Recent progress on materials for functional additive manufacturing
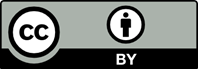
Material science in additive manufacturing (AM) has experienced remarkable advancements in the development of functional materials. This review systematically investigates the state-of-the-art research on AM of functional materials, providing a comprehensive overview of AM systems and methodologies employed for functional materials and applications. The review delves into various functional materials, including magnetic, metal powder, perovskite, piezoelectric, thermoelectric, and carbon-based materials, exploring their fabrication and applications in creating multifunctional components and devices. Furthermore, it examines the integration of these functional materials, enabling manufacturing on curved surfaces, the development of flexible components, and the enhancement of functional properties. By analyzing the latest developments in this rapidly evolving field, this review offers insights into current challenges, future directions, and potential innovations, promoting a deeper understanding of AM technology and stimulating further advancements toward the realization of advanced functional devices and systems.
- Madhavadas V, Srivastava D, Chadha U, et al. A review on metal additive manufacturing for intricately shaped aerospace components. CIRP J Manuf Sci Technol. 2022;39:18-36. doi: 10.1016/j.cirpj.2022.07.005
- Park JW, Shin YC, Kang HG, et al. In vivo analysis of post-joint-peserving surgery fracture of 3D-printed Ti-6Al-4V implant to treat bone cancer. Bio Des Manuf. 2021;4:879-888. doi: 10.1007/s42242-021-00147-2
- Kim T, Kim JG, Park S, et al. Virtual surface morphology generation of Ti-6Al-4V directed energy deposition via conditional generative adversarial network. Virtual Phys Prototyp. 2023;18(1):e2124921. doi: 10.1080/17452759.2022.2124921
- Tan LJ, Zhu W, Zhou K. Recent progress on polymer materials for additive manufacturing. Adv Funct Mater. 2020;30(43):2003062. doi: 10.1002/adfm.202003062
- Wu H, Fahy WP, Kim S, et al. Recent developments in polymers/polymer nanocomposites for additive manufacturing. Prog Mater Sci. 2020;111:100638. doi: 10.1016/j.pmatsci.2020.100638
- Li J, Lin X, Kang N, Lu J, Wang Q, Huang W. Microstructure, tensile and wear poperties of a novel graded al matrix composite pepared by direct energy deposition. J Alloy Compd. 2020;826:154077. doi: 10.1016/j.jallcom.2020.154077
- Seo E, Sung H, Jeon H, et al. Laser powder bed fusion for AI assisted digital metal components. Virtual Phys Prototyp. 2022;17(4):806-820. doi: 10.1080/17452759.2022.2068804
- Hotz H, Zimmermann M, Greco S, Kirsch B, Aurich JC. Additive manufacturing of functionally graded Ti-Al structures by laser-based direct energy deposition. J Manuf Process. 2021;68:1524-1534. doi: 10.1016/j.jmapro.2021.06.068
- Jung ID, Lee MS, Lee J, et al. Embedding sensors using selective laser melting for self-cognitive metal parts. Addit Manuf. 2020;33:101151. doi: 10.1016/j.addma.2020.101151
- Lee MS, Kim H, Koo YT, et al. Selective laser melting process for sensor embedding into SUS316L with heat dissipative inner cavity design. Met Mater Int. 2022;28:1-9. doi: 10.1007/s12540-021-01106-3
- Lee J, Choe J, Park J, Yu JH, Kim S, Sung H. Microstructural effects on the tensile and fracture behavior of selective laser melted H13 tool steel under varying conditions. Mater Charact. 2019;55:109817. doi: 10.1016/j.matchar.2019.109817
- Verma S, Yang CK, Lin CH, Jeng JY. Additive manufacturing of lattice structures for high strength mechanical interlocking of metal and resin during injection molding. Addit Manuf. 2022;49:102463. doi: 10.1016/j.addma.2021.102463
- Joyee EB, Pan Y. Additive manufacturing of multi-material soft robot for on-demand drug delivery applications. J Manuf Process. 2020;56:1178-1184. doi: 10.1016/j.jmapro.2020.03.059
- Rafiee M, Farahani RD, Therriault D. Multi‐material 3D and 4D printing: A survey. Adv Sci. 2020;7(12):1902307. doi: 10.1002/advs.201902307
- Champeau M, Heinze DA, Viana TN, de Souza ER, Chinellato AC, Titotto S. 4D printing of hydrogels: A review. Adv Funct Mater. 2020;30(31):1910606. doi: 10.1002/adfm.201910606
- Liu J, Shang Y, Shao Z, Liu X, Zhang C. Three-dimensional printing to translate simulation to architecting for three-dimensional high performance piezoelectric Energy harvester. Ind Eng Chem Res. 2021;61(1):433-440. doi: 10.1021/acs.iecr.1c04433
- Zhang D, Lim WYS, Duran SSF, Loh XJ, Suwardi A. Additive manufacturing of thermoelectrics: Emerging trends and outlook. ACS Energy Lett. 2022;7(2):720-735. doi: 10.1021/acsenergylett.1c02553
- Park JW, Seo E, Park H, et al. Hybrid solid mesh structure for electron beam melting customized implant to treat bone cancer. Int J Bioprinting. 2023;9(4):716. doi: 10.18063/ijb.716
- Culmone C, Smit G, Breedveld P. Additive manufacturing of medical instruments: A state-of-the-art review. Addit Manuf. 2019;27:461-473. doi: 10.1016/j.addma.2019.03.015
- Huang J, Chen Q, Jiang H, et al. A survey of design methods for material extrusion polymer 3D printing. Virtual Phys Prototyp. 2020;15(2):148-162. doi: 10.1080/17452759.2019.1708027
- Hossain SS, Gao B, Park S, Bae CJ. Incorporating nanoparticles in alumina ink for improved solid-loading and sinterability of extrusion-based 3D printing. ACS Appl Nano Mater. 2022;5(12):17828-17838. doi: 10.1021/acsanm.2c03792
- Kim JH, Park S, Ahn J, et al. Meniscus‐guided micro‐printing of prussian blue for smart electrochromic display. Adv Sci. 2023;10(3):2205588. doi: 10.1002/advs.202205588
- Lee S, Kim JH, Wajahat M, et al. Three-dimensional printing of silver microarchitectures using newtonian nanoparticle inks. ACS Appl Mater Interfaces. 2017;9(22):18918-18924. doi: 10.1021/acsami.7b02581
- Gu X, Shaw L, Gu K, Toney MF, Bao Z. The meniscus-guided deposition of semiconducting polymers. Nat Commun. 2018;9(1):534. doi: 10.1038/s41467-018-02833-9
- Pagac M, Hajnys J, Ma QP, et al. A review of vat photopolymerization technology: Materials, applications, challenges, and future trends of 3D printing. Polymers. 2021;13(4):598. doi: 10.3390/polym13040598
- Gross B, Lockwood SY, Spence DM. Recent advances in analytical chemistry by 3D printing. Anal Chem. 2017;89(1):57-70. doi: 10.1021/acs.analchem.6b04344
- Chiappone A, Fantino E, Roppolo I, et al. 3D printed PEG-based hybrid nanocomposites obtained by sol-gel technique. ACS Appl Mater Interfaces. 2016;8(8):5627-5633. doi: 10.1021/acsami.5b12578
- Wang W, Liu S, Liu L, Alfarhan S, Jin K, Chen X. High-speed and high-resolution 3D printing of self-healing and ion-conductive hydrogels via μCLIP. ACS Mater Lett. 2023;5(6):1727-1737. doi: 10.1021/acsmaterialslett.3c00439
- Jose RR, Rodriguez MJ, Dixon TA, Omenetto F, Kaplan DL. Evolution of bioinks and additive manufacturing technologies for 3D bioprinting. ACS Biomater Sci Eng. 2016;2(10):1662-1678. doi: 10.1021/acsbiomaterials.6b00088
- Ouyang H, Li X, Lu X, Xia H. Selective laser sintering 4D printing of dynamic cross-linked polyurethane containing diels-alder bonds. ACS Appl Polym Mater. 2022;4(5):4035-4046. doi: 10.1021/acsapm.2c00565
- Sireesha M, Lee J, Kiran ASK, Babu VJ, Kee BBT, Ramakrishna S. A review on additive manufacturing and its way into the oil and gas industry. RSC Adv. 2018;8(40):22460-22468. doi: 10.1039/C8RA03194K
- Hines L, Petersen K, Lum GZ, Sitti M. Soft actuators for small‐scale robotics. Adv Mater. 2017;29(13):1603483. doi: 10.1002/adma.201603483
- Ju Y, Hu R, Xie Y, et al. Reconfigurable magnetic soft robots with multimodal locomotion. Nano Energy. 2021;87:106169. doi: 10.1016/j.nanoen.2021.106169
- Kim Y, Zhao X. Magnetic soft materials and robots. Chem Rev. 2022;122(5):5317-5364. doi: 10.1021/acs.chemrev.1c00481
- Zhang C, Li X, Jiang L, et al. 3D pinting of functional magnetic materials: From design to applications. Adv Funct Mater. 2021;31(34):2102777. doi: 10.1002/adfm.20210277
- Zhao R, Kim Y, Chester SA, Sharma P, Zhao X. Mechanics of hard-magnetic soft materials. J Mech Phys Solids. 2019;124:244-263. doi: 10.1016/j.jmps.2018.10.008
- Lantean S, Barrera G, Pirri CF, et al. 3D printing of magnetoresponsive polymeric materials with tunable mechanical and magnetic properties by digital light processing. Adv Mater Technol. 2019;4(11):1900505. doi: 10.1002/admt.201900505
- Shasha C, Krishnan KM. Nonequilibrium dynamics of magnetic nanoparticles with applications in biomedicine. Adv Mater. 2021;33(23):1904131. doi: 10.1002/adma.201904131
- Xiao Y, Du J. Superparamagnetic nanoparticles for biomedical applications. J Mater Chem B. 2020;8(3):354-367. doi: 10.1039/C9TB01955C
- Ghazanfari MR, Kashefi M, Shams SF, Jaafari MR. Perspective of Fe3O4 nanoparticles role in biomedical applications. Biochem Res Int. 2016;2016:7840161. doi: 10.1155/2016/7840161
- Wajahat M, Kim JH, Kim JH, Jung ID, Pyo J, Seol SK. 4D printing of ultrastretchable magnetoactive soft material architectures for soft actuators. ACS Appl Mater Interfaces. 2023;15(51):59582-59591. doi: 10.1021/acsami.3c12173
- Wu S, Hamel CM, Ze Q, Yang F, Qi HJ, Zhao R. Evolutionary algorithm‐guided voxel‐encoding printing of functional hard‐magnetic soft active materials. Adv Intell Syst. 2020;2(8):2000060. doi: 10.1002/aisy.202000060
- Ren Z, Hu W, Dong X, Sitti M. Multi-functional soft-bodied jellyfish-like swimming. Nat Commun. 2019;10(1):2703. doi: 10.1038/s41467-019-10549-7
- Kim Y, Yuk H, Zhao R, Chester SA, Zhao X. Printing ferromagnetic domains for untethered fast-transforming soft materials. Nature. 2018;558(7709):274-279. doi: 10.1038/s41586-018-0185-0
- Zhang Y, Wang Q, Yi S, et al. 4D printing of magnetoactive soft materials for on-demand magnetic aactuation transformation. ACS Appl Mater Interfaces. 2021;13(3):4174-4184. doi: 10.1021/acsami.0c19280
- Kim Y, Parada GA, Liu S, Zhao X. Ferromagnetic soft continuum robots. Sci Robot. 2019;4(33):eaax7329. doi: 10.1126/scirobotics.aax7329
- Zhang Y, Pan C, Liu P, et al. Coaxially printed magnetic mechanical electrical hybrid structures with actuation and sensing functionalities. Nat Commun. 2023;14(1):4428. doi: 10.1038/s41467-023-40109-z
- Hofmann M. 3D printing gets a boost and opportunities with polymer materials. ACS Macro Lett. 2014;3(4):295-397. doi: 10.1021/mz4006556
- Xu T, Zhang J, Salehizadeh M, Onaizah O, Diller E. Millimeter-scale flexible robots with programmable three-dimensional magnetization and motions. Sci Robot. 2019;4(29):eaav4494. doi: 10.1126/scirobotics.aav4494
- Shao G, Ware HOT, Huang J, Hai R, Li L, Sun C. 3D printed magnetically-actuating micro-gripper operates in air and water. Addit Manuf. 2021;38:101834. doi: 10.1016/j.addma.2020.101834
- Siripongpreda T, Hoven VP, Narupai B, Rodthongkum N. Emerging 3D printing based on polymers and nanomaterial additives: Enhancement of properties and potential applications. Eur Polym J. 2021;184:111806. doi: 10.1016/j.eurpolymj.2022.111806
- Tan HW, An J, Chua CK, Tran T. Metallic nanoparticle inks for 3D printing of electronics. Adv Electron Mater. 2019;5(5):1800831. doi: 10.1002/aelm.201800831
- Huang Q, Zhu Y. Printing conductive nanomaterials for flexible and stretchable electronics: A review of materials, processes, and applications. Adv Mater Technol. 2019;4(5):1800546. doi: 10.1002/admt.201800546
- Tan HW, Choong YYC, Kuo CN, Low HY, Chua CK. 3D printed electronics: Processes, materials and future trends. Prog Mater Sci. 2022;127:100945. doi: 10.1016/j.pmatsci.2022.100945
- Yang C, Gu H, Lin W, et al. Silver nanowires: From scalable synthesis to recyclable foldable electronics. Adv Mater. 2011;23(27):3052-3056. doi: 10.1002/adma.201100530
- Fu D, Yang R, Wang Y, Wang R, Hua F. Silver nanowire synthesis and applications in composites: Progress and prospects. Adv Mater Technol. 2022;7(11):2200027. doi: 10.1002/admt.202200027
- Kim J, Kim WS. Stretching silver: Printed metallic nano inks in stretchable conductor applications. IEEE Nanotechnol Mag. 2014,8(4):6-13. doi: 10.1109/MNANO.2014.2355274
- Jo H, Park JS, Lim HY, Lee GY. Laser sintered silver nanoparticles on the PDMS for a wearable strain sensor capable of detecting finger motion. ACS Appl Nano Mater. 2023;6(24):22998-23011. doi: 10.1021/acsanm.3c04386
- Lee GY, Kim MS, Min SH, et al. Highly sensitive solvent-free silver nanoparticle strain sensors with tunable sensitivity created using an aerodynamically focused nanoparticle printer. ACS Appl Mater Interfaces. 2019;11(29):26421-26432. doi: 10.1021/acsami.9b00943
- Jain PK, El-Sayed IH, El-Sayed MA. Au nanoparticles target cancer. Nano Today. 2007;2(1):18-29. doi: 10.1016/S1748-0132(07)70016-6
- Boisselier E, Astruc D. Gold nanoparticles in nanomedicine: Preparations, imaging, diagnostics, therapies and toxicity. Chem Soc Rev. 2009;6:1759-1782. doi: 10.1039/B806051G
- Dykman L, Khlebtsov N. Gold nanoparticles in biomedical applications: Recent advances and perspectives. Chem Soc Rev. 2012;41:2256-2282. doi: 10.1039/C1CS15166E
- Park BK, Kim D, Jeong S, Moon J, Kim JS. Direct writing of copper conductive patterns by ink-jet printing. Thin Solid Films. 2007;515(19):7706-7711. doi: 10.1016/j.tsf.2006.11.142
- Jeong S, Woo K, Kim D, et al. Controlling the thickness of the surface oxide layer on Cu nanoparticles for the fabrication of conductive structures by ink-jet printing. Adv Funct Mater. 2008;18(5):671-830. doi: 10.1002/adfm.200700902
- Kim YJ, Ryu CH, Park SH, Kim HS. The effect of poly (N-vinylpyrrolidone) molecular weight on flash light sintering of copper nanopaste. Thin Solid Films. 2014;570:114-122. doi: 10.1016/j.tsf.2014.09.035
- Dharmadasa R, Jha M, Amos DA, Druffel T. Room temperature synthesis of a copper ink for the intense pulsed light sintering of conductive copper films. ACS Appl Mater Interfaces. 2013;5(24):12773-13484. doi: 10.1021/am404226e
- Zhan P, Jia Y, Zhai W, et al. A fibrous flexible strain sensor with Ag nanoparticles and carbon nanotubes for synergetic high sensitivity and large response range. Compos A Appl Sci Manuf. 2023;167:107431. doi: 10.1016/j.compositesa.2023.107431
- Aslanidis E, Skotadis E, Tsoukalas D. Resistive crack-based nanoparticle strain sensors with extreme sensitivity and adjustable gauge factor, made on flexible substrates. Nanoscale. 2021;13(5):3263-3274. doi: 10.1039/d0nr07002e
- Soe HM, Manaf AA, Matsuda A, Jaafar M. Development and fabrication of highly flexible, stretchable, and sensitive strain sensor for long durability based on silver nanoparticles-polydimethylsiloxane composite. J Mater Sci Mater Electron. 2020;31:11897-11910. doi: 10.1007/s10854-020-03744-6
- Hammock ML, Chortos A, Tee BCK, Tok JBH, Bao Z. 25th Anniversary article: The evolution of electronic skin (E-Skin): A brief history, design considerations, and recent progress. Adv Mater. 2013;25(42):5997-6038. doi: 10.1002/adma.201302240
- Guo SZ, Qiu K, Meng F, Park SH, McAlpine MC. 3D printed stretchable tactile sensors. Adv Mater. 2017;29(27):1701218. doi: 10.1002/adma.201701218
- Valentine AD, Busbee TA, Boley JW, et al. Hybrid 3D printing of soft electronics. Adv Mater. 2017;29(40):1703817. doi: 10.1002/adma.201703817
- Zhu Z, Guo SZ, Hirdler T, et al. 3D printed functional and biological materials on moving freeform surfaces. Adv Mater. 2018;30(23):1707495. doi: 10.1002/adma.201707495
- Zhou LY, Gao Q, Zhan JF, Xie CQ, Fu JZ, He Y. Three-dimensional printed wearable sensors with liquid metals for detecting the pose of snakelike soft robots. ACS Appl Mater Interfaces. 2018;10(27):23208-23217. doi: 10.1021/acsami.8b06903
- Peng X, Kuang X, Roach DJ, et al. Integrating digital light processing with direct ink writing for hybrid 3D printing of functional structures and devices. Addit Manuf. 2021;40:101911. doi: 10.1016/j.addma.2021.101911
- Park NG. Perovskite solar cells: An emerging photovoltaic technology. Mater Today. 2015;18(2):65-72. doi: 10.1016/j.mattod.2014.07.007
- Zhang X, Turiansky ME, Shen JX, Van de Walle CG. Defect tolerance in halide perovskites: A first-principles perspective. J Appl Phys. 2022;131(9):090901. doi: 10.1063/5.0083686
- Motta C, El-Mellouhi F, Sanvito S. Charge carrier mobility in hybrid halide perovskites. Sci Rep. 2015;5(1):12746. doi: 10.1038/srep12746
- Wei Z, Chen H, Yan K, Yang S. Inkjet printing and instant chemical transformation of a CH3NH3PbI3/nanocarbon electrode and interface for planar perovskite solar cells. Angew Chem Int Ed Engl. 2014;53(48):13239-13243. doi: 10.1002/anie.201408638
- Mathies F, Abzieher T, Hochstuhl A, et al. Multipass inkjet printed planar methylammonium lead iodide perovskite solar cells. J Mater Chem A. 2016;4(48):19207-19213. doi: 10.1039/c6ta07972e
- Choi JW, Woo HC, Huang X, et al. Organic-inorganic hybrid perovskite quantum dots with high PLQY and enhanced carrier mobility through crystallinity control by solvent engineering and solid-state ligand exchange. Nanoscale. 2018;10(28):13356-13367. doi: 10.1039/c8nr00806j
- Liang H, Yuan F, Johnston A, et al. High color purity lead-free perovskite light-emitting diodes via sn stabilization. Adv Sci (Weinh). 2020;7(8):1903213. doi: 10.1002/advs.201903213
- Bak T, Kim K, Seo E, et al. Accelerated design of high-efficiency lead-free tin perovskite solar cells via machine learning. Int J Precis Eng Manuf Green Technol. 2023;10(1):109-121. doi: 10.1007/s40684-022-00417-z
- Ahmed T, Seth S, Samanta A. Boosting the photoluminescence of CsPbX3 (X=Cl, Br, I) perovskite nanocrystals covering a wide wavelength range by postsynthetic treatment with tetrafluoroborate salts. Chem Mat. 2018;30(11):3633-3637. doi: 10.1021/acs.chemmater.8b01235
- Li F, Liu SF, Liu W, et al. 3D printing of inorganic nanomaterials by photochemically bonding colloidal nanocrystals. Science. 2023;381(6665):1468-1474. doi: 10.1126/science.adg6681
- Liu J, Shabbir B, Wang C, et al. Flexible, printable soft-X-ray detectors based on all-inorganic perovskite quantum dots. Adv Mater. 2019;31(30):1901644. doi: 10.1002/adma.201901644
- Zheng J, Zhang M, Lau CFJ, et al. Spin-coating free fabrication for highly efficient perovskite solar cells. Sol Energy Mater Sol Cells. 2017;168:165-171. doi: 10.1016/j.solmat.2017.04.029
- Bishop JE, Smith JA, Lidzey DG. Development of spray-coated perovskite solar cells. ACS Appl Mater Interfaces. 2020;12(43):48237-48245. doi: 10.1021/acsami.0c14540
- Kim JH, Williams ST, Cho N, Chueh CC, Jen AKY. Enhanced environmental stability of planar heterojunction perovskite solar cells based on blade‐coating. Adv Energy Mater. 2014;5(4):1401229. doi: 10.1002/aenm.201401229
- Zhang L, Chen S, Wang X, et al. Ambient inkjet‐printed high‐efficiency perovskite solar cells: Manipulating the spreading and crystallization behaviors of picoliter perovskite droplets. Sol RRL. 2021;5(5):2100106. doi: 10.1002/solr.202100106
- Chen M, Zhou Z, Hu S, et al. 3D printing of arbitrary perovskite nanowire heterostructures. Adv Funct Mater. 2023;33(15):2212146. doi: 10.1002/adfm.202212146
- Zhu M, Duan Y, Liu N, et al. Electrohydrodynamically printed high‐resolution full‐color hybrid perovskites. Adv Funct Mater. 2019;29(35):1903294. doi: 10.1002/adfm.201903294
- Tang Y, Liu B, Yuan H, et al. In situ synthesis of MAPbX3 perovskite quantum dot-polycaprolactone composites for fluorescent 3D printing filament. J Alloy Compd. 2022;916:164961. doi: 10.1016/j.jallcom.2022.164961
- Jeon H, Wajahat M, Park S, et al. 3D Printing of luminescent perovskite quantum dot-polymer architectures. Adv Funct Mater. 2024;2024:2400594. doi: 10.1002/adfm.202400594
- Eggers H, Schackmar F, Abzieher T, et al. Inkjet‐printed micrometer‐thick perovskite solar cells with large columnar grains. Adv Energy Mater. 2019;10(6):1903184. doi: 10.1002/aenm.201903184
- Wang Q, Zhang G, Zhang H, Duan Y, Yin Z, Huang Y. High‐resolution, flexible, and full‐color perovskite image photodetector via electrohydrodynamic printing of ionic‐liquid‐based ink. Adv Funct Mater. 2021;31(28):2100857. doi: 10.1002/adfm.202100857
- Satake K, Sato Y, Narazaki K, et al. Fabrication of perovskite nanocrystal light-emitting diodes via inkjet printing with high-temperature annealing. ACS Appl Opti Mater. 2022;1(1):282-288. doi: 10.1021/acsaom.2c00053
- Liu H, Shi G, Khan R, et al. Large‐area flexible perovskite light‐emitting diodes enabled by inkjet printing. Adv Mater. 2024;36(8):2309921. doi: 10.1002/adma.202309921
- Liu Y, Li F, Qiu L, et al. Fluorescent Microarrays of in situ crystallized perovskite nanocomposites fabricated for patterned applications by using inkjet printing. ACS Nano. 2019;13(2):2042-2049. doi: 10.1021/acsnano.8b08582
- Yang Z, Zhou S, Zu J, Inman D. High-performance piezoelectric energy harvesters and their applications. Joule. 2018;2(4):642-697. doi: 10.1016/j.joule.2018.03.011
- Wu Y, Ma Y, Zheng H, Ramakrishna S. Piezoelectric materials for flexible and wearable electronics: A review. Mater Des. 2021;211:110164. doi: 10.1016/j.matdes.2021.110164
- Smith GL, Pulskamp JS, Sanchez LM, et al. PZT‐based piezoelectric MEMS technology. J Am Ceram Soc. 2012;95(6):1777-1792. doi: 10.1111/j.1551-2916.2012.05155.x
- Park KI, Xu S, Liu Y, et al. Piezoelectric BaTiO(3) thin film nanogenerator on plastic substrates. Nano Lett. 2010;10(12):4939-4943. doi: 10.1021/nl102959k
- Ueberschlag P. PVDF piezoelectric polymer. Sens Rev. 2001;21(2):118-126. doi: 10.1108/02602280110388315
- Bhavanasi V, Kumar V, Parida K, Wang J, Lee PS. Enhanced piezoelectric energy harvesting performance of flexible PVDF-TrFE bilayer films with graphene oxide. ACS Appl Mater Interfaces. 2016;8(1):521-529. doi: 10.1021/acsami.5b09502
- Zhilun G, Longtu L, Suhua G, Xiaowen Z. Low‐temperature sintering of lead‐based piezoelectric ceramics. J Am Ceram Soc. 1989;72(3):486-491. doi: 10.1111/j.1151-2916.1989.tb06160.x
- Wang H, Godara M, Chen Z, Xie H. A one-step residue-free wet etching process of ceramic PZT for piezoelectric transducers. Sens Actuator A Phys. 2019;290:130-136. doi: 10.1016/j.sna.2019.03.028
- Amagata Y, Mihara S, Nishioki N, Higuchi T. A New Fabrication Method for Microactuators with Piezoelectric Thin Film Using Precision Cutting Technique. United States: IEEE; 1996. p. 307-311. doi: 10.1109/MEMSYS.1996.493999
- Megdich A, Habibi M, Laperrière L. A review on 3D printed piezoelectric energy harvesters: Materials, 3D printing techniques, and applications. Mater Today Commun. 2023;35:105541. doi: 10.1016/j.mtcomm.2023.105541
- Zhang J, Ye S, Liu H, et al. 3D printed piezoelectric BNNTs nanocomposites with tunable interface and microarchitectures for self-powered conformal sensors. Nano Energy. 2020;77:105300. doi: 10.1016/j.nanoen.2020.105300
- Song L, Dai R, Li Y, Wang Q, Zhang C. Polyvinylidene fluoride energy harvester with boosting piezoelectric performance through 3D printed biomimetic bone structures. ACS Sustain Chem Eng. 2021;9(22):7561-7568. doi: 10.1021/acssuschemeng.1c01305
- Malakooti MH, Julé F, Sodano HA. Printed nanocomposite energy harvesters with controlled alignment of barium titanate nanowires. ACS Appl Mater Interfaces. 2018;10(44):38359-38367. doi: 10.1021/acsami.8b13643
- Liu CL, Du Q, Zhang C, Wu JM, Zhang G, Shi YS. Fabrication and properties of BaTiO3 ceramics via digital light processing for piezoelectric energy harvesters. Addit Manuf. 2022;56:102940. doi: 10.1016/j.addma.2022.102940
- Pabst O, Perelaer J, Beckert E, Schubert US, Eberhardt R, Tünnermann A. All inkjet-printed piezoelectric polymer actuators: Characterization and applications for micropumps in lab-on-a-chip systems. Org Electron. 2013;14(12):3423- 3429. doi: 10.1016/j.orgel.2013.09.009
- Maity K, Mondal A, Saha MC. Cellulose nanocrystal-based all-3D-printed pyro-piezoelectric nanogenerator for hybrid energy harvesting and self-powered cardiorespiratory monitoring toward the human-machine interface. ACS Appl Mater Interfaces. 2023;15(11):13956-13970. doi: 10.1021/acsami.2c21680
- Islam MN, Rupom RH, Adhikari PR, et al. Boosting piezoelectricity by 3D printing PVDF‐MoS2 composite as a conformal and high‐sensitivity piezoelectric sensor. Adv Funct Mater. 2023;33(42):2302946. doi: 10.1002/adfm.202302946
- Nassar H, Khandelwal G, Chirila R, et al. Fully 3D printed piezoelectric pressure sensor for dynamic tactile sensing. Addit Manuf. 2023;71:103601. doi: 10.1016/j.addma.2023.103601
- Cui H, Yao D, Hensleigh R, et al. Design and printing of proprioceptive three-dimensional architected robotic metamaterials. Science. 2022;376(6599):1287-1293. doi: 10.1126/science.abn0090
- Burton M, Howells G, Atoyo J, Carnie M. Printed thermoelectrics. Adv Mater. 2022;34(18):e2108183. doi: 10.1002/adma.202108183
- Lee H, Chidambaram Seshadri R, Han SJ, Sampath S. TiO 2-X based thermoelectric generators enabled by additive and layered manufacturing. Appl Energy. 2017;192:24-32. doi: 10.1016/j.apenergy.2017.02.001
- Shin S, Kumar R, Roh JW, et al. High-performance screen-printed thermoelectric films on fabrics. Sci Rep. 2017;7(1):7317. doi: 10.1038/s41598-017-07654-2
- Juntunen T, Jussila H, Ruoho M, et al. Inkjet printed large‐area flexible few‐layer graphene thermoelectrics. Adv Funct Mater. 2018;28(22):1800480. doi: 10.1002/adfm.201800480
- Bulman G, Barletta P, Lewis J, et al. Superlattice-bsed thin-film thermoelectric modules with high cooling fluxes. Nat Commun. 2016;7:10302. doi: 10.1038/ncomms10302
- Zhao X, Han W, Zhao C, et al. Fabrication of transparent paper-based flexible thermoelectric generator for wearable energy harvester using modified distributor printing technology. ACS Appl Mater Interfaces. 2019;11(10):10301-10309. doi: 10.1021/acsami.8b21716
- Liu H, Li G, Zhao X, Ma X, Shen C. Investigation of the impact of the thermoelectric geometry on the cooling performance and thermal-mechanic characteristics in a thermoelectric cooler. Energy. 2023;267:126471. doi: 10.1016/j.energy.2022.126471
- Li P, Nie XL, Fang WB, et al. Fabrication and planar cooling performance of flexible Bi0.5Sb1.5Te3/epoxy composite thermoelectric films. J Inorg Mater. 2019;34(6):679. doi: 10.15541/jim20180528
- Lu Z, Layani M, Zhao X, et al. Fabrication of flexible thermoelectric thin film devices by inkjet printing. Small. 2014;10(17):3551-3554. doi: 10.1002/smll.201303126
- Venkatasubramanin R, Siivola E, Colpitts T, O’Quinn B. Thin-film thermoelectric devices with high room-temperature figures of merit. Nature. 2001;413:597-602. doi: 10.1038/35098012
- Du J, Zhang B, Jiang M, et al. Inkjet printing flexible thermoelectric devices using metal chalcogenide nanowires. Adv Funct Mater. 2023;33(26):2213564. doi: 10.1002/adfm.202213564
- Mytafides CK, Tzounis L, Karalis G, Formanek P, Paipetis AS. High-power all-carbon fully printed and wearable SWCNT-based organic thermoelectric generator. ACS Appl Mater Interfaces. 2021;13(9):11151-11165. doi: 10.1021/acsami.1c00414
- Schroeder V, Savagatrup S, He M, Lin S, Swager TM. Carbon nanotube chemical sensors. Chem Rev. 2019;119(1):599-663. doi: 10.1021/acs.chemrev.8b00340
- Jung ID, Kim M, Gao C, et al. Selective ion sweeping on prussian blue analogue nanoparticles and activated carbon for electrochemical kinetic energy harvesting. Nano Letters. 2020;20(3):1800-1807. doi: 10.1021/acs.nanolett.9b05029
- Sidhu JS, Misra A, Bhardwaj A. Fabrication of Carbon Nanotube Components Using 3D Printing: Review. In: Mater Today Proceedings; 2023. doi: 10.1016/j.matpr.2023.08.040
- Lawes S, Riese A, Sun Q, Cheng N, Sun X. Printing nanostructured carbon for energy storage and conversion applications. Carbon. 2015;92:150-176. doi: 10.1016/j.carbon.2015.04.008
- Alshammari AS, Alenezi MR, Lai KT, Silva SRP. Inkjet printing of polymer functionalized CNT gas sensor with enhanced sensing properties. Mater Lett. 2017;189:299-302. doi: 10.1016/j.matlet.2016.11.033
- Beniwal A, Ganguly P, Aliyana AK, Khandelwal G, Dahiya R. Screen-printed graphene-carbon ink based disposable humidity sensor with wireless communication. Sens Actuators B Chem. 2023;374:132731. doi: 10.1016/j.snb.2022.132731
- Hassan K, Tung TT, Stanley N, et al. Graphene ink for 3D extrusion micro printing of chemo-resistive sensing devices for volatile organic compound detection. Nanoscale. 2021;13(10):5356-5368. doi: 10.1039/d1nr00150g
- Anca FB, Filipescu M, Voicu SI, Lippert T, Palla- Papavlu A. Facile fabrication of hybrid carbon nanotube sensors by laser direct transfer. Nanomaterials (Basel). 2021;11(10):2604. doi: 10.3390/nano11102604
- Wajahat M, Lee S, Kim JH, et al. Flexible strain sensors fabricated by meniscus-guided printing of carbon nanotube-polymer composites. ACS Appl Mater Interfaces. 2018;10(23):19999-20005. doi: 10.1021/acsami.8b04073
- Alsharari M, Chen B, Shu W. 3D printing of highly stretchable and sensitive strain sensors using graphene based composites. Proceedings. 2018;2:792. doi: 10.3390/proceedings2130792
- Ren Y, Meng F, Zhang S, et al. CNT@MnO2 composite ink toward a flexible 3D printed micro‐zinc‐ion battery. Carbon Energy. 2022;4(3):446-457. doi: 10.1002/cey2.177
- Kushwaha A, Jangid MK, Bhatt BB, Mukhopadhyay A, Gupta D. Inkjet-printed environmentally friendly graphene film for application as a high-performance anode in Li-Ion batteries. ACS Appl Energy Mater. 2021;4(8):7911-7921. doi: 10.1021/acsaem.1c01249
- Wang J, Sun Q, Gao X, et al. Toward high areal energy and power density electrode for Li-Ion batteries via optimized 3D printing approach. ACS Appl Mater Interfaces. 2018;10(46):39794-39801. doi: 10.1021/acsami.8b14797
- Chen Q, Xu R, He Z, Zhao K, Pan L. Printing 3D gel polymer electrolyte in lithium-ion microbattery using stereolithography. J Electrochem Soc. 2017;164:X18. doi: 10.1149/2.0651709jes