Development of a 3D-printed polycaprolactone/ magnesium phosphate composite scaffold functionalized with novel antimicrobial peptides for enhanced bone defect regeneration
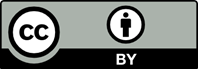
Treating large-sized infectious bone defects is currently one of the most urgent clinical challenges that need to be addressed in clinical practice. The clinical application of autologous and allogeneic bone grafts faces numerous persistent challenges that remain unresolved. Therefore, there is an urgent need to develop a bone repair scaffold capable of large-scale production, safe for in vivo use, and possessing robust bone repair and anti-infective properties. In this study, a 3D-printed bone repair scaffold was fabricated using a polycaprolactone (PCL) and magnesium phosphate (MgP) composite material. The scaffold subsequently underwent surface modification with the antimicrobial peptide Tet213 with a DOPA tail, ultimately leading to the development of a novel bone repair scaffold named DTet213@PCL/MgP. The experimental results demonstrated that the DTet213@PCL/ MgP scaffold exhibited outstanding antibacterial efficacy against Escherichia coli (E. coli) and Staphylococcus aureus (S. aureus), along with superior proliferation and osteogenesis capabilities for MC3T3-E1 preosteoblastic cells. In a rat radial defect model, the scaffold effectively induced new bone formation at the defect site, resulting in rapid bone regeneration. Furthermore, histopathological examination (HE staining) of major organs confirmed the excellent in vivo biocompatibility and safety profile of the DTet213@PCL/MgP scaffold. In the future, the DTet213@PCL/MgP scaffold represents a novel solution for the treatment of large-scale infected bone defects, capitalizing on its dual functionality in osteogenesis and infection control.

- Toosi S, Javid-Naderi MJ, Tamayol A, et al. Additively manufactured porous scaffolds by design for treatment of bone defects. Front Bioeng Biotechnol. 2024;11:1252636. doi: 10.3389/fbioe.2023.1252636
- Arrington ED, Smith WJ, Chambers HG, et al. Complications of iliac crest bone graft harvesting. Clin Orthop Relat Res. 1996;(329): 300-309. doi: 10.1097/00003086-199608000-00037
- Yuan X, Zhu W, Yang ZY, et al. Recent advances in 3D printing of smart scaffolds for bone tissue engineering and regeneration. Adv Mater. 2024;36(34):e2403641. doi: 10.1002/adma.202403641
- Cao ZM, Qin ZD, Duns GJ, et al. Repair of infected bone defects with hydrogel materials. Polymers (Basel). 2024;16(2):281. doi: 10.3390/polym16020281
- Costerton JW, Stewart PS, Greenberg EP. Bacterial biofilms: a common cause of persistent infections. Science.1999;284(5418):1318-1322. doi: 10.1126/science.284.5418.1318
- Yang C, Zhou L, Geng X, et al. New dual-function in situ bone repair scaffolds promote osteogenesis and reduce infection. J Biol Eng. 2022;16(1):23. doi: 10.1186/s13036-022-00302-y
- Wright GD. The Janus effect: the biochemical logic of antibiotic resistance. Biochemistry. 2025;64(2):301-311. doi: 10.1021/acs.biochem.4c00585
- Balasegaram M, Outterson K, Røttingen J-A. The time to address the antibiotic pipeline and access crisis is now. Lancet. 2024;404(10461):1385-1387. doi: 10.1016/S0140-6736(24)01940-8
- Bahar AA, Ren D. Antimicrobial peptides. Pharmaceuticals (Basel, Switzerland). 2013;6(12):1543-1575. doi: 10.3390/ph6121543
- Yeaman MR, Yount NY. Mechanisms of antimicrobial peptide action and resistance. Pharmacol Rev. 2003;55(1): 27-55. doi: 10.1124/pr.55.1.2
- Ibrahim DM, Sani ES, Soliman AM, et al. Bioactive and elastic nanocomposites with antimicrobial properties for bone tissue regeneration. ACS Applied Bio Materi. 2020;3(5):3313-3325. doi: 10.1021/acsabm.0c00250
- Lei B, Gao X, Zhang R, et al. In situ magnesium phosphate/ polycaprolactone 3D-printed scaffold induce bone regeneration in rabbit maxillofacial bone defect model. Mater Design. 2022;215:110477. doi: 10.1016/j.matdes.2022.110477
- Zhan J, Xu H, Zhong Y, et al. Surface modification of patterned electrospun nanofibrous films via the adhesion of DOPA-bFGF and DOPA-ponericin G1 for skin wound healing. Mater Design. 2020;188:108432. doi: 10.1016/j.matdes.2019.108432
- Blair JMA, Webber MA, Baylay AJ, et al. Molecular mechanisms of antibiotic resistance. Nat Rev Microbiol. 2015;13(1):42-51. doi: 10.1038/nrmicro3380
- Chang DH, Lee M-R, Wang N, et al. Establishing quantifiable guidelines for antimicrobial α/β-peptide design: a partial least-squares approach to improve antimicrobial activity and reduce mammalian cell toxicity. Acs Infect Dis. 2023;9(12): 2632-2651. doi: 10.1021/acsinfecdis.3c00468
- Zhang H, Jin L, Wang Q. Activities of antimicrobial peptides and the reconstruction of the natural antimicrobial peptides. Chem Life. 2011;31(2):227-232. doi: 10.13488/j.smhx.2011.02.020
- Yang C, Yu Z, Qin D, et al. Research progress in structures, mechanisms, and modification of antimicrobial peptides. Acta Microbiol Sinica. 2024;64(7):2242-2259. doi: 10.13343/j.cnki.wsxb.20230812
- Wu D, Wang L. Research progress in molecular design and optimization strategies of fish-derived antimicrobial peptides and their applications. Chin J Anim Nutr. 2023;35(12):7541- 7551. doi: 10.12418/CJAN2023.684
- Ardhaoui M, Naciri M, Mullen T, et al. Evaluation of cell behaviour on atmospheric plasma deposited siloxane and fluorosiloxane coatings. J Adhesion Sci Technol. 2010;24(5):889-903. doi: 10.1163/016942409x12598231567943
- Dziadek M, Menaszek E, Zagrajczuk B, et al. New generation poly(ε-caprolactone)/gel-derived bioactive glass composites for bone tissue engineering: part I. Material properties. Mater Sci Eng C Mater Biol Appl. 2015;56:9-21. doi: 10.1016/j.msec.2015.06.020
- Zhang Y, Xu T, Li T, et al. A three-phase strategy of bionic drug reservoir scaffold by 3D printing and layer-by-layer modification for chronic relapse management in traumatic osteomyelitis. Mater Today Bio. 2024;29:101356. doi: 10.1016/j.mtbio.2024.101356
- Zhang S, Xiao Z, Luo J, et al. Dose-dependent effects of Runx2 on bone development. J Bone Mineral Res. 2009;24(11): 1889-1904. doi: 10.1359/jbmr.090502
- Bai R, Miao MZ, Li H, et al. Increased Wnt/β-catenin signaling contributes to autophagy inhibition resulting from a dietary magnesium deficiency in injury-induced osteoarthritis. Arthritis Res Ther. 2022;24(1):165. doi: 10.1186/s13075-022-02848-0
- Xu C, Guan SQ, Hou WY, et al. Magnesium-organic framework modified biodegradable electrospun scaffolds for promoting osteogenic differentiation and bone regeneration. Eur Polym J. 2022;181:111692. doi: 10.1016/j.eurpolymj.2022.111692
- Liu L, Luo P, Wen PF, et al. The role of magnesium in the pathogenesis of osteoporosis. Front Endocrinol (Lausanne). 2024;15:1406248. doi: 10.3389/fendo.2024.1406248
- Li L, Lu H, Zhao Y, et al. Functionalized cell-free scaffolds for bone defect repair inspired by self healing of bone fractures: a review and new perspectives. Mater Sci Eng C Mater Biol Appl. 2019;98:1241-1251. doi: 10.1016/j.msec.2019.01.075
- Golafshan N, Vorndran E, Zaharievski S, et al. Tough magnesium phosphate-based 3D-printed implants induce bone regeneration in an equine defect model. Biomaterials. 2020;261:120302. doi: 10.1016/j.biomaterials.2020.120302
- Hung C-C, Chaya A, Liu K, et al. The role of magnesium ions in bone regeneration involves the canonical Wnt signaling pathway. Acta Biomater. 2019;98:246-255. doi: 10.1016/j.actbio.2019.06.001
- Khotib J, Gani MA, Budiatin AS, et al. Signaling pathway and transcriptional regulation in osteoblasts during bone healing: direct involvement of hydroxyapatite as a biomaterial. Pharmaceuticals. 2021;14(7):615. doi: 10.3390/ph14070615