Optimization of alginate/gelatin/dextran-aldehyde bioink for 3D bioprinting and cell engraftment
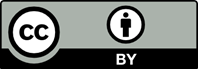
The development of bioinks with optimized printability, mechanical properties, and biocompatibility is critical for advancing three-dimensional (3D) bioprinting and tissue engineering. In this study, we introduce an alginate/gelatin/dextran-aldehyde (AGDA) bioink, designed to balance structural integrity and cellular functionality. Among the tested formulations, AGDA1 demonstrated superior performance, with optimized printability and high cell compatibility. AGDA bioinks involve dual crosslinking (ionic gelation of alginate and Schiff base formation between gelatin and dextran-aldehyde), permitting appropriate stiffness, viscosity, and thixotropic behavior. Fibroblasts encapsulated in AGDA, either as single cells, spheroids, or a combination of both, exhibited high viability and proliferative capacity. Notably, the combination method supported the highest cellular density and fibroblast-specific morphological transformations, surpassing the commercially available GelXA bioink. These findings highlight AGDA’s potential as a versatile bioink for fabricating complex and scalable tissue constructs. In summary, this study contributes to the development of bioinks tailored for enhanced cell engraftment and regenerative applications.

- Dey M, Ozbolat IT. 3D bioprinting of cells, tissues and organs. Sci Rep. 2020;10(1):14023. doi: 10.1038/s41598-020-70086-y2
- Shukla P, Yeleswarapu S, Heinrich MA, Prakash J, Pati F. Mimicking tumor microenvironment by 3D bioprinting: 3D cancer modeling. Biofabrication. 2022;14(3):032002. doi: 10.1088/1758-5090/ac6d11
- Wang Z, Xiang L, Lin F, Tang Y, Cui WJ. 3D bioprinting of emulating homeostasis regulation for regenerative medicine applications. Control Release. 2023;353:147-165. doi: 10.1016/j.jconrel.2022.11.035
- Maharjan S, Ma C, Singh B, et al. Advanced 3D imaging and organoid bioprinting for biomedical research and therapeutic applications. Adv Drug Deliv Rev. 2024;208:115237. doi: 10.1016/j.addr.2024.115237
- Zennifer A, Manivannan S, Sethuraman S, Kumbar SG, Sundaramurthi D. 3D bioprinting and photocrosslinking: emerging strategies & future perspectives. Biomater Adv. 2022;134:112576. doi: 10.1016/j.msec.2021.112576
- Zhang J, Wehrle E, Rubert M, Müller R. 3D bioprinting of human tissues: biofabrication, bioinks, and bioreactors. Int J Mol Sci. 2021;22(8):3971. doi: 10.3390/ijms22083971
- Zhang W, Kuss M, Yan Y, Shi W. Dynamic alginate hydrogel as an antioxidative bioink for bioprinting. Gels. 2023;9(4):312. doi: 10.3390/gels9040312
- Lan X, Ma Z, Szojka ARA, et al. TEMPO-oxidized cellulose nanofiber-alginate hydrogel as a bioink for human meniscus tissue engineering. Front Bioeng Biotechnol. 2021;9:766399. doi: 10.3389/fbioe.2021.766399
- Kim J, Choi YJ, Gal CW, Sung A, Park H, Yun HS. Development of an alginate-gelatin bioink enhancing osteogenic differentiation by gelatin release. Int J Bioprint. 2023;9(2):660. doi: 10.18063/ijb.v9i2.660
- Gao Q, Kim BS, Gao G. Advanced strategies for 3D bioprinting of tissue and organ analogs using alginate hydrogel bioinks. Mar Drugs. 2021;19(12):708. doi: 10.3390/md19120708
- Hao L, Zhao S, Hao S, et al. Functionalized gelatin-alginate based bioink with enhanced manufacturability and biomimicry for accelerating wound healing. Int J Biol Macromol. 2023;240:124364. doi: 10.1016/j.ijbiomac.2023.124364
- Stola GP, Paoletti C, Nicoletti L, et al. Internally-crosslinked alginate dialdehyde/alginate/gelatin-based hydrogels as bioinks for prospective cardiac tissue engineering applications. Int J Bioprint. 2024:10;4014. doi: 10.36922/ijb.4014
- Cruz EM, Machado LS, Zamproni LN, et al. A gelatin methacrylate-based hydrogel as a potential bioink for 3D bioprinting and neuronal differentiation. Pharmaceutics. 2023;15(2):627. doi: 10.3390/pharmaceutics15020627
- Shi W, Fang F, Kong Y, et al. Dynamic hyaluronic acid hydrogel with covalent linked gelatin as an anti-oxidative bioink for cartilage tissue engineering. Biofabrication. 2021;14(1). doi: 10.1088/1758-5090/ac42de
- Musilová L, Achbergerová E, Vítková L, et al. Cross-linked gelatine by modified dextran as a potential bioink prepared by a simple and non-toxic process. Polymers (Basel). 2022;14(3):391. doi: 10.3390/polym14030391
- Banigo AT, Nauta L, Zoetebier B, Karperien M. Coaxial bioprinting of enzymatically crosslinkable hyaluronic acid-tyramine bioinks for tissue regeneration. Polymers (Basel). 2024;16(17):2470. doi: 10.3390/polym16172470
- Ouyang L, Armstrong JPK, Lin Y, et al. Expanding and optimizing 3D bioprinting capabilities using complementary network bioinks. Sci Adv. 2020;6(38):eabc5529. doi: 10.1126/sciadv.abc5529
- Hauptstein J, Forster L, Nadernezhad A, et al. Bioink platform utilizing dual-stage crosslinking of hyaluronic acid tailored for chondrogenic differentiation of mesenchymal stromal cells. Macromol Biosci. 2022;22:e2100331. doi: 10.1002/mabi.202100331
- Hu T, Cui X, Zhu M, et al. 3D-printable supramolecular hydrogels with shear-thinning property: fabricating strength tunable bioink via dual crosslinking. Bioact Mater. 2020;5:808-818. doi: 10.1016/j.bioactmat.2020.06.001
- Shin JY, Yeo YH, Jeong JE, Park SA, Park WH. Dual-crosslinked methylcellulose hydrogels for 3D bioprinting applications. Carbohydr Polym. 2020;238:116192. doi: 10.1016/j.carbpol.2020.116192
- Glaeser JD, Bao X, Kaneda G, et al. iPSC-neural crest derived cells embedded in 3D printable bio-ink promote cranial bone defect repair. Sci Rep. 2022;12:18701. doi: 10.1038/s41598-022-22502-8
- Oliveros AL, Kingham PJ, Lammi MJ, Wiberg M, Kelk P. Three-dimensional osteogenic differentiation of bone marrow mesenchymal stem cells promotes matrix metallopeptidase 13 (MMP13) expression in Type I collagen hydrogels. Int J Mol Sci. 2021;22:13594. doi: 10.3390/ijms222413594
- Chang HK, Yang DH, Ha MY, et al. 3D printing of cell-laden visible light curable glycol chitosan bioink for bone tissue engineering. Carbohydr Polym. 2022;287:119328. doi: 10.1016/j.carbpol.2022.119328
- Fang Y, Ji M, Yang Y, et al. 3D printing of vascularized hepatic tissues with a high cell density and heterogeneous microenvironment. Biofabrication. 2023;15(4). doi: 10.1088/1758-5090/ace5e0
- Kripamol R, Velayudhan S, Anil Kumar PR. Evaluation of allylated gelatin as a bioink supporting spontaneous spheroid formation of HepG2 cells. Int J Biol Macromol. 2024;274(Pt 1):133259. doi: 10.1016/j.ijbiomac.2024.133259
- Lee HW, Chen KT, Li YE, Yeh YC, Chiang CY, Lee IC. Dual crosslinking silk fibroin/pectin-based bioink development and the application on neural stem/progenitor cells spheroid laden 3D bioprinting. Int J Biol Macromol. 2024; 269(Pt 2):131720. doi: 10.1016/j.ijbiomac.2024.131720
- Sun W, Zhang J, Qin Y, et al. A simple and efficient strategy for preparing a cell-spheroid-based bioink. Adv Healthc Mater. 2022;11(15):e2200648. doi: 10.1002/adhm.202200648
- Jeong JE, Han SS, Shin HE, et al. Hyaluronic microparticle-based biomimetic artificial neighbors of cells for three-dimensional cell culture. Carbohydrate Polym. 2022;294:119770. doi: 10.1016/j.carbpol.2022.119770
- Schmidt SK, Schmid R, Arkudas A, Kengelbach-Weigand A, Bosserhoff AK. Tumor cells develop defined cellular phenotypes after 3D-bioprinting in different bioinks. Cells. 2019;8:1295. doi: 10.3390/cells8101295
- Zhang Y, Ding Z, Liu Y, Zhang Y, Jiang S. White-light-emitting hydrogels with self-healing properties and adjustable emission colors. J Colloid Interface Sci. 2021;582:825-835. doi: 10.1016/j.jcis.2020.08.080
- Kang SW, Jeon O, Kim BS. Poly(lactic-co-glycolic acid) microspheres as an injectable scaffold for cartilage tissue engineering. Tissue Eng. 2005;11:438-447. doi: 10.1089/ten.2005.11.438
- Park KH, Ryu B, Song JJ, et al. Hyaluronic acid microparticles for effective spheroid culture and transplantation in liver tissue. Chem Eng J. 2023;464:142666. doi: 10.1016/j.cej.2023.142666
- Im S, Choe G, Seok JM, et al. An osteogenic bioink composed of alginate, cellulose nanofibrils, and polydopamine nanoparticles for 3D bioprinting and bone tissue engineering. Int J Biol Macromol. 2022:205:520-529. doi: 10.1016/j.ijbiomac.2022.02.012
- Rashik C, Muhire BS, Vijayavenkataraman S. Computational fluid dynamics assessment of the effect of bioprinting parameters in extrusion bioprinting. Int J Bioprint 2022;8:545. doi: 10.18063/ijb.v8i2.545
- Andreas B, Filipa D, Campos D, et al. Controlling shear stress in 3D bioprinting is a key factor to balance printing resolution and stem cell integrity. Adv Healthc Mater. 2016;5:326-333. doi: 10.1002/adhm.201500677
- Rajabi M, McConnell M, Cabral J, Ali MA. Chitosan hydrogels in 3D printing for biomedical applications. Carbohydr Polym. 2021;260:117768. doi: 10.1016/j.carbpol.2021.117768
- Falcone G, Schrüfer S, Kuth S, et al. Ready-to-print alginate inks: the effect of different divalent cations on physico-chemical properties of 3D printable alginate hydrogels. Carbohydr Polym Technol Appl. 2024;7:100524. doi: 10.1016/j.carpta.2024.100524
- Piras CC, Smith DK. Multicomponent polysaccharide alginate-based bioinks. J Mater Chem B. 2020;8(36):8171-8188. doi: 10.1039/D0TB01005G
- Lee M, Kim YS, Park J, et al. A paintable and adhesive hydrogel cardiac patch with sustained release of ANGPTL4 for infarcted heart repair. Bioact Mater. 2023;31:395-407. doi: 10.1016/j.bioactmat.2023.08.020
- Hong SJ, Kim DH, Ryoo JH, et al. Influence of gelatin on adhesion, proliferation, and adipogenic differentiation of adipose tissue-derived stem cells cultured on soy protein-agarose scaffolds. Foods. 2024;13(14):2247. doi: 10.3390/foods13142247
- Li K, Wang X, Li J, Wang J, Yu W, Ge L. Bioinspired gelatin nano-film implanted into composite scaffold exhibiting both expandable adhesion and enhanced proliferation. Int J Biol Macromol. 2022;220:1570-1578. doi: 10.1016/j.ijbiomac.2022.09.080
- Nelson C, Tuladhar S, Launen L, Habib MA. 3D bio-printability of hybrid pre-crosslinked hydrogels. Int J Mol Sci. 2021;22:13481. doi: 10.3390/ijms222413481
- Choe G, Lee M, Oh S, et al. Three-dimensional bioprinting of mesenchymal stem cells using an osteoinductive bioink containing alginate and BMP-2-loaded PLGA nanoparticles for bone tissue engineering. Biomater Adv. 2022;136:212789. doi: 10.1016/j.bioadv.2022.212789
- Kumar A, Nune KC, Murr LE, Misra RDK. Biocompatibility and mechanical behaviour of three-dimensional scaffolds for biomedical devices: process–structure–property paradigm. Int Mater Rev. 2016;61:20-25. doi: 10.1080/09506608.2015.1128310
- Park KW, Truong TT, Park JH, et al. Robust and customizable spheroid culture system for regenerative medicine. Biofabrication. 2024;16:045016. doi: 10.1088/1758-5090/ad6795
- Han SS, Cho Mo, Huh KM, Kang SW. Effects of nanopatterned-surface dishes on chondrocyte growth and cell progression. RSC Adv. 2021;11:39-47. doi: 10.1039/d0ra08256b
- Han SS, Yoon HY, Yhee JY, et al. In situ cross-linkable hyaluronic acid hydrogels using copper free click chemistry for cartilage tissue engineering. Polym Chem. 2018;9:20-27. doi: 10.1039/C7PY01654A