Intratumoral Bacillus Calmette–Guérin as a dual immunometabolic therapy for triple-negative breast cancer: A comprehensive narrative review of mechanistic insights and translational potential
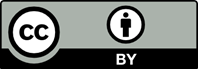
Triple-negative breast cancer (TNBC) is an aggressive and metabolically distinct subtype of breast cancer characterized by immune evasion, a high reliance on glycolysis, and poor treatment outcomes. Given the limitations of conventional therapies, there is an urgent need for novel, targeted approaches that integrate immune stimulation with metabolic disruption. This review explores the potential of intratumoral Bacillus Calmette–Guérin (BCG) therapy as a dual-action strategy in TNBC, focusing on its ability to convert immunologically “cold” tumors into “hot” tumors when simultaneously targeting TNBC’s metabolic vulnerabilities. A comprehensive narrative review was conducted using PubMed, Scopus, and Web of Science, identifying 60 peer-reviewed studies published between 2000 and 2024. The selection criteria focused on BCG’s role in oncology, its immunological and metabolic effects, and its application in solid tumors. Studies were assessed for methodological rigor using the Scale for the Assessment of Narrative Review Articles checklist. BCG enhances antitumor immunity by engaging Toll-like receptors, triggering proinflammatory cytokine release (e.g., tumor necrosis factor alpha, interferon gamma, and interleukin-12), and promoting the infiltration of tumor-infiltrating lymphocytes, including cytotoxic T-cells and natural killer cells. This immune activation reprograms the tumor microenvironment, increasing susceptibility to immunotherapy. Simultaneously, BCG disrupts TNBC’s glycolytic dependence by downregulating hexokinase 2 and pyruvate kinase M2, forcing a metabolic shift toward oxidative phosphorylation. This metabolic stress induces mitochondrial dysfunction, reactive oxygen species accumulation, and tumor cell apoptosis. In addition, BCG-induced “trained immunity” epigenetically reprograms innate immune cells, enhancing long-term tumor surveillance and reducing recurrence risk. Intratumoral BCG presents a promising immunometabolic intervention for TNBC by simultaneously enhancing immune activation and disrupting tumor metabolism. Future studies should focus on optimizing its clinical application, developing sustained-release formulations, and exploring synergistic combinations with immune checkpoint inhibitors and metabolic inhibitors. By addressing TNBC’s dual vulnerabilities, this strategy may redefine treatment paradigms and improve patient outcomes.
- Chen JQ, Russo J. ERalpha-negative and triple negative breast cancer: Molecular features and potential therapeutic approaches. Biochim Biophys Acta. 2009;1796(2):162-175. doi: 10.1016/j.bbcan.2009.06.003
- Aysola K, Desai A, Welch C, et al. Triple negative breast cancer - an overview. Hereditary Genet. 2013;2013(Suppl 2):001. doi: 10.4172/2161-1041.S2-001
- Cardillo F, Bonfim M, Vasconcelos Sousa PDS, et al. Bacillus Calmette-Guérin Immunotherapy for cancer. Vaccines (Basel). 2021;9(5):439. doi: 10.3390/vaccines9050439
- Zhang W, Yu L, Chang Z, Xiong H. BCG immunotherapy promotes tumor-derived T-cell activation through the FLT3/FLT3LG pathway in bladder cancer. J Cancer. 2024;15(3):623-631. doi: 10.7150/jca.90085
- Suttmann H, Jacobsen M, Reiss K, Jocham D, Böhle A, Brandau S. Mechanisms of bacillus Calmette-Guerin mediated natural killer cell activation. J Urol. 2004;172(4 Pt 1):1490-1495. doi: 10.1097/01.ju.0000131944.52354.63
- Schiliro C, Firestein BL. Mechanisms of metabolic reprogramming in cancer cells supporting enhanced growth and proliferation. Cells. 2021;10(5):1056. doi: 10.3390/cells10051056
- Wang Z, Jiang Q, Dong C. Metabolic reprogramming in triple-negative breast cancer. Cancer Biol Med. 2020;17(1):44-59. doi: 10.20892/j.issn.2095-3941.2019.0210
- Chen J, Gao L, Wu X, et al. BCG-induced trained immunity: History, mechanisms and potential applications. J Transl Med. 2023;21(1):106. doi: 10.1186/s12967-023-03944-8
- Yao H, He G, Yan S, et al. Triple-negative breast cancer: Is there a treatment on the horizon? Oncotarget. 2017;8(1):1913-1924. doi: 10.18632/oncotarget.12284
- Derakhshan F, Reis-Filho JS. Pathogenesis of triple-negative breast cancer. Ann Rev Pathol. 2022;17:181-204. doi: 10.1146/annurev-pathol-042420-093238
- Eustace AJ, Lee MJ, Colley G, Roban J, Downing T, Buchanan PJ. Aberrant calcium signalling downstream of mutations in TP53 and the PI3K/AKT pathway genes promotes disease progression and therapy resistance in triple negative breast cancer. Cancer Drug Resistance (Alhambra, Calif). 2022;5(3):560-576. doi: 10.20517/cdr.2022.41
- Sun X, Wang M, Wang M, et al. Metabolic reprogramming in triple-negative breast cancer. Front Oncol. 2020;10:428. doi: 10.3389/fonc.2020.00428
- Zhu Y, Li X, Wang L, Hong X, Yang J. Metabolic reprogramming and crosstalk of cancer-related fibroblasts and immune cells in the tumor microenvironment. Front Endocrinol (Lausanne). 2022;13:988295. doi: 10.3389/fendo.2022.988295
- Li JJ, Tsang JY, Tse GM. Tumor microenvironment in breast cancer-updates on therapeutic implications and pathologic assessment. Cancers (Basel). 2021;13(16):4233. doi: 10.3390/cancers13164233
- Chen X, Feng L, Huang Y, Wu Y, Xie N. Mechanisms and strategies to overcome PD-1/PD-L1 blockade resistance in triple-negative breast cancer. Cancers (Basel). 2022;15(1):104. doi: 10.3390/cancers15010104
- Choi S, Lee YR, Kim KM, Choi E, Jeon BH. Dual function of secreted APE1/Ref-1 in TNBC tumorigenesis: An apoptotic initiator and a regulator of chronic inflammatory signaling. Int J Mol Sci. 2022;23(16):9021. doi: 10.3390/ijms23169021
- Liu H, Wang Z, Zhou Y, Yang Y. MDSCs in breast cancer: An important enabler of tumor progression and an emerging therapeutic target. Front Immunol. 2023;14:1199273. doi: 10.3389/fimmu.2023.1199273
- Guo S, Huang J, Li G, Chen W, Li Z, Lei J. The role of extracellular vesicles in circulating tumor cell-mediated distant metastasis. Mol Cancer. 2023;22(1):193. doi: 10.1186/s12943-023-01909-5
- Li Y, Zheng Y, Tan X, Du Y, Wei Y, Liu S. Extracellular vesicle-mediated pre-metastatic niche formation via altering host microenvironments. Front Immunol. 2024;15:1367373. doi: 10.3389/fimmu.2024.1367373
- Li R, Cao L. The role of tumor-infiltrating lymphocytes in triple-negative breast cancer and the research progress of adoptive cell therapy. Front Immunol. 2023;14:1194020. doi: 10.3389/fimmu.2023.1194020
- Li F, Li C, Cai X, et al. The association between CD8+ tumor-infiltrating lymphocytes and the clinical outcome of cancer immunotherapy: A systematic review and meta-analysis. EClinicalMedicine. 2021;41:101134. doi: 10.1016/j.eclinm.2021.101134
- Matuszczak M, Kiljańczyk A, Marciniak W, et al. Antioxidant properties of zinc and copper-blood zinc-to copper-ratio as a marker of cancer risk brcA1 mutation carriers. Antioxidants (Basel). 2024;13(7):841. doi: 10.3390/antiox13070841
- Li J, Lu J, Wang G, Zhao A, Xu M. Past, Present and future of Bacillus Calmette-Guérin vaccine use in China. Vaccines. 2022;10(7):1157. doi: 10.3390/vaccines10071157
- Pooransingh S, Sakhamuri S. Need for BCG vaccination to prevent TB in high-incidence countries and populations. Emerg Infect Dis. 2020;26(3):624-625. doi: 10.3201/eid2603.191232
- Guallar-Garrido S, Julián E. Bacillus Calmette-Guérin (BCG) therapy for bladder cancer: An update. Immunotargets Therapy. 2020;9:1-11. doi: 10.2147/ITT.S202006
- Claps F, Pavan N, Ongaro L, et al. BCG-unresponsive non-muscle-invasive bladder cancer: Current treatment landscape and novel emerging molecular targets. Int J Mol Sci. 2023;24(16):12596. doi: 10.3390/ijms241612596
- Covián C, Fernández-Fierro A, Retamal-Díaz A, et al. BCG-induced cross-protection and development of trained immunity: Implication for vaccine design. Front Immunol. 2019;10:2806. doi: 10.3389/fimmu.2019.02806
- Wicherska-Pawłowska K, Wróbel T, Rybka J. Toll-Like receptors (TLRs), NOD-Like receptors (NLRs), and RIG-I-like receptors (RLRs) in innate immunity. TLRs, NLRs, and RLRs ligands as immunotherapeutic agents for hematopoietic diseases. Int J Mol Sci. 2021;22(24):13397. doi: 10.3390/ijms222413397
- Foster M, Hill PC, Setiabudiawan TP, Koeken VACM, Alisjahbana B, Van Crevel R. BCG-induced protection against Mycobacterium tuberculosis infection: Evidence, mechanisms, and implications for next-generation vaccines. Immunol Rev. 2021;301(1):122-144. doi: 10.1111/imr.12965
- Singh S, Saavedra-Avila NA, Tiwari S, Porcelli SA. A century of BCG vaccination: Immune mechanisms, animal models, non-traditional routes and implications for COVID-19. Front Immunol. 2022;13:959656. doi: 10.3389/fimmu.2022.959656
- Ochando J, Mulder WJM, Madsen JC, Netea MG, Duivenvoorden R. Trained immunity - basic concepts and contributions to immunopathology. Nat Rev Nephrol. 2023;19(1):23-37. doi: 10.1038/s41581-022-00633-5
- Jiang S, Redelman-Sidi G. BCG in bladder cancer immunotherapy. Cancers (Basel). 2022;14:3073. doi: 10.3390/cancers14133073
- Chelakkot C, Chelakkot VS, Shin Y, Song K. Modulating glycolysis to improve cancer therapy. Int J Mol Sci. 2023;24(3):2606. doi: 10.3390/ijms24032606
- Arts RJW, Carvalho A, La Rocca C, et al. Immunometabolic pathways in BCG-induced trained immunity. Cell Rep. 2016;17(10):2562-2571. doi: 10.1016/j.celrep.2016.11.011
- Mogensen TH. Pathogen recognition and inflammatory signaling in innate immune defenses. Clin Microbiol Rev. 2009;22(2):240-273. doi: 10.1128/CMR.00046-08
- Moorlag SJC, Rodriguez-Rosales YA, Gillard J, et al. BCG vaccination induces long-term functional reprogramming of human neutrophils. Cell Rep. 2020;33(7):108387. doi: 10.1016/j.celrep.2020.108387
- Ramírez-Labrada A, Pesini C, Santiago L, et al. All about (NK Cell-Mediated) death in two acts and an unexpected encore: Initiation, execution and activation of adaptive immunity. Front Immunol. 2022;13:896228. doi: 10.3389/fimmu.2022.896228
- Harding CV, Boom WH. Regulation of antigen presentation by Mycobacterium tuberculosis: A role for Toll-like receptors. Nat Rev Microbiol. 2010;8(4):296-307. doi: 10.1038/nrmicro2321
- Liu S, Li Y, Yuan M, Song Q, Liu M. Correlation between the Warburg effect and progression of triple-negative breast cancer. Front Oncol. 2023;12:1060495. doi: 10.3389/fonc.2022.1060495
- Kühtreiber WM, Takahashi H, Keefe RC, et al. BCG vaccinations upregulate myc, a central switch for improved glucose metabolism in diabetes. iScience. 2020;23(5):101085. doi: 10.1016/j.isci.2020.101085
- Puckett DL, Alquraishi M, Chowanadisai W, Bettaieb A. The role of PKM2 in metabolic reprogramming: Insights into the regulatory roles of non-coding RNAs. Int J Mol Sci. 2021;22(3):1171. doi: 10.3390/ijms22031171
- Nayak AP, Kapur A, Barroilhet L, Patankar MS. Oxidative phosphorylation: A target for novel therapeutic strategies against ovarian cancer. Cancers. 2018;10(9):337. doi: 10.3390/cancers10090337
- Kuo CL, Ponneri Babuharisankar A, Lin YC, et al. Mitochondrial oxidative stress in the tumor microenvironment and cancer immunoescape: Foe or friend? J Biomed Sci. 2022;29(1):74. doi: 10.1186/s12929-022-00859-2
- Madura Larsen J, Benn CS, Fillie Y, et al. BCG stimulated dendritic cells induce an interleukin-10 producing T-cell population with no T helper 1 or T helper 2 bias in vitro. Immunology. 2007;121(2):276-282. doi: 10.1111/j.1365-2567.2007.02575.x
- Zhou D, Duan Z, Li Z, Ge F, Wei R, Kong L. The significance of glycolysis in tumor progression and its relationship with the tumor microenvironment. Front Pharmacol. 2022;13:1091779. doi: 10.3389/fphar.2022.1091779
- Liu Q, Liu Z, Zhang X, Zeng A, Song L. Revisiting of cancer immunotherapy: Insight from the dialogue between glycolysis and PD-1/PD-L1 axis in the tumor microenvironment. Int J Biol Sci. 2025;21(3):1202-1221. doi: 10.7150/ijbs.104079
- Ambe RC, Bhalla S, Alvarado A, Barragan J, Cervantes J. Bacille-Calmette-Guerin modulates human macrophage and dendritic cell response to SARS-CoV-2 S-glycoprotein. Infect Med (Beijing). 2023;2(3):241-245. doi: 10.1016/j.imj.2023.08.004
- Akl MM, Ahmed A. Exploring the interplay between the warburg effect and glucolipotoxicity in cancer development: A novel perspective on cancer etiology. Adv Pharm Bull. 2024;14(3):705-713. doi: 10.34172/apb.2024.049
- Cheng S, Li Y, Sun X, et al. The impact of glucose metabolism on inflammatory processes in sepsis-induced acute lung injury. Front Immunol. 2024;15:1508985. doi: 10.3389/fimmu.2024.1508985
- Drouillard D, Craig BT, Dwinell MB. Physiology of chemokines in the cancer microenvironment. Am J Physiol Cell Physiol. 2023;324(1):C167-C182. doi: 10.1152/ajpcell.00151.2022
- Hu A, Sun L, Lin H, et al. Harnessing innate immune pathways for therapeutic advancement in cancer. Sig Transduct Target Ther. 2024;9:68. doi: 10.1038/s41392-024-01765-9
- Chen Z, Han F, Du Y, et al. Hypoxic microenvironment in cancer: Molecular mechanisms and therapeutic interventions. Sig Transduct Target Ther. 2023;8:70. doi: 10.1038/s41392-023-01332-8
- Karasarides M, Cogdill AP, Robbins PB, et al. Hallmarks of resistance to immune-checkpoint inhibitors. Cancer Immunol Res. 2022;10(4):372-383 doi: 10.1158/2326-6066.CIR-20-0586
- Briukhovetska D, Dörr J, Endres S, et al. Interleukins in cancer: From biology to therapy. Nat Rev Cancer. 2021;21:481-499. doi: 10.1038/s41568-021-00363-z
- Matsumoto H, Koo SL, Dent R, Tan PH, Iqbal J. Role of inflammatory infiltrates in triple negative breast cancer. J Clin Pathol. 2015;68(7):506-510. doi: 10.1136/jclinpath-2015-202944
- Huang R, Kang T, Chen S. The role of tumor-associated macrophages in tumor immune evasion. J Cancer Res Clin Oncol. 2024;150(5):238. doi: 10.1007/s00432-024-05777-4