Selective laser melting of ferritic/martensitic oxide dispersion-strengthened steel: Processing, microstructure, and mechanical properties
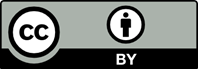
Oxide dispersion-strengthened (ODS) ferritic/martensitic steels have emerged as a promising structural material for nuclear power applications due to their high heat resistance. However, the fabrication of complex ODS steel components remains a significant challenge. This study presents the influence of the main selective laser melting process parameters and heat treatment on the densification, microstructure, and tensile properties at room and elevated temperatures of high chromium ferritic/martensitic ODS steel strengthened with 0.25 wt.% yttrium oxide (Y2O3). The optimization of process parameters and platform pre-heating enabled the production of parts with a density above 98%. The application of pre-heating allowed for higher scanning speeds to be used to achieve similar relative density and avoid cracking. Partial recrystallization after heat treatment was noted, affecting grain morphology by increasing equiaxedness and decreasing size. X-ray analysis was employed to determine the phase composition. However, the results were ambivalent and required confirmation by other methods. The addition of 0.25 wt.% Y2O3 resulted in an ultimate tensile strength value of 978 MPa for the as-built material at room temperature. At elevated temperatures, the properties are comparable to those of the base steel, indicating the necessity for further research.
- Li A, Chen Q, Wang P, et al. Microstructure and properties of oxide-reinforced FeCrAl matrix alloy manufactured by selective laser melting. Mater Today Commun. 2024;39:109226. doi: 10.1016/j.mtcomm.2024.109226
- Wang M, Sun H, Zou L, Zhang G, Li S, Zhou Z. Structural evolution of oxide dispersion strengthened austenitic powders during mechanical alloying and subsequent consolidation. Powder Technol. 2015;272:309-315. doi: 10.1016/j.powtec.2014.12.008
- Little EA, Stow DA. Void-swelling in irons and ferritic steels: II. An experimental survey of materials irradiated in a fast reactor. J Nucl Mater. 1979;87(1):25-39. doi: 10.1016/0022-3115(79)90123-5
- Shashanka R. Non-lubricated dry sliding wear behavior of spark plasma sintered nano-structured stainless steel. J Mater Environ Sci. 2019;10(8):767-777.
- Walker JC, Berggreen KM, Jones AR, Sutcliffe CJ. Fabrication of Fe-Cr-Al oxide dispersion strengthened PM2000 alloy using selective laser melting. Adv Eng Mater. 2009;11(7):541-546. doi: 10.1002/adem.200800407
- Wilms MB, Rittinghaus SK, Goßling M, Gökce B. Additive manufacturing of oxide-dispersion strengthened alloys: Materials, synthesis and manufacturing. Prog Mater Sci. 2023;133:101049. doi: 10.1016/j.pmatsci.2022.101049
- Suryanarayana C. Mechanical alloying and milling. Prog Mater Sci. 2001;46(1-2):1-184. doi: 10.1016/S0079-6425(99)00010-9
- Ou Lahcen EM, Ángel Alcázar MM, Almeida CP. New high strength ODS Eurofer steel processed by mechanical alloying. Mater Sci Eng A. 2021;817:141288. doi: 10.1016/j.msea.2021.141288
- Noh S, Choi BK, Kang SH, Kim TK. Influence of mechanical alloying atmospheres on the microstructures and mechanical properties of 15Cr ODS steels. Nucl Eng Technol. 2014;46(6):857-862. doi: 10.5516/NET.07.2013.096
- Verma L, Dabhade VV. Synthesis of Fe-15Cr-2W oxide dispersion strengthened (ODS) steel powders by mechanical alloying. Powder Technol. 2023;425:118554. doi: 10.1016/j.powtec.2023.118554
- Oksiuta Z, Ozieblo A, Perkowski K, Osuchowski M, Lewandowska M. Influence of HIP pressure on tensile properties of a 14Cr ODS ferritic steel. Fusion Eng Design. 2014;89(2):137-141. doi: 10.1016/j.fusengdes.2014.01.052
- Deng L, Luo J ru, Tu J, et al. Achieving excellent mechanical properties of ODS steel by Y2O3 addition. Mater Sci Eng A. 2023;872:145008. doi: 10.1016/j.msea.2023.145008
- Shi W, Yu L, Liu C, et al. Evolution of Y2O3 precipitates in ODS-316 L steel during reactive-inspired ball-milling and spark plasma sintering processes. Powder Technol. 2022;398:117072. doi: 10.1016/j.powtec.2021.117072
- Macía E, García-Junceda A, Serrano M, Hong SJ, Campos M. Effect of mechanical alloying on the microstructural evolution of a ferritic ODS steel with (Y-Ti-Al-Zr) addition processed by Spark Plasma Sintering (SPS). Nucl Eng Technol. 2021;53(8):2582-2590. doi: 10.1016/j.net.2021.02.002
- Zhao M, Zhang P, Xu J, et al. Optimization of microstructure and tensile properties for a 13Cr-1W ODS steel prepared by mechanical alloying and spark plasma sintering using pre-alloyed powder. Mater Character. 2024;207:113581. doi: 10.1016/j.matchar.2023.113581
- Li XP, Kang CW, Huang H, Sercombe TB. The role of a low-energy-density re-scan in fabricating crack-free Al85Ni5Y6Co2Fe2 bulk metallic glass composites via selective laser melting. Mater Des. 2014;63:407-411. doi: 10.1016/j.matdes.2014.06.022
- Vasquez E, Giroux PF, Lomello F, et al. Elaboration of oxide dispersion strengthened Fe-14Cr stainless steel by selective laser melting. J Mater Process Technol. 2019;267:403-413. doi: 10.1016/j.jmatprotec.2018.12.034
- Sagaradze VV, Kozlov KA, Kataeva NV. Oxide-dispersion strengthened radiation-resistant steels. Phys Met Metallogr. 2018;119(13):1350-1353. doi: 10.1134/S0031918X18130112
- Tanno T, Ohtsuka S, Yano Y, Kaito T, Tanaka K. Effects of manufacturing process on impact properties and microstructures of ODS steels. J Nucl Mater. 2014;455(1):480-485. doi: 10.1016/j.jnucmat.2014.07.075
- Gil E, Ordás N, García-Rosales C, Iturriza I. Microstructural characterization of ODS ferritic steels at different processing stages. Fus Eng Des. 2015;98-99:1973-1977. doi: 10.1016/j.fusengdes.2015.06.010
- Hsiung LL, Fluss MJ, Kimura A. Structure of oxide nanoparticles in Fe-16Cr MA/ODS ferritic steel. Mater Lett. 2010;64(16):1782-1785. doi: 10.1016/j.matlet.2010.05.039
- Amini R, Alijani F, Ghaffari M, Alizadeh M, Okyay AK. Formation of B19′, B2, and amorphous phases during mechano-synthesis of nanocrystalline NiTi intermetallics. Powder Technol. 2014;253:797-802. doi: 10.1016/j.powtec.2013.12.029
- Smith TM, Thompson AC, Gabb TP, Bowman CL, Kantzos CA. Efficient production of a high-performance dispersion strengthened, multi-principal element alloy. Sci Rep. 2020;10(1):9663. doi: 10.1038/s41598-020-66436-5
- Ozerskoi N, Volokitina E, Razumov N, Popovich A. Mechanical properties of ODS steel fabrication by mechanical alloying and sparking plasma sintering. AIP Conf Proc. 2024;3154(1):020029. doi: 10.1063/5.0201304
- Jia H, Zhou Z, Li S. A new strategy for additive manufacturing ODS steel using Y-containing gas atomized powder. Mater Character. 2022;187:111876. doi: 10.1016/j.matchar.2022.111876
- Cakmak O, Yeom H, Cho JW. In-situ synthesis of Yttria-based precipitates and their effects on Fe12Cr6Al in laser powder bed fusion. J Mater Res Technol. 2024;33:6714-6721. doi: 10.1016/j.jmrt.2024.11.059
- Autones L, Aubry P, Ribis J, Leguy H, Legris A, De Carlan Y. Assessment of ferritic ODS steels obtained by laser additive manufacturing. Materials (Basel). 2023;16(6):2397. doi: 10.3390/ma16062397
- Zhai W, Zhou W, Nai SML. Effect of interface wettability on additively manufactured metal matrix composites: A case study of 316L-Y2O3 oxide dispersion-strengthened steel. Metals. 2024;14(2):170. doi: 10.3390/met14020170
- Jia H, Sun H, Wang H, Wu Y, Wang H. Scanning strategy in selective laser melting (SLM): A review. Int J Adv Manuf Technol. 2021;113(9):2413-2435. doi: 10.1007/s00170-021-06810-3
- Polekhina NA, Litovchenko IY, Tyumentsev AN, Astafurova ЕG, Chernov VM, Leontyeva-Smirnova MV. The effect of tempering temperature on the features of phase transformations in the ferritic-martensitic steel EK-181. J Nucl Mater. 2014;455(1-3):496-499. doi: 10.1016/j.jnucmat.2014.08.012
- Qin SS. Influence of Preheating Temperature on Microstructure Evolution and Hardness of High‐Speed Steel AISI M50 Processed by Laser Powder Bed Fusion - Qin - 2023 - Steel Research International. Wiley Online Library. https:// onlinelibrary.wiley.com/doi/10.1002/srin.202200784 [Last accessed on 2025 Jan 29].
- Qin S, Saewe J, Kunz J, et al. Influence of preheating temperature on microstructure evolution and hardness of high‐speed steel AISI M50 processed by laser powder bed fusion. Steel Res Int. 2023;94(6):2200784. doi: 10.1002/srin.202200784
- Masuo H, Tanaka Y, Morokoshi S, et al. Effects of defects, surface roughness, and HIP on fatigue strength of Ti-6Al-4V manufactured by additive manufacturing. Proc Struct Integr. 2017;7:19-26. doi: 10.1016/j.prostr.2017.11.055
- Shi Y, Lu Z, Xu H, Xie R, Ren Y, Yang G. Microstructure characterization and mechanical properties of laser additive manufactured oxide dispersion strengthened Fe-9Cr alloy. J Alloys Compd. 2019;791:121-133. doi: 10.1016/j.jallcom.2019.03.284
- Spiridonova KV, Litovchenko IYu, Polekhina NA, et al. Structural-phase transformations of 12% chromium ferritic-martensitic steel EP-823. Izv Ferrous Metall. 2023;66(6):725-732. doi: 10.17073/0368-0797-2023-6-725-732
- Wang Z, Liu Z, Ma J, et al. Investigation on microstructure and mechanical properties of electron-beam-welded joint of reduced activation ferritic/martensitic steel fabricated by selective laser melting. Mater Sci Eng A. 2023;881:145333. doi: 10.1016/j.msea.2023.145333
- Seede R, Zhang B, Whitt A, et al. Effect of heat treatments on the microstructure and mechanical properties of an ultra-high strength martensitic steel fabricated via laser powder bed fusion additive manufacturing. Addit Manuf. 2021;47:102255. doi: 10.1016/j.addma.2021.102255
- Zaitceva M, Erutin D, Popovich A, Sufiiarov V. Effect of pre-heating during selective laser melting of chromium steel on structure and properties. Global Energy. 2024;30(3):43-51. doi: 10.18721/JEST.30303
- Ghayoor M, Mirzababaei S, Lee K, et al. Strengthening of 304L Stainless Steel by Addition of Yttrium Oxide and Grain Refinement during Selective Laser Melting. In: Solid Freeform Fabrication 2019: Proceedings of the 30th Annual International Solid Freeform Fabrication Symposium-An Additive Manufacturing Conference; 2019. p. 967-976.
- Sagaradze VV, Kochetkova TN, Kataeva NV, et al. Structure and creep of Russian reactor steels with a BCC structure. Phys Metals Metallogr. 2017;118(5):494-506. doi: 10.1134/S0031918X17050131
- Votinin SN, Balashov VD, Krylov EA, et al. Effect of Neutron Irradiation on High-Temperature Properties of Stainless Steels type Cr13 (Влияние Нейтронного Облучения на Высокотемпературные Свойства Нержавеющих Сталей Типа Х13). In: Proceedings of the Scientific and Technical Conference “Nuclear Energy: Fuel Cycles, Radiation Materials Science”, Ulyanovsk, October 5-10; 1971. p. 351-379.