msec.2017.05.132
- dos Santos AJ, Oliveira Dalla Valentina LV, Hidalgo Schulz AA, Tomaz MA. From obtaining to degradation of PHB: Material properties. Part I. Ingeniería y ciencia. 2017;13(26):269-298.
- Ramot Y, Haim-Zada M, Domb AJ, Nyska A. Biocompatibility and safety of PLA and its copolymers. Adv Drug Deliv Rev. 2016;107(1):153-162. doi: 10.1016/j.addr.2016.03.012
- Qu XH, Wu Q, Zhang KY, Chen GQ. In vivo studies of poly(3-hydroxybutyrate-co-3-hydroxyhexanoate) based polymers: Biodegradation and tissue reactions. Biomaterials. 2006;27(19):3540-3548. doi: 10.1016/j.biomaterials.2006.02.015
- Arza CR, Jannasch P, Johansson P, Per Magnusson, Werker A, Maurer FHJ. Effect of additives on the melt rheology and thermal degradation of poly[(R)-3-hydroxybutyric acid]. J Appl Polym Sci. 2015;132(15):1-6. doi: 10.1002/app.41836
- Kovalcik A, Sangroniz L, Kalina M, et al. Properties of scaffolds prepared by fused deposition modeling of poly(hydroxyalkanoates). Int J Biol Macromol. 2020;161(1):364-376. doi: 10.1016/j.ijbiomac.2020.06.022
- Festas AJ, Ramos A, Davim JP. Medical devices biomaterials: A review. Proc Inst Mech Eng L J Mater Design Appl. 2020;234(1):218-228. doi: 10.1177/1464420719882458
- Park SA, Lee SH, Kim WD. Fabrication of porous polycaprolactone/hydroxyapatite (PCL/HA) blend scaffolds using a 3D plotting system for bone tissue engineering. Bioprocess Biosyst Eng. 2011;34(4):505-513. doi: 10.1007/s00449-010-0499-2
- Prakash C, Singh G, Singh S, et al. Mechanical reliability and in vitro bioactivity of 3D-printed porous polylactic acid-hydroxyapatite scaffold. J Mater Eng Perform. 2021;30(7):4946-4956. doi: 10.1007/s11665-021-05566-x
- Ho-Shui-Ling A, Bolander J, Rustom LE, Amy Wagoner Johnson 4, Luyten FP, Picart C. Bone regeneration strategies: Engineered scaffolds, bioactive molecules and stem cells current stage and future perspectives. Biomaterials. 2018;180(1):143-162. doi: 10.1016/j.biomaterials.2018.07.017
- Garot C, Bettega G, Picart C. Additive manufacturing of material scaffolds for bone regeneration: Toward application in the clinics. Adv Funct Mater. 2021;31(5):2006967. doi: https://doi.org/10.1002/adfm.202006967
- Eltom A, Zhong G, Muhammad A. Scaffold techniques and designs in tissue engineering functions and purposes: A review. Adv Mater Sci Eng. 2019;(1):1-13. doi: 10.1155/2019/3429527
- Choi WJ, Hwang KS, Kwon HJ, et al. Rapid development of dual porous poly(lactic acid) foam using fused deposition modeling (FDM) 3D printing for medical scaffold application. Mater Sci Eng C. 2020;110(1) 1-9. doi: 10.1016/j.msec.2020.110693
- Backes EH, Fernandes EM, Diogo GS, et al. Engineering 3D printed bioactive composite scaffolds based on the combination of aliphatic polyester and calcium phosphates for bone tissue regeneration. Mater Sci Eng C. 2021;122(111928):1-13. doi: 10.1016/j.msec.2021.111928
- Ribeiro JFM, Oliveira SM, Alves JL, et al. Structural monitoring and modeling of the mechanical deformation of three-dimensional printed poly(ε-caprolactone) scaffolds. Biofabrication. 2017;9(2):025015. doi: 10.1088/1758-5090/aa698e
- Ye X, Zhang Y, Liu T, et al. Beta-tricalcium phosphate enhanced mechanical and biological properties of 3D-printed polyhydroxyalkanoates scaffold for bone tissue engineering. Int J Biol Macromol. 2022;209(1):1553-1561. doi: 10.1016/j.ijbiomac.2022.04.056
- Sa’ude N, Kamarudin K, Ibrahim M, Irwan Ibrahim MH. Melt Flow Index of Recycle ABS for Fused Deposition Modeling (FDM) Filament. Trans Tech Publ. 2015.
- Aumnate C, Pongwisuthiruchte A, Pattananuwat P, Potiyaraj P. Fabrication of ABS/graphene oxide composite filament for fused filament fabrication (FFF) 3D printing. Adv Mater Sci Eng. 2018;(1):1-9. doi: 10.1155/2018/2830437
- Ang KC, Leong KF, Chua CK, Margam C. Investigation of the mechanical properties and porosity relationships in fused deposition modelling‐fabricated porous structures. Rapid Prototyp J. 2006;1(1):1-6.
- Ivorra-Martinez J, Peydro MÁ, Gomez-Caturla J, Sanchez- Nacher L, Boronat T, Balart R. The effects of processing parameters on mechanical properties of 3D-printed polyhydroxyalkanoates parts. Virtual Phys Prototyp. 2023;18(1):e2164734. doi: 10.1080/17452759.2022.2164734
- Nugroho A, Ardiansyah R, Rusita L, Larasati IL. Effect of Layer Thickness on Flexural Properties of PLA (PolyLactic Acid) by 3D Printing. IOP Publishing. 2018.
- Mahmood H, Pegoretti A, Brusa RS, Ceccato R. Molecular transport through 3-hydroxybutyrate co- 3-hydroxyhexanoate biopolymer films with dispersed graphene oxide nanoparticles: Gas barrier, structural and mechanical properties. Polym Test.2020;81(1):1-9. doi: 10.1016/j.polymertesting.2019.106181
- Loh QL, Choong C. Three-dimensional scaffolds for tissue engineering applications: Role of porosity and pore size. Tissue Eng B Rev. 2013;19(6):485-502. doi: 10.1089/ten.teb.2012.0437
- Monshi M, Esmaeili S, Kolooshani A, Moghadas BK, Saber- Samandari S, Khandan A. A novel three-dimensional printing of electroconductive scaffolds for bone cancer therapy application. Nanomed J. 2020;7(2):138-148.
- Ivorra-Martinez J, Quiles-Carrillo L, Boronat T, Torres-Giner S, A José. Covas. Assessment of the mechanical and thermal properties of injection-molded poly(3-hydroxybutyrate-co- 3-hydroxyhexanoate)/hydroxyapatite nanoparticles parts for use in bone tissue engineering. Polymers. 2020;12(6) 1-21. doi: 10.3390/polym12061389
- Bordes P, Pollet E, Bourbigot S, Avérous L. Structure and properties of PHA/clay nano-biocomposites prepared by melt intercalation. Macromol Chem Phys. 2008;209(14):1473-1484. doi: 10.1002/macp.200800022
- Chacón JM, Caminero MA, García-Plaza E, Núñez PJ. Additive manufacturing of PLA structures using fused deposition modelling: Effect of process parameters on mechanical properties and their optimal selection. Mater Design. 2017;124(1):143-157. doi: 10.1016/j.matdes.2017.03.065
- Kiendl J, Gao C. Controlling toughness and strength of FDM 3D-printed PLA components through the raster layup. Compos B Eng. 2020;180(1):1-6. doi: 10.1016/j.compositesb.2019.107562
- Zhou M, Zhou X, Si L, Chen P. Modeling of bonding strength for fused filament fabrication considering bonding interface evolution and molecular diffusion. J Manuf Process. 2021;68(1):1485-1494. doi: 10.1016/j.jmapro.2021.06.064
- Santo J, Penumakala PK, Adusumalli RB. Mechanical and electrical properties of three-dimensional printed polylactic acid–graphene–carbon nanofiber composites. Polym Compos. 2021;42(7):3231-3242. doi: 10.1002/pc.26053
- Singh S, Singh G, Prakash C, Ramakrishna S. Current status and future directions of fused filament fabrication. J Manuf Process. 2020;55(1):288-306. doi: 10.1016/j.jmapro.2020.04.049
- Ecker JV, Haider A, Burzic I, Huber A, Eder G, Hild S. Mechanical properties and water absorption behavior of PLA and PLA/wood composites prepared by 3D printing and injection moulding. Rapid Prototyp J. 2019;25(4):672- 678. doi: 10.1108/RPJ-06-2018-0149
- Cisneros-López EO, Pal AK, Rodriguez AU, et al. Recycled poly(lactic acid)–based 3D printed sustainable biocomposites: A comparative study with injection molding. Mater Today Sustain. 2020;7-8(1):1-12. doi: 10.1016/j.mtsust.2019.100027
- Lay M, Thajudin NLN, Hamid ZAA, Rusli A. Comparison of physical and mechanical properties of PLA, ABS and nylon 6 fabricated using fused deposition modeling and injection molding. Compos B Eng. 2019;176(1):107341. doi: 10.1016/j.compositesb.2019.107341
- Komal UK, Kasaudhan BK, Singh I. Comparative performance analysis of polylactic acid parts fabricated by 3D printing and injection molding. J Mater Eng Perform. 2021;30(9):6522-6528. doi: 10.1007/s11665-021-05889-9
- Xu H, Xie L, Hakkarainen M. Beyond a model of polymer processing-triggered shear: Reconciling shish-kebab formation and control of chain degradation in sheared poly(l-lactic acid). ACS Sustain Chem Eng. 2015;3(7):1443-1452. doi: 10.1021/acssuschemeng.5b00320
- Farrag Y, Barral L, Gualillo O, et al. Effect of different plasticizers on thermal, crystalline, and permeability properties of poly(3–hydroxybutyrate–co−3–hydroxyhexanoate) films. Polymers. 2022;14(17):1-14. doi: 10.3390/polym14173503
- Watai JS, Calvão PS, Rigolin TR, do Prado Bettini SH, Souza AMC. Retardation effect of nanohydroxyapatite on the hydrolytic degradation of poly (lactic acid). Polym Eng Sci. 2020;60(9):2152-2162. doi: 10.1002/pen.25459
- Garcia Gonçalves LM, Rigolin TR, Frenhe BM, Bettini SHP. On the recycling of a biodegradable polymer: Multiple extrusion of poly (lactic acid). Mater Res. 2020;23(5):1-7. doi: 10.1590/1980-5373-MR-2020-0274
- Chaitanya S, Singh I, Song JI. Recyclability analysis of PLA/ sisal fiber biocomposites. Compos B Eng. 2019;173(1):1-9. doi: 10.1016/j.compositesb.2019.05.106
- Touati N, Kaci M, Bruzaud S, Grohens Y. The effects of reprocessing cycles on the structure and properties of isotactic polypropylene/cloisite 15A nanocomposites. Polym Degrad Stab. 2011;96(6):1064-1073. doi: 10.1016/j.polymdegradstab.2011.03.015
- Agüero A, Morcillo MC, Quiles-Carrillo L, et al. Study of the influence of the reprocessing cycles on the final properties of polylactide pieces obtained by injection molding. Polymers. 2019;11(12):1-21. doi: 10.3390/polym11121908
- Majerczak K, Wadkin-Snaith D, Magueijo V, Mulheran P, Liggat J, Johnston K. Polyhydroxybutyrate: A review of experimental and simulation studies of the effect of fillers on crystallinity and mechanical properties. Polym Int. 2022;71(12):1398-1408. doi: 10.1002/pi.6402
- Trakoolwannachai V, Kheolamai P, Ummartyotin S. Characterization of hydroxyapatite from eggshell waste and polycaprolactone (PCL) composite for scaffold material. Compos B Eng. 2019;173(1):1-7. doi: 10.1016/j.compositesb.2019.106974
- Martín-Alfonso JE, Franco JM. Influence of polymer reprocessing cycles on the microstructure and rheological behavior of polypropylene/mineral oil oleogels. Polym Test. 2015;45(1):12-19. doi: 10.1016/j.polymertesting.2015.04.016
- Dhar P, Tarafder D, Kumar A, Katiyar V. Effect of cellulose nanocrystal polymorphs on mechanical, barrier and thermal properties of poly(lactic acid) based bionanocomposites. RSC Adv. 2015;5(74):60426-60440. doi: 10.1039/c5ra06840a
- Wang S, Chen W, Xiang H, Yang J, Zhou Z, Zhu M. Modification and potential application of short-chain-length polyhydroxyalkanoate (SCL-PHA). Polymers. 2016;8(8):1-28. doi: 10.3390/polym8080273
- Thomas S, Sarathchandran C, Chandran N. Rheology of Polymer Blends and Nanocomposites: Theory, Modelling and Applications. Elsevier. 2019.
- Arrigo R, Bartoli M, Malucelli G. Poly(lactic acid)-biochar biocomposites: Effect of processing and filler content on rheological, thermal, and mechanical properties. Polymers. 2020;12(4) 1-13. doi: 10.3390/POLYM12040892
- Mantia FPL, Morreale M, Scaffaro R, Tulone S. Rheological and mechanical behavior of LDPE/calcium carbonate nanocomposites and microcomposites. J Appl Polym Sci. 2013;127(4):2544-2552. doi: 10.1002/app.37875
- Petrucci R, Torre L. Filled polymer composites, In: Modification of Polymer Properties. 2017;Elsevier, 23-46.
- Vaezi M, Yang S. Extrusion-based additive manufacturing of PEEK for biomedical applications. Virtual Phys Prototyp. 2015;10(3):123-135. doi: 10.1080/17452759.2015.1097053
- Jiang W, Shi J, Li W, Sun K. Morphology, wettability, and mechanical properties of polycaprolactone/hydroxyapatite composite scaffolds with interconnected pore structures fabricated by a mini‐deposition system. Polym Eng Sci. 2012;52(11):2396-2402.
- Fukushima K, Tabuani D, Dottori M, Armentano I, Kenny JM, Camino G. Effect of temperature and nanoparticle type on hydrolytic degradation of poly(lactic acid) nanocomposites. Polym Degrad Stab. 2011;96(12):2120-2129. doi: 10.1016/j.polymdegradstab.2011.09.018
- Sultana N, Khan TH. In vitro degradation of PHBV scaffolds and nHA/PHBV composite scaffolds containing hydroxyapatite nanoparticles for bone tissue engineering. J Nanomater. 2012;(1):1-13. doi: 10.1155/2012/190950
- Kim YA, Chun SY, Park SB, et al. Scaffold-supported extracellular matrices preserved by magnesium hydroxide nanoparticles for renal tissue regeneration. Biomater Sci. 2020;8(19):5427-5440. doi: 10.1039/d0bm00871k
- Jurak M, Wiącek AE, Ładniak A, Przykaza K, Szafran K. What affects the biocompatibility of polymers? Adv Colloid Interface Sci. 2021;294(1):1-15. doi: 10.1016/j.cis.2021.102451
- Wang W, Zhang B, Li M, Li J. 3D printing of PLA/n-HA composite scaffolds with customized mechanical properties and biological functions for bone tissue engineering. Compos B Eng. 2021;224(1):1-12. doi: 10.1016/j.compositesb.2021.109192
- Pachekoski WM, Dalmolin C, Agnelli JAM. The influence of the industrial processing on the degradation of poly(hidroxybutyrate)—PHB. Mater Res. 2013;16(2): 327-332. doi: 10.1590/S1516-14392012005000180
- Laput O, Vasenina I, Salvadori MC, Savkin KP. Low-temperature plasma treatment of polylactic acid and PLA/HA composite material. J Mater Sci. 2019;54(17): 11726-11738. doi: 10.1007/s10853-019-03693-4
- Gómez-Cerezo MN, Lozano D, Arcos D, Vallet-Regí M, Vaquette C. The effect of biomimetic mineralization of 3D-printed mesoporous bioglass scaffolds on physical properties and in vitro osteogenicity. Mater Sci Eng C. 2020;109(1):1-11. doi: 10.1016/j.msec.2019.110572