Use of melatonin for the treatment of dementia: Addressing core symptoms and behavioral challenges
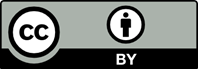
Dementia mainly includes Alzheimer’s disease (AD), dementia with Lewy bodies (DLB), frontotemporal dementia (FTD), and vascular dementia (VaD). In addition to cognitive impairment, patients often suffer from behavioral and psychological symptoms of dementia (BPSD). Melatonin administration has emerged as a promising therapeutic strategy with potential benefits for BPSD. This review focuses on the therapeutic effects of melatonin on the core symptoms of dementia and BPSD. Specifically, the impact of melatonin on memory impairment, sleep disturbances, anxiety, depression, delusions, and hallucinations is reviewed. The melatonin’s role in amyloid-β, synuclein aggregation, reactive oxygen species, and dementia subtypes are discussed. We aim to provide an up-to-date review of the emerging application of melatonin; thus, dementia patient care and social welfare can be improved by the appropriate usage of this medication.
- Karran E, Mercken M, de Strooper B, 2011, The amyloid cascade hypothesis for Alzheimer’s disease: An appraisal for the development of therapeutics. Nat Rev Drug Discov, 10: 698–712. https://doi.org/10.1038/nrd3505
- Pinheiro L, Faustino C, 2019, Therapeutic strategies targeting amyloid-β in Alzheimer’s disease. Curr Alzheimer Res, 16: 418–452. https://doi.org/10.2174/1567205016666190321163438
- Meyer JS, Rauch G, Rauch RA, et al., 2000, Risk factors for cerebral hypoperfusion, mild cognitive impairment, and dementia. Neurobiol Aging, 21: 161–169. https://doi.org/10.1016/s0197-4580(00)00136-6
- Coca A, 2013, Hypertension and vascular dementia in the elderly: The potential role of anti-hypertensive agents. Curr Med Res Opin, 29: 1045–1054. https://doi.org/10.1185/03007995.2013.813841
- Pantoni L, 2004, Treatment of vascular dementia: Evidence from trials with non-cholinergic drugs. J Neurol Sci, 226: 67–70. https://doi.org/10.1016/j.jns.2004.09.014
- Taylor JP, McKeith IG, Burn DJ, et al., 2020, New evidence on the management of Lewy body dementia. Lancet Neurol, 19: 157–169. https://doi.org/10.1016/S1474-4422(19)30153-X
- Olney NT, Spina S, Miller BL, 2017, Frontotemporal dementia. Neurol Clin, 35: 339–374. https://doi.org/10.1016/j.ncl.2017.01.008
- Schmidt MF, Gan ZY, Komander D, et al., 2021, Ubiquitin signalling in neurodegeneration: Mechanisms and therapeutic opportunities. Cell Death Differ, 28: 570–590. https://doi.org/10.1038/s41418-020-00706-7
- Shi L, Chen SJ, Ma MY, et al., 2018, Sleep disturbances increase the risk of dementia: A systematic review and meta-analysis. Sleep Med Rev, 40: 4–16. https://doi.org/10.1016/j.smrv.2017.06.010
- Fan R, Peng X, Xie L, et al., 2022, Importance of Bmal1 in Alzheimer’s disease and associated aging-related diseases: Mechanisms and interventions. Aging Cell, 21: e13704. https://doi.org/10.1111/acel.13704
- Reppert SM, Godson C, Mahle CD, et al., 1995, Molecular characterization of a second melatonin receptor expressed in human retina and brain: The Mel1b melatonin receptor. Proc Natl Acad Sci U S A, 92: 8734–8738. https://doi.org/10.1073/pnas.92.19.8734
- Pandi-Perumal SR, Trakht I, Srinivasan V, et al., 2008, Physiological effects of melatonin: Role of melatonin receptors and signal transduction pathways. Prog Neurobiol, 85: 335–353. https://doi.org/10.1016/j.pneurobio.2008.04.001
- Alghamdi BS, 2018, The neuroprotective role of melatonin in neurological disorders. J Neurosci Res, 96: 1136–1149. https://doi.org/10.1002/jnr.24220
- Kwon KJ, Kim HJ, Shin CY, et al., 2010, Melatonin potentiates the neuroprotective properties of resveratrol against beta-amyloid-induced neurodegeneration by modulating AMP-activated protein kinase pathways. J Clin Neurol, 6: 127–137. https://doi.org/10.3988/jcn.2010.6.3.127
- Bonnefont-Rousselot D, Collin F, 2010, Melatonin: Action as antioxidant and potential applications in human disease and aging. Toxicology, 278: 55–67. https://doi.org/10.1016/j.tox.2010.04.008
- Das A, McDowell M, Pava MJ, et al., 2010, The inhibition of apoptosis by melatonin in VSC4.1 motoneurons exposed to oxidative stress, glutamate excitotoxicity, or TNF-alpha toxicity involves membrane melatonin receptors. J Pineal Res, 48: 157–169. https://doi.org/10.1111/j.1600-079X.2009.00739.x
- Esposito E, Cuzzocrea S, 2010, Antiinflammatory activity of melatonin in central nervous system. Curr Neuropharmacol, 8: 228–242. https://doi.org/10.2174/157015910792246155
- Zhang HM, Zhang Y, 2014, Melatonin: A well-documented antioxidant with conditional pro-oxidant actions. J Pineal Res, 57: 131–146. https://doi.org/10.1111/jpi.12162
- Feng Z, Chang Y, Cheng Y, et al., 2004, Melatonin alleviates behavioral deficits associated with apoptosis and cholinergic system dysfunction in the APP 695 transgenic mouse model of Alzheimer’s disease. J Pineal Res, 37: 129–136. https://doi.org/10.1111/j.1600-079X.2004.00144.x
- Iwashita H, Matsumoto Y, Maruyama Y, et al., 2021, The melatonin metabolite N1-acetyl-5-methoxykynuramine facilitates long-term object memory in young and aging mice. J Pineal Res, 70: e12703. https://doi.org/10.1111/jpi.12703
- Sano M, Iwashita H, Hattori A, et al., 2022, Melatonin enhances object recognition memory through melatonin MT1 and MT2 receptor-mediated and non-receptor-mediated mechanisms in male mice. J Behav Brain Sci, 12: 640–657. https://doi.org/10.4236/jbbs.2022.1212038
- Galano A, Tan DX, Reiter RJ, 2013, On the free radical scavenging activities of melatonin’s metabolites, AFMK and AMK. J Pineal Res, 54: 245–257. https://doi.org/10.1111/jpi.12010
- Rudnitskaya EA, Maksimova KY, Muraleva NA, et al., 2015, Beneficial effects of melatonin in a rat model of sporadic Alzheimer’s disease. Biogerontology, 16: 303–316. https://doi.org/10.1007/s10522-014-9547-7
- Gong YH, Hua N, Zang X, et al., 2018, Melatonin ameliorates aβ1-42 -induced Alzheimer’s cognitive deficits in mouse model. J Pharm Pharmacol, 70: 70–80. https://doi.org/10.1111/jphp.12830
- Muhammad T, Ali T, Ikram M, et al., 2019, Melatonin rescue oxidative stress-mediated neuroinflammation/ neurodegeneration and memory impairment in scopolamine-induced amnesia mice model. J Neuroimmune Pharmacol, 14: 278–294. https://doi.org/10.1007/s11481-018-9824-3
- Barchas J, DaCosta F, Spector S, 1967, Acute pharmacology of melatonin. Nature, 214: 919–920. https://doi.org/10.1038/214919a0
- Nickkholgh A, Schneider H, Sobirey M, et al., 2011, The use of high-dose melatonin in liver resection is safe: First clinical experience. J Pineal Res, 50: 381–388. https://doi.org/10.1111/j.1600-079X.2011.00854.x
- Zisapel N, 2023, Melatonin-dopamine interactions: From basic neurochemistry to a clinical setting. Cell Mol Neurobiol, 21: 605–616. https://doi.org/10.1023/a:1015187601628
- Leeboonngam T, Pramong R, Sae-Ung K, et al., 2018, Neuroprotective effects of melatonin on amphetamine-induced dopaminergic fiber degeneration in the hippocampus of postnatal rats. J Pineal Res, 64. https://doi.org/10.1111/jpi.12456
- Iuvone PM, Gan J, 1995, Functional interaction of melatonin receptors and D1 dopamine receptors in cultured chick retinal neurons. J Neurosci, 15: 2179–2185. https://doi.org/10.1523/JNEUROSCI.15-03-02179.1995
- Shibata ACE, Ueda HH, Eto K, et al., 2021, Photoactivatable CaMKII induces synaptic plasticity in single synapses. Nat Commun, 12: 751. https://doi.org/10.1038/s41467-021-21025-6
- Xu J, Kawahata I, Izumi H, et al., 2021, T-type ca2+ enhancer SAK3 activates CaMKII and proteasome activities in Lewy body dementia mice model. Int J Mol Sci, 22: 6185. https://doi.org/10.3390/ijms22126185
- Jarome TJ, Ferrara NC, Kwapis JL, et al., 2016, CaMKII regulates proteasome phosphorylation and activity and promotes memory destabilization following retrieval. Neurobiol Learn Mem, 128: 103–109. https://doi.org/10.1016/j.nlm.2016.01.001
- Benítez-King G, Ríos A, Martínez A, et al., 1996, In vitro inhibition of ca2+/calmodulin-dependent kinase II activity by melatonin. Biochim Biophys Acta, 1290: 191–196. https://doi.org/10.1016/0304-4165(96)00025-6
- León J, Escames G, Rodríguez MI, et al., 2006, Inhibition of neuronal nitric oxide synthase activity by N1-acetyl- 5-methoxykynuramine, a brain metabolite of melatonin. J Neurochem, 98: 2023–2033. https://doi.org/10.1111/j.1471-4159.2006.04029.x
- Argueta J, Solís-Chagoyán H, Estrada-Reyes R, et al., 2022, Further evidence of the melatonin calmodulin interaction: Effect on CaMKII activity. Int J Mol Sci, 23: 2479. https://doi.org/10.3390/ijms23052479
- Jiang H, Ashraf GM, Liu M, et al., 2021, Tilianin ameliorates cognitive dysfunction and neuronal damage in rats with vascular dementia via p-CaMKII/ERK/CREB and ox-CaMKII-dependent MAPK/NF- κ B pathways. Oxid Med Cell Longev, 2021: 6673967. https://doi.org/10.1155/2021/6673967
- Chang CF, Huang HJ, Lee HC, et al., 2012, Melatonin attenuates kainic acid-induced neurotoxicity in mouse hippocampus via inhibition of autophagy and α-synuclein aggregation. J Pineal Res, 52: 312–321. https://doi.org/10.1111/j.1600-079X.2011.00945.x
- Sutcu R, Yonden Z, Yilmaz A, et al., 2006, Melatonin increases NMDA receptor subunits 2A and 2B concentrations in rat hippocampus. Mol Cell Biochem, 283: 101–105. https://doi.org/10.1007/s11010-006-2385-4
- Furuta T, Nakagawa I, Yokoyama S, et al., 2022, Melatonin-induced postconditioning suppresses NMDA receptor through opening of the mitochondrial permeability transition pore via melatonin receptor in mouse neurons. Int J Mol Sci, 23: 3822. https://doi.org/10.3390/ijms23073822
- Liu J, Chang L, Song Y, Li H, Wu Y. The role of NMDA receptors in alzheimer’s disease. Front Neurosci, 2019; 13:43. https://doi.org/10.3389/fnins.2019.00043
- Albert-Gascó H, Ros-Bernal F, Castillo-Gómez E, et al., 2020, MAP/ERK signaling in developing cognitive and emotional function and its effect on pathological and neurodegenerative processes. Int J Mol Sci, 21: 4471. https://doi.org/10.3390/ijms21124471
- Liu SC, Tsai CH, Wang YH, et al., 2022, Melatonin abolished proinflammatory factor expression and antagonized osteoarthritis progression in vivo. Cell Death Dis, 13: 215. https://doi.org/10.1038/s41419-022-04656-5
- Shin EJ, Chung YH, Le HLT, et al., 2014, Melatonin attenuates memory impairment induced by Klotho gene deficiency via interactive signaling between MT2 receptor, ERK, and Nrf2- related antioxidant potential. Int J Neuropsychopharmacol, 18: pyu105. https://doi.org/10.1093/ijnp/pyu105
- Iwashita H, Sano M, Kawaguchi M, et al., 2023, N-acetyl-5- methoxykynuramine enhance object location and working memory performances via modulating CaMKII, ERK and CREB phosphorylation. Neuroreport, 34: 299–307. https://doi.org/10.1097/WNR.0000000000001893
- Salminen A, 2022, Role of indoleamine 2,3-dioxygenase 1 (IDo1) and kynurenine pathway in the regulation of the aging process. Ageing Res Rev, 75: 101573. https://doi.org/10.1016/j.arr.2022.101573
- de Oliveira Silva S, Ximenes VF, Livramento JA, et al., 2005, High concentrations of the melatonin metabolite, N1-acetyl- N2-formyl-5-methoxykynuramine, in cerebrospinal fluid of patients with meningitis: A possible immunomodulatory mechanism. J Pineal Res, 39: 302–306. https://doi.org/10.1111/j.1600-079X.2005.00247.x
- Xie Z, Chen F, Li WA, et al., 2017, A review of sleep disorders and melatonin. Neurol Res, 39: 559–565. https://doi.org/10.1080/01616412.2017.1315864
- Reiter RJ, Sharma R, Cucielo MS, et al., 2023, Brain washing and neural health: Role of age, sleep, and the cerebrospinal fluid melatonin rhythm. Cell Mol Life Sci, 80: 88. https://doi.org/10.1007/s00018-023-04736-5
- Cui SY, Li SJ, Cui XY, et al., 2016, Phosphorylation of CaMKII in the rat dorsal raphe nucleus plays an important role in sleep-wake regulation. J Neurochem, 136: 609–619. https://doi.org/10.1111/jnc.13431
- Mikhail C, Vaucher A, Jimenez S, et al., 2017, ERK signaling pathway regulates sleep duration through activity-induced gene expression during wakefulness. Sci Signal, 10: eaai9219. https://doi.org/10.1126/scisignal.aai9219
- Nie L, Wei G, Peng S, et al., 2017, Melatonin ameliorates anxiety and depression-like behaviors and modulates proteomic changes in triple transgenic mice of Alzheimer’s disease. Biofactors, 43: 593–611. https://doi.org/10.1002/biof.1369
- Boiko DI, Shkodina AD, Hasan MM, et al., 2022, Melatonergic receptors (MT1/MT2) as a potential additional target of novel drugs for depression. Neurochem Res, 47: 2909–2924. https://doi.org/10.1007/s11064-022-03646-5
- Binfaré RW, Mantovani M, Budni J, et al., 2010, Involvement of dopamine receptors in the antidepressant-like effect of melatonin in the tail suspension test. Eur J Pharmacol, 638: 78–83. https://doi.org/10.1016/j.ejphar.2010.04.011
- Xu Z, Li W, Sun Y, et al., 2023, Melatonin alleviates PTSD-like behaviors and restores serum GABA and cortisol levels in mice. Psychopharmacology (Baerl), 240: 259–269. https://doi.org/10.1007/s00213-023-06312-y
- Yabuki Y, Takahata I, Matsuo K, et al., 2018, Ramelteon improves post-traumatic stress disorder-like behaviors exhibited by fatty acid-binding protein 3 null mice. Mol Neurobiol, 55: 3577–3591. https://doi.org/10.1007/s12035-017-0587-2
- Shioda N, Yamamoto Y, Watanabe M, et al., 2010, Heart-type fatty acid binding protein regulates dopamine D2 receptor function in mouse brain. J Neurosci, 30: 3146–3155. https://doi.org/10.1523/JNEUROSCI.4140-09.2010
- Hamdi A, 1998, Melatonin administration increases the affinity of D2 dopamine receptors in the rat striatum. Life Sci, 63: 2115–2120. https://doi.org/10.1016/s0024-3205(99)80008-3
- Zhang S, Xie C, Wang Q, et al., 2014, Interactions of CaMKII with dopamine D2 receptors: Roles in levodopa-induced dyskinesia in 6-hydroxydopamine lesioned Parkinson’s rats. Sci Rep, 4: 6811. https://doi.org/10.1038/srep06811
- Zhang R, Manza P, Tomasi D, et al., 2021, Dopamine D1 and D2 receptors are distinctly associated with rest-activity rhythms and drug reward. J Clin Invest, 131: e149722. https://doi.org/10.1172/JCI149722
- Murakoshi H, Shin ME, Parra-Bueno P, et al., 2017, Kinetics of endogenous CaMKII required for synaptic plasticity revealed by optogenetic kinase inhibitor. Neuron, 94: 37–47.e5. https://doi.org/10.1016/j.neuron.2017.02.036
- Panmak P, Nopparat C, Permpoonpattana K, et al., 2021, Melatonin protects against methamphetamine-induced Alzheimer’s disease-like pathological changes in rat hippocampus. Neurochem Int, 148: 105121. https://doi.org/10.1016/j.neuint.2021.105121
- Chinchalongporn V, Shukla M, Govitrapong P, 2018, Melatonin ameliorates Aβ42-induced alteration of βAPP-processing secretases via the melatonin receptor through the Pin1/GSK3β/NF-κB pathway in SH-SY5Y cells. J Pineal Res, 64: e12470. https://doi.org/10.1111/jpi.12470
- Sun C, Qiu X, Wang Y, et al., 2020, Long-term oral melatonin alleviates memory deficits, reduces amyloid-β deposition associated with downregulation of BACE1 and mitophagy in APP/PS1 transgenic mice. Neurosci Lett, 735: 135192. https://doi.org/10.1016/j.neulet.2020.135192
- Andrade MK, Souza LC, Azevedo EM, et al., 2023, Melatonin reduces β-amyloid accumulation and improves short-term memory in streptozotocin-induced sporadic Alzheimer’s disease model. IBRO Neurosci Rep, 14: 264–272. https://doi.org/10.1016/j.ibneur.2023.01.005
- Kamsrijai U, Wongchitrat P, Nopparat C, et al., 2020, Melatonin attenuates streptozotocin-induced Alzheimer-like features in hyperglycemic rats. Neurochem Int, 132: 104601. https://doi.org/10.1016/j.neuint.2019.104601
- Zhong M, Kou H, Zhao P, et al., 2021, Nasal delivery of D-penicillamine hydrogel upregulates a disintegrin and metalloprotease 10 expression via melatonin receptor 1 in Alzheimer’s disease models. Front Aging Neurosci, 13: 660249. https://doi.org/10.3389/fnagi.2021.660249
- Choi Y, Attwood SJ, Hoopes MI, et al., 2014, Melatonin directly interacts with cholesterol and alleviates cholesterol effects in dipalmitoylphosphatidylcholine monolayers. Soft Matter, 10: 206–213. https://doi.org/10.1039/c3sm52064a
- Mihardja M, Roy J, Wong KY, et al., 2020, Therapeutic potential of neurogenesis and melatonin regulation in Alzheimer’s disease. Ann N Y Acad Sci, 1478: 43–62. https://doi.org/10.1111/nyas.14436
- Shen S, Liao Q, Wong YK, et al., 2022, The role of melatonin in the treatment of Type 2 diabetes mellitus and Alzheimer’s disease. Int J Biol Sci, 18: 983–994. https://doi.org/10.7150/ijbs.66871
- Rosales-Corral SA, Lopez-Armas G, Cruz-Ramos J, et al., 2012, Alterations in lipid levels of mitochondrial membranes induced by Amyloid-β: A protective role of melatonin. Int J Alzheimers Dis, 2012: 459806. https://doi.org/10.1155/2012/459806
- O’Day DH, Eshak K, Myre MA, 2015, Calmodulin binding proteins and Alzheimer’s disease. J Alzheimers Dis, 46: 553–569. https://doi.org/10.3233/JAD-142772
- Reese LC, Taglialatela G, 2011, A role for calcineurin in Alzheimer’s disease. Curr Neuropharmacol, 9: 685–692. https://doi.org/10.2174/157015911798376316
- Premratanachai A, Suwanjang W, Govitrapong P, et al., 2020, Melatonin prevents calcineurin-activated the nuclear translocation of nuclear factor of activated T-cells in human neuroblastoma SH-SY5Y cells undergoing hydrogen peroxide-induced cell death. J Chem Neuroanat, 106: 101793. https://doi.org/10.1016/j.jchemneu.2020.101793
- Penny CJ, Gold MG, 2018, Mechanisms for localising calcineurin and CaMKII in dendritic spines. Cell Signal, 49: 46–58. https://doi.org/10.1016/j.cellsig.2018.05.010
- Kubokawa M, Nakamura K, Komagiri Y, 2011, Interaction between calcineurin and Ca/calmodulin kinase-II in modulating cellular functions. Enzyme Res, 2011: 587359. https://doi.org/10.4061/2011/587359
- Fukunaga K, Izumi H, Yabuki Y, et al., 2019, Alzheimer’s disease therapeutic candidate SAK3 is an enhancer of T-type calcium channels. J Pharmacol Sci, 139: 51–58. https://doi.org/10.1016/j.jphs.2018.11.014
- Meeks TW, Ropacki SA, Jeste DV, 2006, The neurobiology of neuropsychiatric syndromes in dementia. Curr Opin Psychiatry, 19: 581–586. https://doi.org/10.1097/01.yco.0000245746.45384.0e
- Srinivasan V, Kaur C, Pandi-Perumal S, et al., 2010, Melatonin and its agonist ramelteon in Alzheimer’s disease: Possible therapeutic value. Int J Alzheimers Dis, 2011: 741974. https://doi.org/10.4061/2011/741974
- Gehrman PR, Connor DJ, Martin JL, et al., 2009, Melatonin fails to improve sleep or agitation in double-blind randomized placebo-controlled trial of institutionalized patients with Alzheimer disease. Am J Geriatr Psychiatry, 17: 166–169. https://doi.org/10.1097/JGP.0b013e318187de18
- Savaskan E, Ayoub MA, Ravid R, et al., 2005, Reduced hippocampal MT2 melatonin receptor expression in Alzheimer’s disease. J Pineal Res, 38: 10–16. https://doi.org/10.1111/j.1600-079X.2004.00169.x
- Ono K, Mochizuki H, Ikeda T, et al., 2012, Effect of melatonin on α-synuclein self-assembly and cytotoxicity. Neurobiol Aging, 33: 2172–2185. https://doi.org/10.1016/j.neurobiolaging.2011.10.015
- Chen ST, Chuang JI, Hong MH, et al., 2002, Melatonin attenuates MPP+-induced neurodegeneration and glutathione impairment in the nigrostriatal dopaminergic pathway. J Pineal Res, 32: 262–269. https://doi.org/10.1034/j.1600-079x.2002.01871.x
- Brito-Armas JM, Baekelandt V, Castro-Hernández JR, et al., 2013, Melatonin prevents dopaminergic cell loss induced by lentiviral vectors expressing a30p mutant alpha-synuclein. Histol Histopathol, 28: 999–1006. https://doi.org/10.14670/HH-28.999
- Maurizi CP, 2000, A preliminary understanding of mania: Roles for melatonin, vasotocin and rapid-eye-movement sleep. Med Hypotheses, 54: 26–29. https://doi.org/10.1054/mehy.1998.0812
- Thangwong P, Jearjaroen P, Tocharus C, et al., 2023, Melatonin suppresses inflammation and blood-brain barrier disruption in rats with vascular dementia possibly by activating the SIRT1/PGC-1α/pparγ signaling pathway. Inflammopharmacology, 31: 1481–1493. https://doi.org/10.1007/s10787-023-01181-5
- Singh P, Gupta S, Sharma B, 2015, Melatonin receptor and KATP channel modulation in experimental vascular dementia. Physiol Behav, 142: 66–78. https://doi.org/10.1016/j.physbeh.2015.02.009
- Thangwong P, Jearjaroen P, Govitrapong P, et al., 2022, Melatonin improves cognitive function by suppressing endoplasmic reticulum stress and promoting synaptic plasticity during chronic cerebral hypoperfusion in rats. Biochem Pharmacol, 198: 114980. https://doi.org/10.1016/j.bcp.2022.114980
- Gupta S, Singh P, Sharma BM, et al., 2015, Neuroprotective effects of agomelatine and vinpocetine against chronic cerebral hypoperfusion induced vascular dementia. Curr neurovasc Res, 12: 240–252. https://doi.org/10.2174/1567202612666150603130235
- Bin-Jaliah I, Sakr HF, 2018, Melatonin ameliorates brain oxidative stress and upregulates senescence marker protein-30 and osteopontin in a rat model of vascular dementia. Physiol Int, 105: 38–52. https://doi.org/10.1556/2060.105.2018.1.1
- Chen BH, Park JH, Lee YL, et al., 2018, Melatonin improves vascular cognitive impairment induced by ischemic stroke by remyelination via activation of ERK1/2 signaling and restoration of glutamatergic synapses in the gerbil hippocampus. Biomed Pharmacother, 108: 687–697. https://doi.org/10.1016/j.biopha.2018.09.077
- Shen D, Tian X, Sang W, et al., 2016, Effect of melatonin and resveratrol against memory impairment and hippocampal damage in a rat model of vascular dementia. Neuroimmunomodulation, 23: 318–331. https://doi.org/10.1159/000454681
- Imbesi M, Uz T, Dzitoyeva S, et al., 2008, Stimulatory effects of a melatonin receptor agonist, ramelteon, on BDNF in mouse cerebellar granule cells. Neurosci Lett, 439: 34–36. https://doi.org/10.1016/j.neulet.2008.04.099
- Lauterbach EC, Kuppuswamy PS, Greenway LL, 2010, Differential pharmacological responses of catatonia-like signs in frontotemporal dementia. Neurocase, 16: 436– 450. https://doi.org/10.1080/13554791003623326
- Callegari I, Mattei C, Benassi F, et al., 2023, Agomelatine improves apathy in frontotemporal dementia. Neurodegener Dis, 16: 352–356. https://doi.org/10.1159/000445873