Biomechanically inspired composite hydrogel bioink enhances engineered cartilage formation
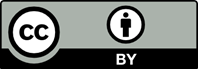
Current therapies for articular cartilage defects or degeneration, such as osteoarthritis, remain largely unsatisfactory. There are significant clinical demands for the development of more efficient approaches to enhance the repair or regeneration of articular cartilage lesions. In this study, a newly defined alginate-gelatin (A-G) composite bioink was synthesized using the carbodiimide chemistry crosslinking method. Then, the dual-crosslinking (DC) bioscaffolds containing both chemical and ionic networks were fabricated by 3D-bioprinting technique and Ca2+ treatment. Mimicking the mechanical properties of the pericellular matrix of native articular cartilage, the optimized networks formed in the DC bioscaffolds provided a desirable Young’s modulus and geometric constraints, which were superior to that of the single-crosslinking control group. Chondrocytes encapsulated in the 3D printed A-G-DC bioscaffolds exhibited above 95% viability and higher proliferation rate compared to those in the alginate-only group after 7 days of in vitro culture. Chondrogenic differentiation in vitro was improved in the A-G-DC bioscaffolds compared to that of the alginate-only group, indexed by upregulation of chondrogenic marker gene expression, including SOX9, COL2A1, COMP, and ACAN. In subcutaneous transplantation and osteochondral defect models in severe combined immunodeficiency mice, the A-G-DC bioscaffolds exhibited superior chondrogenesis and repair capacity compared to the alginate-only or no-implant control groups. This was evidenced by increased expression of SOX9 and collagen type II (Col II) and higher ICRS II scores. These findings demonstrate that the biomechanically inspired A-G composite bioink and the 3D-printed A-G-DC bioscaffolds possess excellent cell biocompatibility, satisfactory biomechanical properties, and the ability to promote chondrogenesis, making them a promising candidate for enhancing cartilage regeneration in patients with articular cartilage lesions.

- Mithoefer K, McAdams T, Williams RJ, Kreuz PC, Mandelbaum BR. Clinical efficacy of the microfracture technique for articular cartilage repair in the knee: an evidence-based systematic analysis. Am J Sports Med. 2009;37(10):2053-2063. doi: 10.1177/0363546508328414
- Dixit M, Doan T, Kirschner R, Dixit N. Significant acute kidney injury due to non-steroidal anti-inflammatory drugs: inpatient setting. Pharmaceuticals (Basel). 2010;3(4):1279-1285. doi: 10.3390/ph3041279
- Yamashita F, Sakakida K, Suzu F, Takai S. The transplantation of an autogeneic osteochondral fragment for osteochondritis dissecans of the knee. Clin Orthop Relat Res. 1985;(201):43-50. doi: 10.1097/00003086-198512000-00007
- Chung C, Burdick JA. Engineering cartilage tissue. Adv Drug Deliv Rev. 2008;60(2):243-262. doi: 10.1016/j.addr.2007.08.027
- Vinatier C, Guicheux J. Cartilage tissue engineering: from biomaterials and stem cells to osteoarthritis treatments. Ann Phys Rehabil Med. 2016;59(3):139-144. doi: 10.1016/j.rehab.2016.03.002
- Semba JA, Mieloch AA, Rybka JD. Introduction to the state-of-the-art 3D bioprinting methods, design, and applications in orthopedics. Bioprinting. 2020;18:e00070. doi: 10.1016/j.bprint.2019.e00070
- Kilian D, Ahlfeld T, Akkineni AR, Bernhardt A, Gelinsky M, Lode A. 3D bioprinting of osteochondral tissue substitutes– in vitro-chondrogenesis in multi-layered mineralized constructs. Sci Rep. 2020 ;10(1):8277. doi: 10.1038/s41598-020-65050-9
- Zhang H, Zhou Z, Zhang F, Wan C. Hydrogel-based 3D bioprinting technology for articular cartilage regenerative engineering. Gels. 2024;10(7):430. doi: 10.3390/gels10070430
- Fu Z, Naghieh S, Xu C, Wang C, Sun W, Chen X. Printability in extrusion bioprinting. Biofabrication. 2021; 13(3):1-15. doi: 10.1088/1758-5090/abe7ab
- Malekpour A, Chen X. Printability and cell viability in extrusion-based bioprinting from experimental, computational, and machine learning views. J Funct Biomater. 2022;13(2):40. doi: 10.3390/jfb13020040
- Placone JK, Engler AJ. Recent advances in extrusion-based 3D printing for biomedical applications. Adv Healthc Mater. 2018;7(8):e1701161. doi: 10.1002/adhm.201701161
- Ng WL, Shkolnikov V. Jetting-based bioprinting: process, dispense physics, and applications. Bio-Design Manuf. 2024;7(5):771-799. doi: 10.1007/s42242-024-00285-3
- Barron JA, Spargo BJ, Ringeisen BR. Biological laser printing of three dimensional cellular structures. Appl Phys A. 2004;79:1027-1030. doi: 10.1007/s00339-004-2620-3
- Heinrich MA, Liu W, Jimenez A, et al. 3D bioprinting: from benches to translational applications. Small. 2019;15(23):e1805510. doi: 10.1002/smll.201805510
- Li Y, Zhang X, Zhang X, Zhang Y, Hou D. Recent progress of the vat photopolymerization technique in tissue engineering: a brief review of mechanisms, methods, materials, and applications. Polymers (Basel). 2023; 15(19):3940. doi: 10.3390/polym15193940
- Koons GL, Diba M, Mikos AG. Materials design for bone-tissue engineering. Nat Rev Mater. 2020;5(8):584-603. doi: 10.1038/s41578-020-0204-2
- Collins MN, Ren G, Young K, Pina S, Reis RL, Oliveira JM. Scaffold fabrication technologies and structure/function properties in bone tissue engineering. Adv Funct Mater. 2021;31(21):2010609. doi: 10.1002/adfm.202010609
- Pitacco P, Sadowska JM, O’Brien FJ, Kelly DJ. 3D bioprinting of cartilaginous templates for large bone defect healing. Acta Biomater. 2023;156:61-74. doi: 10.1016/j.actbio.2022.07.037
- Awad HA, Wickham MQ, Leddy HA, Gimble JM, Guilak F. Chondrogenic differentiation of adipose-derived adult stem cells in agarose, alginate, and gelatin scaffolds. Biomaterials. 2004;25(16):3211-3222. doi: 10.1016/j.biomaterials.2003.10.045
- Schuurman W, Levett PA, Pot MW, et al. Gelatin‐methacrylamide hydrogels as potential biomaterials for fabrication of tissue‐engineered cartilage constructs. Macromol Biosci. 2013;13(5):551-561. doi: 10.1002/mabi.201200471
- Annabi N, Tamayol A, Uquillas JA, et al. 25th anniversary article: rational design and applications of hydrogels in regenerative medicine. Adv Mater. 2014;26(1):85-124. doi: 10.1002/adma.201303233
- Yang Y, Wang X, Yang F, Shen H, Wu D. A universal soaking strategy to convert composite hydrogels into extremely tough and rapidly recoverable double‐network hydrogels. Adv Mater. 2016;28(33):7178-7184. doi: 10.1002/adma.201601742
- Baei P, Daemi H, Mostafaei F, Sayahpour FA, Baharvand H, Eslaminejad MB. A tough polysaccharide-based cell-laden double-network hydrogel promotes articular cartilage tissue regeneration in rabbits. Chem Eng J. 2021;418:129277. doi: 10.1016/j.cej.2021.129277
- Hua Y, Xia H, Jia L, et al. Ultrafast, tough, and adhesive hydrogel based on hybrid photocrosslinking for articular cartilage repair in water-filled arthroscopy. Sci Adv. 2021;7(35):eabg0628. doi: 10.1126/sciadv.abg0628
- Chen F-M, Liu X. Advancing biomaterials of human origin for tissue engineering. Prog Polym Sci. 2016;53:86-168. doi: 10.1016/j.progpolymsci.2015.02.004
- D’Souza SE, Ginsberg MH, Plow EF. Arginyl-glycyl-aspartic acid (RGD): a cell adhesion motif. Trends Biochem Sci. 1991;16:246-250. doi: 10.1016/0968-0004(91)90096-e
- Rowley JA, Madlambayan G, Mooney DJ. Alginate hydrogels as synthetic extracellular matrix materials. Biomaterials. 1999;20(1):45-53. doi: 10.1016/s0142-9612(98)00107-0
- Lozano R, Stevens L, Thompson BC, et al. 3D printing of layered brain-like structures using peptide modified gellan gum substrates. Biomaterials. 2015;67:264-273. doi: 10.1016/j.biomaterials.2015.07.022
- Jahanbakhsh A, Nourbakhsh MS, Bonakdar S, Shokrgozar MA, Haghighipour N. Evaluation of alginate modification effect on cell-matrix interaction, mechanotransduction and chondrogenesis of encapsulated MSCs. Cell Tissue Res. 2020;381(2):255-272. doi: 10.1007/s00441-020-03216-7
- Zatorski JM, Montalbine AN, Ortiz-Cárdenas JE, Pompano RR. Quantification of fractional and absolute functionalization of gelatin hydrogels by optimized ninhydrin assay and 1H NMR. Anal Bioanal Chem. 2020;412(24):6211-6220. doi: 10.1007/s00216-020-02792-5
- Smith DL, Lemieux EN, Barua S. Correction in bicinchoninic acid (BCA) absorbance assay to analyze protein concentration. Nano Life. 2018;8(03): 1850005. doi: 10.1142/S1793984418500058
- Mintz BR, Cooper Jr JA. Hybrid hyaluronic acid hydrogel/ poly (ɛ‐caprolactone) scaffold provides mechanically favorable platform for cartilage tissue engineering studies. J Biomed Mater Res A. 2014;102(9):2918-2926. doi: 10.1002/jbm.a.34957.
- Chen M, Lichtler AC, Sheu TJ, et al. Generation of a transgenic mouse model with chondrocyte‐specific and tamoxifen‐inducible expression of Cre recombinase. Genesis. 2007;45(1):44-50. doi: 10.1002/dvg.20261
- Zhang F, He Q, Tsang WP, Garvey WT, Chan WY, Wan C. Insulin exerts direct, IGF-1 independent actions in growth plate chondrocytes. Bone Res. 2014;2(1):1-10. doi: 10.1038/boneres.2014.12
- Grosskopf AK, Correa S, Baillet J, et al. Consistent tumorigenesis with self-assembled hydrogels enables high-powered murine cancer studies. Commun Biol. 2021;4(1):985. doi: 10.1038/s42003-021-02500-8
- Wang P, Zhang F, He Q, et al. Flavonoid compound icariin activates hypoxia inducible factor-1α in chondrocytes and promotes articular cartilage repair. PLOS ONE. 2016;11(2):e0148372. doi: 10.1371/journal.pone.0148372
- Mainil-Varlet P, Van Damme B, Nesic D, Knutsen G, Kandel R, Roberts S. A new histology scoring system for the assessment of the quality of human cartilage repair: ICRS II. Am J Sports Med. 2010;38(5):880-890. doi: 10.1177/0363546509359068
- Grigore A, Sarker B, Fabry B, Boccaccini AR, Detsch R. Behavior of encapsulated MG-63 cells in RGD and gelatine-modified alginate hydrogels. Tissue Eng Part A. 2014; 20(15-16):2140-2150. doi: 10.1089/ten.tea.2013.0416
- Ye J, Xiong J, Sun R. The fluorescence property of Schiff ’s bases of carboxymethyl cellulose. Carbohydr Polym. 2012;88(4):1420-1424. doi: 10.1016/j.carbpol.2012.02.030
- Kolesky DB, Truby RL, Gladman AS, Busbee TA, Homan KA, Lewis JA. 3D bioprinting of vascularized, heterogeneous cell‐laden tissue constructs. Adv Mater. 2014;26(19):3124-3130. doi: 10.1002/adma.201305506
- Duan B, Hockaday LA, Kang KH, Butcher JT. 3D bioprinting of heterogeneous aortic valve conduits with alginate/gelatin hydrogels. J Biomed Mater Res A. 2013;101(5):1255-1264.doi: 10.1002/jbm.a.34420.
- Jiang S, Lyu C, Zhao P, et al. Cryoprotectant enables structural control of porous scaffolds for exploration of cellular mechano-responsiveness in 3D. Nat Commun. 2019;10(1):3491. doi: 10.1038/s41467-019-11397-1
- Han CD. Rheology in Polymer Processing. Academic Press; 1976.
- de Melo BA, Jodat YA, Mehrotra S, et al. 3D printed cartilage‐like tissue constructs with spatially controlled mechanical properties. Adv Funct Mater. 2019;29(51):1906330. doi: 10.1002/adfm.201906330
- Xu W, Zhu J, Hu J, Xiao L. Engineering the biomechanical microenvironment of chondrocytes towards articular cartilage tissue engineering. Life Sci. 2022;309:121043. doi: 10.1016/j.lfs.2022.121043
- Engler AJ, Sen S, Sweeney HL, Discher DE. Matrix elasticity directs stem cell lineage specification. Cell. 2006;126(4):677-689. doi: 10.1016/j.cell.2006.06.044
- Trappmann B, Chen CS. How cells sense extracellular matrix stiffness: a material’s perspective. Curr Opin Biotechnol. 2013;24(5):948-953. doi: 10.1016/j.copbio.2013.03.020
- Michon C, Cuvelier G, Launay B. Concentration dependence of the critical viscoelastic properties of gelatin at the gel point. Rheologica Acta. 1993;32(1):94-103. doi: 10.1007/BF00396681
- Guilak F, Alexopoulos LG, Haider MA, Ting-Beall HP, Setton LA. Zonal uniformity in mechanical properties of the chondrocyte pericellular matrix: micropipette aspiration of canine chondrons isolated by cartilage homogenization. Ann Biomed Eng. 2005;33(10):1312-1318. doi: 10.1007/s10439-005-4479-7
- Cox TR, Erler JT. Remodeling and homeostasis of the extracellular matrix: implications for fibrotic diseases and cancer. Dis Model Mech. 2011;4(2):165-178. doi: 10.1242/dmm.004077
- Cai Z, Tang Y, Wei Y, Wang P, Zhang H. Double–network hydrogel based on exopolysaccharides as a biomimetic extracellular matrix to augment articular cartilage regeneration. Acta Biomater. 2022;152:124-143. doi: 10.1016/j.actbio.2022.08.062
- Jung Y, Park MS, Lee JW, Kim YH, Kim S-H, Kim SH. Cartilage regeneration with highly-elastic three-dimensional scaffolds prepared from biodegradable poly (l-lactide-co-ɛ-caprolactone). Biomaterials. 2008;29(35):4630-4636. doi: 10.1016/j.biomaterials.2008.08.031
- Nava MM, Draghi L, Giordano C, Pietrabissa R. The effect of scaffold pore size in cartilage tissue engineering. J Appl Biomater Funct Mater. 2016;14(3):e223-9. doi: 10.5301/jabfm.5000302
- Zhang Q, Lu H, Kawazoe N, Chen G. Pore size effect of collagen scaffolds on cartilage regeneration. Acta Biomater. 2014;10(5):2005-2013. doi: 10.1016/j.actbio.2013.12.042
- Elisseeff J. Injectable cartilage tissue engineering. Expert Opin Biol Ther. 2004;4(12):1849-1859. doi: 10.1517/14712598.4.12.1849
- Vinatier C, Mrugala D, Jorgensen C, Guicheux J, Noël D. Cartilage engineering: a crucial combination of cells, biomaterials and biofactors. Trends Biotechnol. 2009;27(5):307-314. doi: 10.1016/j.tibtech.2009.02.005
- Fisch KM, Gamini R, Alvarez-Garcia O, et al. Identification of transcription factors responsible for dysregulated networks in human osteoarthritis cartilage by global gene expression analysis. Osteoarthritis Cartilage. 2018;26(11):1531-1538. doi: 10.1016/j.joca.2018.07.012
- Alexopoulos LG, Youn I, Bonaldo P, Guilak F. Developmental and osteoarthritic changes in Col6a1‐knockout mice: biomechanics of type VI collagen in the cartilage pericellular matrix. Arthritis Rheum. 2009;60(3):771-779. doi: 10.1002/art.24293
- Wilusz RE, Zauscher S, Guilak F. Micromechanical mapping of early osteoarthritic changes in the pericellular matrix of human articular cartilage. Osteoarthritis Cartilage. 2013;21(12):1895-1903. doi: 10.1016/j.joca.2013.08.026
- Guilak F, Nims RJ, Dicks A, Wu C-L, Meulenbelt I. Osteoarthritis as a disease of the cartilage pericellular matrix. Matrix Biol. 2018;71-72:40-50. doi: 10.1016/j.matbio.2018.05.008
- Sassi N, Laadhar L, Allouche M, et al. Wnt signaling is involved in human articular chondrocyte de-differentiation in vitro. Biotech Histochem. 2014;89(1):29-40. doi: 10.3109/10520295.2013.811285