Porous titanium alloys for medical application: Progress in preparation process and surface modification research
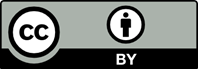
Excellent mechanical properties and biocompatibility are the most sought-after attributes in biomedical materials for the regeneration of damaged tissues. However, conventional dense titanium alloys possess a modulus significantly higher than that of human tissues, leading to potential stress-shielding effects. Medical porous titanium alloys can reduce the elastic modulus of the material, promote tissue fixation and vascular regeneration, and improve the suitability for human tissue properties. With the continuous development of technology, the preparation process of porous titanium alloys has undergone a series of multifaceted transformations and improvements in the aspects of powder sintering, fiber preparation, and additive manufacturing processes, and its structural characteristics and mechanical properties are constantly evolving in a controllable direction. Alongside the enhancement of the material’s mechanical properties through porous design, optimization of the properties at the implant-tissue interface also leads to improved antimicrobial and osteogenic properties of porous titanium. Due to the complex internal structure of porous titanium alloys, surface modification is mainly carried out in fluid media, which is realized by morphological modification and the introduction of functional substances. Over time, the surface modification of porous titanium alloys for medical applications has progressed from morphological modification and introduction of chemical composition to the loading of bioactive substances. This evolution aims to enhance safety and efficiency in the use of these materials. This paper reviews the preparation and surface modification processes of porous titanium alloys for medical use and summarizes the advantages, disadvantages, and influencing factors among different processes, with a view to providing new ideas for the development of porous implants for medical use.
- Evans JT, Evans JP, Walker RW, Blom AW, Whitehouse MR, Sayers A. How long does a hip replacement last? A systematic review and meta-analysis of case series and national registry reports with more than 15 years of follow-up. Lancet. 2019;393:647-654. doi: 10.1016/S0140-6736(18)31665-9.
- Gupta K, Meena K. Artificial bone scaffolds and bone joints by additive manufacturing: A review. Bioprinting. 2023;31:e00268. doi: 10.1016/j.bprint.2023.e00268
- Denry I, Kelly JR. Emerging ceramic-based materials for dentistry. J Dent Res. 2014;93:1235-1242. doi: 10.1177/0022034514553627.
- Dong T, Duan C, Wang S, et al. Multifunctional surface with enhanced angiogenesis for improving long-term osteogenic fixation of poly(ether ether ketone) implants. ACS Appl Mater Interfaces. 2020;12:14971-14982. doi: 10.1021/acsami.0c02304
- Wang N, Ma Y, Shi H, Song Y, Guo S, Yang S. Mg-, Zn-, and Fe-based alloys with antibacterial properties as orthopedic implant materials. Front Bioeng Biotechnol. 2022;10:888084. doi: 10.3389/fbioe.2022.888084
- Liang W, Zhou C, Zhang H, et al. Recent advances in 3D printing of biodegradable metals for orthopaedic applications. J Biol Eng. 2023;17:56. doi: 10.1186/s13036-023-00371-7
- Song C, Liu L, Deng Z, et al. Research progress on the design and performance of porous titanium alloy bone implants. J Mater Res Technol. 2023;23:2626-2641. doi: 10.1016/j.jmrt.2023.01.155
- Guo AXY, Cheng L, Zhan S, et al. Biomedical applications of the powder‐based 3D printed titanium alloys: A review. J Mater Sci Technol. 2022;125:252-264. doi: 10.1016/j.jmst.2021.11.084
- Gao C, Li C, Wang C, et al. Advances in the induction of osteogenesis by zinc surface modification based on titanium alloy substrates for medical implants. J Alloy Compd. 2017;726:1072-1084. doi: 10.1016/j.jallcom.2017.08.078
- Sun Y, Hu W, Wu C, et al. Research progress on mechanical properties of 3D printed biomedical titanium alloys. J Mater Eng Perform. 2023;32:9489-9503. doi: 10.1007/s11665-023-08248-y
- Liu R, Su Y, Yang W, et al. Novel design and optimization of porous titanium structure for mandibular reconstruction. Appl Bionics Biomech. 2022;2022:8686670. doi: 10.1155/2022/8686670
- Long S, Zhu J, Jing Y, He S, Cheng L, Shi Z. A comprehensive review of surface modification techniques for enhancing the biocompatibility of 3D-printed titanium implants. Coatings. 2023;13:1917. doi: 10.3390/coatings13111917
- Asri RIM, Harun WSM, Samykano M, et al. Corrosion and surface modification on biocompatible metals: A review. Mater Sci Eng C Mater Biol Appl. 2017;77:1261-1274. doi: 10.1016/j.msec.2017.04.102
- Ottria L, Lauritano D, Bassi MA, et al. Mechanical, chemical and biological aspects of titanium and titanium alloys in implant dentistry. J Biol Regul Homeost Agents. 2018;32:81- 90.
- Sheng X, Wang A, Wang Z, Liu H, Wang J, Li C. Advanced surface modification for 3D-printed titanium alloy implant interface functionalization. Front Bioeng Biotechnol. 2022;10:850110. doi: 10.3389/fbioe.2022.850110
- Raman RKS, Wen C, Loeffler JF. Human Body-fluid-assisted fracture of zinc alloys as biodegradable temporary implants: Challenges, research needs and way forward. Materials. 2023;16:4984. doi: 10.3390/ma16144984
- Dziaduszewska M, Zieliński A. Structural and material determinants influencing the behavior of porous Ti and its alloys made by additive manufacturing techniques for biomedical applications. Materials. 2021;14:712. doi: 10.3390/ma14040712
- Khodaei M, Fathi M, Meratian M, Savabi O. The effect of porosity on the mechanical properties of porous titanium scaffolds: Comparative study on experimental and analytical values. Mater Res Express. 2018;5:055401. doi: 10.1088/2053-1591/aabfa2
- Xiong YZ, Gao RN, Zhang H, Dong LL, Li JT, Li X. Rationally designed functionally graded porous Ti6Al4V scaffolds with high strength and toughness built via selective laser melting for load-bearing orthopedic applications. J Mech Behav Biomed Mater. 2020;104:103673. doi: 10.1016/j.jmbbm.2020.103673
- Lv Y, Wang B, Liu G, et al. Metal material, properties and design methods of porous biomedical scaffolds for additive manufacturing: A review. Front Bioeng Biotechnol. 2021;9:641130. doi: 10.3389/fbioe.2021.641130
- Wei G, Tan M, Attarilar S, et al. An overview of surface modification, a way toward fabrication of nascent biomedical Ti–6Al–4V alloys. J Mater Res Technol. 2023;24:5896-5921. doi: 10.1016/j.jmrt.2023.04.046
- Oesterle W, Klaffke D, Griepentrog M, Gross U, Kranz I, Knabe C. Potential of wear resistant coatings on Ti-6Al-4V for artificial hip joint bearing surfaces. Wear. 2008;264:505- 517. doi: 10.1016/j.wear.2007.04.001
- Bobbert FSL, Lietaert K, Eftekhari AA, et al. Additively manufactured metallic porous biomaterials based on minimal surfaces: A unique combination of topological, mechanical, and mass transport properties. Acta Biomater. 2017;53:572-584. doi: 10.1016/j.actbio.2017.02.024
- Lv Y, Liu G, Wang B, et al. Pore strategy design of a novel NiTi-Nb biomedical porous scaffold based on a triply periodic minimal surface. Front Bioeng Biotechnol. 2022;10:910475. doi: 10.3389/fbioe.2022.910475
- Zhang T, Wang W, Liu J, Wang L, Tang Y, Wang K. A review on magnesium alloys for biomedical applications. Front Bioeng Biotechnol. 2022;10:953344. doi: 10.3389/fbioe.2022.953344
- Sidhu SS, Singh H, Gepreel MAH. A review on alloy design, biological response, and strengthening of β-titanium alloys as biomaterials. Mater Sci Eng C Mater Biol Appl. 2021;121:111661. doi: 10.1016/j.msec.2020.111661
- Zhang T, Fan Q, Ma X, et al. Effect of laser remelting on microstructural evolution and mechanical properties of Ti-35Nb-2Ta-3Zr alloy. Mater Lett. 2019;253:310-313. doi: 10.1016/j.matlet.2019.06.105
- Wang L, Lin Z, Wang X, et al. Effect of aging treatment on microstructure and mechanical properties of Ti27Nb2Ta3Zr β titanium alloy for implant applications. Mater Trans. 2014;55:141-146. doi: 10.2320/matertrans.M2013187
- Yuan L, Ding S, Wen C. Additive manufacturing technology for porous metal implant applications and triple minimal surface structures: A review. Bioactive Mater. 2019;4:56-70. doi: 10.1016/j.bioactmat.2018.12.003
- Zhao F, Wang J, Guo H, Liu S, He W. The effects of surface properties of nanostructured bone repair materials on their performances. J Nanomater. 2015;2015:893545. doi: 10.1155/2015/893545
- Cao S, Ma N, Zhang Y, Bo R, Lu Y. Fabrication, mechanical properties, and multifunctionalities of particle reinforced foams: A review. Thin Walled Struct. 2023;186:110678. doi: 10.1016/j.tws.2023.110678
- Rodriguez-Contreras A, Punset M, Calero JA, Javier Gil F, Ruperez E, Maria Manero J. Powder metallurgy with space holder for porous titanium implants: A review. J Mater Sci Technol. 2021;76:129-149. doi: 10.1016/j.jmst.2020.11.005
- Ryan G, Pandit A, Apatsidis DP. Fabrication methods of porous metals for use in orthopaedic applications. Biomaterials. 2006;27:2651-2670. doi: 10.1016/j.biomaterials.2005.12.002
- Majumdar T, Eisenstein N, Frith JE, Cox SC, Birbilis N. Additive manufacturing of titanium alloys for orthopedic applications: A materials science viewpoint. Adv Eng Mater. 2018;20:1800172. doi: 10.1002/adem.201800172
- Babaie E, Bhaduri SB. Fabrication aspects of porous biomaterials in orthopedic applications: A review. ACS Biomater Sci Eng. 2018;4:1-39. doi: 10.1021/acsbiomaterials.7b00615
- Luo SD, Qian M. Microwave processing of titanium and titanium alloys for structural, biomedical and shape memory applications: Current status and challenges. Mater Manuf Process. 2018;33:35-49. doi: 10.1080/10426914.2016.1257800
- Oh IH, Nomura N, Masahashi N, Hanada S. Mechanical properties of porous titanium compacts prepared by powder sintering. Scripta Mater. 2003;49:1197-1202. doi: 10.1016/j.scriptamat.2003.08.018
- Torres Y, Lascano S, Bris J, Pavón J, Rodriguez JA. Development of porous titanium for biomedical applications: A comparison between loose sintering and space-holder techniques. Mater Sci Eng C Mater Biol Appl. 2014;37:148- 155. doi: 10.1016/j.msec.2013.11.036
- Annur D, Kartika I, Sudiro T, Supriadi S, Suharno B. Microstructure, mechanical properties, and in vitro studies of porous titanium obtained by spark plasma sintering. Trans Indian Inst Metals. 2022;75:3067-3076. doi: 10.1007/s12666-022-02680-9
- Saadati A, Aghajani H. Fabrication of porous NiTi biomedical alloy by SHS method. J Mater Sci Mater Med. 2019;30:92. doi: 10.1007/s10856-019-6296-9
- Han Q, Wang C, Chen H, Zhao X, Wang J. Porous tantalum and titanium in orthopedics: A review. ACS Biomater Sci Eng. 2019;5:5798-5824. doi: 10.1021/acsbiomaterials.9b00493
- Lascano S, Arevalo C, Montealegre-Melendez I, et al. Porous titanium for biomedical applications: Evaluation of the conventional powder metallurgy frontier and space-holder technique. Appl Sci. 2019;9:982. doi: 10.3390/app9050982
- Nugroho AW, Leadbeater G, Davies IJ. Fabrication and characterization of the porous titanium alloy by argon filled pore expansion technique. IOP Conf Ser Mater Sci Eng. 2018;403:012096. doi: 10.1088/1757-899X/403/1/012096
- Chen YJ, Feng B, Zhu YP, Weng J, Wang JX, Lu X. Fabrication of porous titanium implants with biomechanical compatibility. Mater Lett. 2009;63:2659-2661. doi: 10.1016/j.matlet.2009.09.029
- Rao X, Chu CL, Zheng YY. Phase composition, microstructure, and mechanical properties of porous Ti–Nb–Zr alloys prepared by a two-step foaming powder metallurgy method. J Mech Behav Biomed Mater. 2014;34:27-36. doi: 10.1016/j.jmbbm.2014.02.001
- Ahn MK, Jo IH, Koh YH, Kim HE. Production of highly porous titanium (Ti) scaffolds by vacuum-assisted foaming of titanium hydride (TiH2) suspension. Mater Lett. 2014;120:228-31. doi: 10.1016/j.matlet.2014.01.065
- Gonzalez, Z, Molero, E, Sanchez, J, Ferrari, B. Processing of titanium porous bodies by foaming of gelled aqueous suspensions of powders. Preprints 2021, 2021020099. doi: 10.20944/preprints202102.0099.v1
- Haghjoo R, Sadrnezhaad SK, Hassanzadeh-Nemati N. Synthesis, characterization, and biological studies of sintered porous titanium with three different pore morphologies. Int J Mater Res. 2023;114:43-53. doi: 10.1515/ijmr-2022-0053
- Luo H, Zhao J, Du H, Yin W, Qu Y. Effect of Mg powder’s particle size on structure and mechanical properties of Ti foam synthesized by space holder technique. Materials. 2022;15:8863. doi: 10.3390/ma15248863
- Yang G, Xu B, Lei X, et al. Preparation of porous titanium by direct in-situ reduction of titanium sesquioxide. Vacuum. 2018;157:453-457. doi: 10.1016/j.vacuum.2018.09.021
- Chen Z, Wu C, Liu X, Shen T, Zhang L. Fabricating honeycomb titanium by freeze casting and anodizing for biomedical applications. Adv Eng Mater. 2022;24:2101088. doi: 10.1002/adem.202101088
- Li J, Li Z, Wang Q, et al. Sintered porous Ti6Al4V scaffolds incorporated with recombinant human bone morphogenetic protein-2 microspheres and thermosensitive hydrogels can enhance bone regeneration. RSC Adv. 2019;9:1541-1550. doi: 10.1039/C8RA10200G
- Yang D, Guo Z, Shao H, Liu X, Ji Y. Mechanical properties of porous Ti-Mo and Ti-Nb alloys for biomedical application by gelcasting. Procedia Eng.2012;36:160-167. doi: 10.1016/j.proeng.2012.03.025
- Biasetto L, De Moraes EG, Colombo P, Bonollo F. Ovalbumin as foaming agent for Ti6Al4V foams produced by gelcasting. J Alloys Compd. 2016;687:839-844. doi: 10.1016/j.jallcom.2016.06.218
- Liu P, Tan Q, Wu L, He G. Compressive and pseudo-elastic hysteresis behavior of entangled titanium wire materials. Mater Sci Eng A. 2010;527:3301-3309. doi: 10.1016/j.msea.2010.02.071
- Wang L, Xie L, Zhang LC, et al. Microstructure evolution and superelasticity of layer-like NiTiNb porous metal prepared by eutectic reaction. Acta Mater. 2018;143:214-226. doi: 10.1016/j.actamat.2017.10.021
- Li F, Li J, Xu G, Liu G, Kou H, Zhou L. Fabrication, pore structure and compressive behavior of anisotropic porous titanium for human trabecular bone implant applications. J Mech Behav Biomed Mater. 2015;46:104-114. doi: 10.1016/j.jmbbm.2015.02.023
- Ngo TD, Kashani A, Imbalzano G, Nguyen KTQ, Hui D. Additive manufacturing (3D printing): A review of materials, methods, applications and challenges. Compos B Eng. 2018;143:172-196. doi: 10.1016/j.compositesb.2018.02.012
- Agarwal R, García AJ. Biomaterial strategies for engineering implants for enhanced osseointegration and bone repair. Adv Drug Deliv Rev. 2015;94:53-62. doi: 10.1016/j.addr.2015.03.013
- Structural Characteristics and Mechanical Behavior of Selective Laser Sintered Porous Ti-6Mo Alloy for Biomedical Applications-All Databases, (n.d.). Available from: http:// webvpn.swu.tsgvip.top/https/537775736869676568616f78 756565212abc50b4738e8888c9482a5750aefc5fc6e25954c3 8c5bb888/wos/alldb/full-record/WOS:000379195400020 [Last accessed on 2024 Mar 03].
- Davoodi E, Montazerian H, Mirhakimi AS, et al. Additively manufactured metallic biomaterials. Bioactive Mater. 2022;15:214-249. doi: 10.1016/j.bioactmat.2021.12.027
- Wang C, Huang W, Zhou Y, et al. 3D printing of bone tissue engineering scaffolds. Bioactive Mater. 2020;5:82-91. doi: 10.1016/j.bioactmat.2020.01.004
- Huang S, Kumar P, Yeong WY, Narayan RL, Ramamurty U. Fracture behavior of laser powder bed fusion fabricated Ti41Nb via in-situ alloying. Acta Mater. 2022;225:117593. doi: 10.1016/j.actamat.2021.117593
- Huang S, Narayan RL, Tan JHK, Sing SL, Yeong WL. Resolving the porosity-unmelted inclusion dilemma during in-situ alloying of Ti34Nb via laser powder bed fusion. Acta Mater. 2021;204:116522. doi: 10.1016/j.actamat.2020.116522
- Zadpoor AA. Additively manufactured porous metallic biomaterials. J Mater Chem B. 2019;7:4088-4117. doi: 10.1039/C9TB00420C
- Bikas H, Stavropoulos P, Chryssolouris G. Additive manufacturing methods and modelling approaches: A critical review. Int J Adv Manuf Technol. 2016;83:389-405. doi: 10.1007/s00170-015-7576-2
- Slámečka K, Kashimbetova A, Pokluda J, et al. Fatigue behaviour of titanium scaffolds with hierarchical porosity produced by material extrusion additive manufacturing. Mater Des. 2023;225:111453.
- Sing SL, An J, Yeong WY, Wiria FE. Laser and electron‐beam powder‐bed additive manufacturing of metallic implants: A review on processes, materials and designs. J Orthop Res. 2016;34:369-385. doi: 10.1002/jor.23075
- Qu H. Additive manufacturing for bone tissue engineering scaffolds. Mater Today Commun. 2020;24:101024. doi: 10.1016/j.mtcomm.2020.101024
- Hara D, Nakashima Y, Sato T, et al. Bone bonding strength of diamond-structured porous titanium-alloy implants manufactured using the electron beam-melting technique. Mater Sci Eng C Mater Biol Appl. 2016;59:1047-1052. doi: 10.1016/j.msec.2015.11.025
- Sing SL, Yeong WY, Wiria FE. Selective laser melting of titanium alloy with 50 wt% tantalum: Microstructure and mechanical properties. J Alloys Compd. 2016;660:461-470. doi: 10.1016/j.jallcom.2015.11.141
- Ataee A, Li Y, Wen C. A comparative study on the nanoindentation behavior, wear resistance and in vitro biocompatibility of SLM manufactured CP–Ti and EBM manufactured Ti64 gyroid scaffolds. Acta Biomater. 2019;97:587-596. doi: 10.1016/j.actbio.2019.08.008
- Gómez S, Vlad MD, López J, Fernández E. Design and properties of 3D scaffolds for bone tissue engineering. Acta Biomater. 2016;42:341-350. doi: 10.1016/j.actbio.2016.06.032
- Zieliński TG, Opiela KC, Pawłowski P, et al. Reproducibility of sound-absorbing periodic porous materials using additive manufacturing technologies: Round robin study. Addit Manufact. 2020;36:101564. doi: 10.1016/j.addma.2020.101564
- Kou XY, Tan ST. A simple and effective geometric representation for irregular porous structure modeling. Comput Aided Des. 2010;42:930-941. doi: 10.1016/j.cad.2010.06.006
- Zhao J, Zhang M, Zhu Y, Li X, Wang L, Hu C. Concurrent optimization of additive manufacturing fabricated lattice structures for natural frequencies. Int J Mech Sci. 2019;163:105153. doi: 10.1016/j.ijmecsci.2019.105153
- Ma S, Song K, Lan J, Ma L. Biological and mechanical property analysis for designed heterogeneous porous scaffolds based on the refined TPMS. J Mech Behav Biomed Mater. 2020;107:103727. doi: 10.1016/j.jmbbm.2020.103727
- Lv Y, Wang B, Liu G, et al. Design of bone-like continuous gradient porous scaffold based on triply periodic minimal surfaces. J Mater Res Technol. 2022;21:3650-3665. doi: 10.1016/j.jmrt.2022.10.160
- Lv Y, Guo J, Zhang Q, Wei G, Yu H. Design of low elastic modulus and high strength TC4 porous scaffolds via new variable gradient strategies. Mater Lett. 2022;325:132616. doi: 10.1016/j.matlet.2022.132616
- Zhao D, Huang Y, Ao Y, et al. Effect of pore geometry on the fatigue properties and cell affinity of porous titanium scaffolds fabricated by selective laser melting. J Mech Behav Biomed Mater. 2018;88:478-487. doi: 10.1016/j.jmbbm.2018.08.048
- Novel‐Ink‐Based Direct Ink Writing of Ti6al4v Scaffolds with Sub‐300 μm Structural Pores for Superior Cell Proliferation And Differentiation - Xu - Advanced Healthcare Materials. Wiley Online Library, (n.d.). Available from: https:// onlinelibrary.wiley.com/doi/abs/10.1002/adhm.202302396 [Last accessed on 2024 Jan 13.
- Zhao G, Shao X, Zhang Q, et al. Porous bio-high entropy alloy scaffolds fabricated by direct ink writing. J Mater Sci Technol. 2023;157:21-29. doi: 10.1016/j.jmst.2023.02.015
- Li Z, Yang F, Wang H, Li Y, Chen C, Guo Z. Direct ink writing of porous Ti6Al4V alloys via UV light curing. Adv Eng Mater. 2022;24:2200176. doi: 10.1002/adem.202200176
- Coffigniez M, Gremillard L, Balvay S, Lachambre J, Adrien J, Boulnat X. Direct-ink writing of strong and biocompatible titanium scaffolds with bimodal interconnected porosity. Addit Manuf. 2021;39:101859. doi: 10.1016/j.addma.2021.101859
- Direct ink Writing to Fabricate Porous Acetabular Cups from Titanium Alloy. Bio-Design and Manufacturing, (n.d.). Available from: https://link.springer.com/article/10.1007/ s42242-022-00222-2 [Last accessed on 2024 Jan 13.
- Xu Q, Gabbitas B, Matthews S, Zhang D. The development of porous titanium products using slip casting. J Mater Process Technol. 2013;213:1440-1446. doi: 10.1016/j.jmatprotec.2013.03.011
- Liu Y, Jiang G, He G. Enhancement of entangled porous titanium by BisGMA for load-bearing biomedical applications. Mater Sci Eng C Mater Biol Appl. 2016;61:37-41. doi: 10.1016/j.msec.2015.12.018
- Fiocchi J, Tuissi A, Biffi CA. Heat treatment of aluminium alloys produced by laser powder bed fusion: A review. Mater Des. 2021;204:109651. doi: 10.1016/j.matdes.2021.109651
- Chen LY, Liang SX, Liu Y, Zhang LC. Additive manufacturing of metallic lattice structures: Unconstrained design, accurate fabrication, fascinated performances, and challenges. Mater Sci Eng R Rep. 2021;146:100648. doi: 10.1016/j.mser.2021.100648
- Zhang LC, Chen LY, Wang L. Surface modification of titanium and titanium alloys: Technologies, developments, and future interests. Adv Eng Mater. 2020;22:1901258. doi: 10.1002/adem.201901258
- Fang Y, Wang Q, Yang Z, et al. An efficient approach to endow TiNbTaZr implant with osteogenic differentiation and antibacterial activity in vitro. Mater Des. 2022;221:110987. doi: 10.1016/j.matdes.2022.110987
- Guo Y, Hu B, Tang C, et al. Increased osteoblast function in vitro and in vivo through surface nanostructuring by ultrasonic shot peening. Int J Nanomed. 2015;10:4593-4603. doi: 10.2147/IJN.S83788
- Sypniewska J, Szkodo M. Influence of laser modification on the surface character of biomaterials: Titanium and its alloys-a review. Coatings. 2022;12:1371. doi: 10.3390/coatings12101371
- Surmeneva MA, Khrapov D, Prosolov K, et al. The influence of chemical etching on porous structure and mechanical properties of the Ti6AL4V Functionally Graded Porous Scaffolds fabricated by EBM. Mater Chem Phys. 2022;275:125217. doi: 10.1016/j.matchemphys.2021.125217
- Civantos A, Domínguez C, Pino RJ, et al. Designing bioactive porous titanium interfaces to balance mechanical properties and in vitro cells behavior towards increased osseointegration. Surf Coat Technol. 2019;368:162-174. doi: 10.1016/j.surfcoat.2019.03.001
- Wang D, He G, Tian Y, Ren N, Liu W, Zhang X. Dual effects of acid etching on cell responses and mechanical properties of porous titanium with controllable open‐porous structure. J Biomed Mater Res. 2020;108:2386-2395. doi: 10.1002/jbm.b.34571
- Montazerian M, Hosseinzadeh F, Migneco C, Fook MVL, Baino F. Bioceramic coatings on metallic implants: An overview. Ceram Int. 2022;48:8987-9005. doi: 10.1016/j.ceramint.2022.02.055
- Wang X, Li Y, Hodgson PD, Wen C. Biomimetic modification of porous TiNbZr alloy scaffold for bone tissue engineering. Tissue Eng A. 2010;16:309-316. doi: 10.1089/ten.tea.2009.0074
- Chudinova E, Koptyug A, Mukhortova Y, et al. Functionalization of additive-manufactured Ti6Al4V scaffolds with poly(allylamine hydrochloride)/poly(styrene sulfonate) bilayer microcapsule system containing dexamethasone. Mater Chem Phys. 2021;273:125099. doi: 10.1016/j.matchemphys.2021.125099
- Li L, Li Y, Yang L, et al. Polydopamine coating promotes early osteogenesis in 3D printing porous Ti6Al4V scaffolds. Ann Transl Med. 2019;7:240. doi: 10.21037/atm.2019.04.79
- Jiao Y, Li X, Zhang X, et al. Silver antibacterial surface adjusted by hierarchical structure on 3D printed porous titanium alloy. Appl Surf Sci. 2023;610:155519. doi: 10.1016/j.apsusc.2022.155519
- Vignesh R, Sakthinathan G, Velusamy R, Ramakrishna S. An in-vitro evaluation study on the effects of surface modification via physical vapor deposition on the degradation rates of magnesium-based biomaterials. Surf Coat Technol. 2021;411:126972. doi: 10.1016/j.surfcoat.2021.126972
- Diez-Escudero A, Andersson B, Carlsson E, et al. 3D-printed porous Ti6Al4V alloys with silver coating combine osteocompatibility and antimicrobial properties. Biomater Adv. 2022;133:112629. doi: 10.1016/j.msec.2021.112629
- Wang F, Wang L, Feng Y, et al. Evaluation of an artificial vertebral body fabricated by a tantalum-coated porous titanium scaffold for lumbar vertebral defect repair in rabbits. Sci Rep. 2018;8:8927. doi: 10.1038/s41598-018-27182-x
- MetalsFree Full-Text Electrochemical Surface Treatment of a β-titanium Alloy to Realize an Antibacterial Property and Bioactivity, (n.d.). Available from: https://www.mdpi. com/2075-4701/6/4/76 [Last accessed on 2024 Jan 13.
- Vidal E, Guillem-Marti J, Ginebra MP, Combes C, Ruperez E, Rodriguez D. Multifunctional homogeneous calcium phosphate coatings: Toward antibacterial and cell adhesive titanium scaffolds. Surf Coat Technol. 2021;405:126557. doi: 10.1016/j.surfcoat.2020.126557
- Guo Y, Ren L, Xie K, et al. Functionalized TiCu/Ti-Cu-N-coated 3D-printed porous Ti6Al4V scaffold promotes bone regeneration through BMSC recruitment. Adv Mater Interf. 2020;7:1901632. doi: 10.1002/admi.201901632
- Zhang Z, Li Y, He P, et al. Nanotube-decorated hierarchical tantalum scaffold promoted early osseointegration. Nanomedicine. 2021;35:102390. doi: 10.1016/j.nano.2021.102390
- Shokuhfar T, Hamlekhan A, Chang JY, Choi CK, Sukotjo C, Friedrich C. Biophysical evaluation of cells on nanotubular surfaces: The effects of atomic ordering and chemistry. Int J Nanomedicine. 2014;9:3737-3748. doi: 10.2147/IJN.S67344
- Makurat-Kasprolewicz B, Ossowska A. Recent advances in electrochemically surface treated titanium and its alloys for biomedical applications: A review of anodic and plasma electrolytic oxidation methods. Mater Today Commun. 2023;34:105425. doi: 10.1016/j.mtcomm.2023.105425
- Liang CY, Jiang XJ, Ji RL, et al. Preparation and surface modification of 3D printed Ti–6Al–4V porous implant. Rare Met. 2021;40:1164-1172. doi: 10.1007/s12598-020-01413-5
- Li G, Ma F, Liu P, et al. Review of micro-arc oxidation of titanium alloys: Mechanism, properties and applications. J Alloys Compd. 2023;948:169773. doi: 10.1016/j.jallcom.2023.169773
- Ming X, Wu Y, Zhang Z, Li Y. Micro-arc oxidation in titanium and its alloys: Development and potential of implants. Coatings. 2023;13:2064. doi: 10.3390/coatings13122064
- Wen X, Liu Y, Xi F, Zhang X, Kang Y. Micro-arc oxidation (MAO) and its potential for improving the performance of titanium implants in biomedical applications. Front Bioeng Biotechnol. 2023;11:1282590. doi: 10.3389/fbioe.2023.1282590
- Yan Y, Sun J, Han H, Li D, Cui K. Microstructure and bioactivity of Ca, P and Sr doped TiO2 coating formed on porous titanium by micro-arc oxidation. Surf Coat Technol. 2010;205:1702-1713. doi: 10.1016/j.surfcoat.2010.09.040
- Sun X, Tong S, Yang S, Guo S. The effects of graphene on the biocompatibility of a 3D-printed porous titanium alloy. Coatings. 2021;11:1509. doi: 10.3390/coatings11121509
- Huang H, Wu Z, Yang Z, et al. In vitro application of drug-loaded hydrogel combined with 3D-printed porous scaffolds. Biomed Mater. 2022;17:065019. doi: 10.1088/1748-605X/ac9943
- Chen H, Feng R, Xia T, et al. Progress in surface modification of titanium implants by hydrogel coatings. Gels. 2023;9:423. doi: 10.3390/gels9050423
- Bai H, Cui Y, Wang C, et al. 3D printed porous biomimetic composition sustained release zoledronate to promote osteointegration of osteoporotic defects. Mater Des. 2020;189:108513. doi: 10.1016/j.matdes.2020.108513
- Yu L, Wu Y, Liu J, et al. 3D Culture of bone marrow-derived mesenchymal stem cells (BMSCs) could improve bone regeneration in 3D-printed porous Ti6Al4V scaffolds. Stem Cells Int. 2018;2018:2074021. doi: 10.1155/2018/2074021
- Qiao S, Sheng Q, Li Z, et al. 3D-printed Ti6Al4V scaffolds coated with freeze-dried platelet-rich plasma as bioactive interface for enhancing osseointegration in osteoporosis. Mater Des. 2020;194:108825. doi: 10.1016/j.matdes.2020.108825
- Kumar A, Nune KC, Misra RDK. Biological functionality and mechanistic contribution of extracellular matrix‐ornamented three dimensional Ti‐6Al‐4V mesh scaffolds. J Biomed Mater Res. 2016;104:2751-2763. doi: 10.1002/jbm.a.35809
- Croes M, Bakhshandeh S, Van Hengel IAJ, et al. Antibacterial and immunogenic behavior of silver coatings on additively manufactured porous titanium. Acta Biomater. 2018;81:315-327. doi: 10.1016/j.actbio.2018.09.051
- Wei X, Chen Q, Bu L, et al. Improved muscle regeneration into a joint prosthesis with mechano-growth factor loaded within mesoporous silica combined with carbon nanotubes on a porous titanium alloy. ACS Nano. 2022;16:14344-14361. doi: 10.1021/acsnano.2c04591
- Jiang P, Zhang Y, Shi B, et al. Advanced surface engineering of titanium materials for biomedical applications: From static modification to dynamic responsive regulation. Bioact Mater. 2023;27:15-57. doi: 10.1016/j.bioactmat.2023.03.006
- Wu H, Dong H, Tang Z, et al. Electrical stimulation of piezoelectric BaTiO3 coated Ti6Al4V scaffolds promotes anti-inflammatory polarization of macrophages and bone repair via MAPK/JNK inhibition and OXPHOS activation. Biomaterials. 2023;293:121990. doi: 10.1016/j.biomaterials.2022.121990
- Lascano S, Chávez-Vásconez R, Muñoz-Rojas D, et al. Graphene-coated Ti-Nb-Ta-Mn foams: A promising approach towards a suitable biomaterial for bone replacement. Surf Coat Technol. 2020;401:126250. doi: 10.1016/j.surfcoat.2020.126250