3D printing of microstructured piezoelectric and bioactive PCL-composite scaffolds for bone regeneration
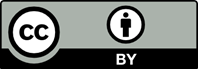
Despite the self-healing ability of bone tissue, the treatment of critical-size defects and the limited regenerative process in osteoporosis or avascular necrosis demand special medical attention. One of the goals of bone tissue engineering is to develop new patient-specific solutions for bone repair. In this study, we focus on the fabrication of degradable subchondral bone scaffolds made of highly loaded polycaprolactone (PCL) using direct ink writing. Barium titanate (BTO) and the bioactive glass 45S5 (BG) were used as filler materials to modify the material’s piezoelectric, mechanical, and bioresponsive properties. The mechanical properties of our composites are in the range of spongy bone, and a compressive modulus of around 181 MPa was achieved for PCL/BTO and 98.3 MPa for PCL/BTO/BG scaffolds. The use of 40 vol.% BTO in combination with PCL showed piezoelectric properties in the range of bone tissue with a d33 of 0.75 pC/N. While adding BG decreases the piezoelectric properties, it increases the bioactivity and the osteogenic response of primary human osteoblasts. In summary, these novel material compositions provide a promising approach for developing multiphasic scaffolds for bone tissue engineering.

- Donate R, Paz R, Moriche R, Sayagués MJ, Alemán- Domínguez ME, Monzón M. An overview of polymeric composite scaffolds with piezoelectric properties for improved bone regeneration. Mater Des. 2023;231:112085. doi: 10.1016/j.matdes.2023.112085
- Koons GL, Diba M, Mikos AG. Materials design for bone-tissue engineering. Nat Rev Mater. 2020;5:584-603. doi: 10.1038/s41578-020-0204-2
- Ahn AC, Grodzinsky AJ. Relevance of collagen piezoelectricity to “Wolff ’s Law”: a critical review. Med Eng Phys. 2009:;31:733-741. doi: 10.1016/j.medengphy.2009.02.006
- Khare D, Basu B, Dubey AK. Electrical stimulation and piezoelectric biomaterials for bone tissue engineering applications. Biomaterials. 2020;258:120280. doi: 10.1016/j.biomaterials.2020.120280
- Rajabi AH, Jaffe M, Arinzeh TL. Piezoelectric materials for tissue regeneration: a review. Acta Biomater. 2015;24:12-23. doi: 10.1016/j.actbio.2015.07.010
- Li S, Shan Y, Chen J, et al. 3D printing and biomedical applications of piezoelectric composites: a critical review. Adv Mater Technol. 2024. doi: 10.1002/admt.202401160
- Sundelacruz S, Li C, Choi YJ, Levin M, Kaplan DL. Bioelectric modulation of wound healing in a 3D in vitro model of tissue-engineered bone. Biomaterials. 2013;34:6695-6705. doi: 10.1016/j.biomaterials.2013.05.040
- Tandon B, Blaker JJ, Cartmell SH. Piezoelectric materials as stimulatory biomedical materials and scaffolds for bone repair. Acta Biomater. 2018;73:1-20. doi: 10.1016/j.actbio.2018.04.026
- Kapat K, Shubhra QTH, Zhou M, Leeuwenburgh S. Piezoelectric nano‐biomaterials for biomedicine and tissue regeneration. Adv Funct Mater. 2020;30. doi: 10.1002/adfm.201909045
- Acosta M, Novak N, Rojas V, et al. BaTiO3-based piezoelectrics: fundamentals, current status, and perspectives. Appl Phys Rev. 2017;4. doi: 10.1063/1.4990046
- Mancuso E, Shah L, Jindal S, et al. Additively manufactured BaTiO3 composite scaffolds: a novel strategy for load bearing bone tissue engineering applications. Mater Sci Eng C Mater Biol Appl. 2021;126:112192. doi: 10.1016/j.msec.2021.112192
- Liu W, Yang D, Wei X, et al. Fabrication of piezoelectric porous BaTiO3 scaffold to repair large segmental bone defect in sheep. J Biomater Appl. 2020;35:544-552. doi: 10.1177/0885328220942906
- Polley C, Distler T, Detsch R, et al. 3D printing of piezoelectric barium titanate-hydroxyapatite scaffolds with interconnected porosity for bone tissue engineering. Materials (Basel, Switzerland). 2020;13:1773. doi: 10.3390/ma13071773
- Polley C, Distler T, Scheufler C, et al. 3D printing of piezoelectric and bioactive barium titanate-bioactive glass scaffolds for bone tissue engineering. Mater Today Bio. 2023;21:100719. doi: 10.1016/j.mtbio.2023.100719
- Hench LL. Chronology of bioactive glass development and clinical applications. NJGC. 2013;03:67-73. doi: 10.4236/njgc.2013.32011
- Filho OP, La Torre GP, Hench LL. Effect of crystallization on apatite-layer formation of bioactive glass 45S5. J Biomed Mater Res. 1996;30:509-514. doi: 10.1002/(SICI)1097-4636(199604)30:4<509::AID-JBM9>3.0.CO;2-T
- Hench LL, Splinter RJ, Allen WC, Greenlee TK. Bonding mechanisms at the interface of ceramic prosthetic materials. J Biomed Mater Res. 1971;5:117-141. doi: 10.1002/jbm.820050611
- Soni R, Kumar NV, Chameettachal S, Pati F, Narayan Rath S. Synthesis and optimization of PCL-bioactive glass composite scaffold for bone tissue engineering. Mater Today Proc. 2019;15:294-299. doi: 10.1016/j.matpr.2019.05.008
- Petretta M, Gambardella A, Boi M, et al. Composite scaffolds for bone tissue regeneration based on PCL and Mg-containing bioactive glasses. Biology. 2021;10:398. doi: 10.3390/biology10050398
- Bi L, Li D, Liu J, et al. Fabrication and characterization of a biphasic scaffold for osteochondral tissue engineering. Mater Lett. 2011;65:2079-2082. doi: 10.1016/j.matlet.2011.04.013
- Mukundan LM, Nirmal RS, Nair PD. Growth and regeneration of osteochondral cells in bioactive niche: a promising approach for osteochondral tissue repair. ACS Appl Bio Mater. 2022;5:2676-2688. doi: 10.1021/acsabm.2c00125
- Fathi A, Kermani F, Behnamghader A, et al. Three-dimensionally printed polycaprolactone/multicomponent bioactive glass scaffolds for potential application in bone tissue engineering. Biomed Glass. 2020;6:57-69. doi: 10.1515/bglass-2020-0006
- Rotan M, Zhuk M, Glaum J. Activation of ferroelectric implant ceramics by corona discharge poling. J European Ceram Soc. 2020;40:5402-5409. doi: 10.1016/j.jeurceramsoc.2020.06.058
- Riaz A, Polley C, Lund H, Springer A, Seitz H. A novel approach to fabricate load-bearing Ti6Al4V-Barium titanate piezoelectric bone scaffolds by coupling electron beam melting and field-assisted sintering. Mater Des. 2023;225:111428. doi: 10.1016/j.matdes.2022.111428
- Polley C, Riaz A, Lund H, Boccaccini AR, Seitz H. Field-assisted sintering of barium titanate and 45S5 bioactive glass for biomedical applications. Ceramics Int. 2024;50(19): 37314-37321. doi: 10.1016/j.ceramint.2024.07.107
- Kokubo T, Takadama H. How useful is SBF in predicting in vivo bone bioactivity?. Biomaterials. 2006;27:2907-2915. doi: 10.1016/j.biomaterials.2006.01.017
- Jonitz-Heincke A, Sellin ML, Seyfarth A, et al. Analysis of cellular activity short-term exposure to cobalt and chromium ions in mature human osteoblasts. Materials (Basel, Switzerland). 2019;12:2771. doi: 10.3390/ma12172771
- Peng Y, Zhuang Y, Liu Y, et al. Bioinspired gradient scaffolds for osteochondral tissue engineering. Exploration (Beijing, China). 2023;3:20210043. doi: 10.1002/EXP.20210043
- Ronca D, Langella F, Chierchia M, et al. Bone tissue engineering: 3D PCL-based nanocomposite scaffolds with tailored properties. Procedia CIRP. 2016;49:51-54. doi: 10.1016/j.procir.2015.07.028
- Rouwkema J, Rivron NC, van Blitterswijk CA. Vascularization in tissue engineering. Trends Biotechnol. 2008;26:434-441. doi: 10.1016/j.tibtech.2008.04.009
- Abbasi N, Hamlet S, Love RM, Nguyen NT. Porous scaffolds for bone regeneration. J Sci Adv Mater Dev. 2020;5:1-9. doi: 10.1016/j.jsamd.2020.01.007
- Sandino C, Lacroix D. A dynamical study of the mechanical stimuli and tissue differentiation within a CaP scaffold based on micro-CT finite element models. Biomech Model Mechanobiol. 2011;10:565-576. doi: 10.1007/s10237-010-0256-0
- Baier RV, Contreras Raggio JI, Giovanetti CM, et al. Shape fidelity, mechanical and biological performance of 3D printed polycaprolactone-bioactive glass composite scaffolds. Biomater Adv. 2022;134:112540. doi: 10.1016/j.msec.2021.112540
- Tian L, Zhang Z, Tian B, Zhang X, Wang N. Study on antibacterial properties and cytocompatibility of EPL coated 3D printed PCL/HA composite scaffolds. RSC Adv. 2020;10:4805-4816. doi: 10.1039/C9RA10275B
- Olate-Moya F, Arens L, Wilhelm M, Mateos-Timoneda MA, Engel E, Palza H. Chondroinductive alginate-based hydrogels having graphene oxide for 3D printed scaffold fabrication. ACS Appl Mater Interfaces. 2020;12:4343-4357. doi: 10.1021/acsami.9b22062
- Fabbri P, Cannillo V, Sola A, Dorigato A, Chiellini F. Highly porous polycaprolactone-45S5 Bioglass® scaffolds for bone tissue engineering. Comp Sci Technol. 2010;70:1869-1878. doi: 10.1016/j.compscitech.2010.05.029
- Pierantozzi D, Scalzone A, Jindal S, et al. 3D printed Sr-containing composite scaffolds: effect of structural design and material formulation towards new strategies for bone tissue engineering. Comp Sci Technol. 2020;191:108069. doi: 10.1016/j.compscitech.2020.108069
- Gómez-Lizárraga KK, Flores-Morales C, Del Prado-Audelo ML, Álvarez-Pérez MA, Piña-Barba MC, Escobedo C. Polycaprolactone- and polycaprolactone/ceramic-based 3D-bioplotted porous scaffolds for bone regeneration: a comparative study. Mater Sci Eng C Mater Biol Appl. 2017;79:326-335. doi: 10.1016/j.msec.2017.05.003
- Ródenas-Rochina J, Ribelles JLG, Lebourg M. Comparative study of PCL-HAp and PCL-bioglass composite scaffolds for bone tissue engineering. J Mater Sci Mater Med. 2013;24:1293-1308. doi: 10.1007/s10856-013-4878-5
- Seo C, Zhang C, Liu H. A review on the 3D printing of composite scaffolds for bone tissue engineering. In: Musculoskeletal Tissue Engineering. Elsevier. 2022:201-241. doi: 10.1016/B978-0-12-823893-6.00001-2
- Heng BC, Bai Y, Li X, et al. The bioelectrical properties of bone tissue. Animal Model Exp Med. 2023;6:120-130. doi: 10.1002/ame2.12300
- Liu J, Gu H, Liu Q, Ren L, Li G. An intelligent material for tissue reconstruction: the piezoelectric property of polycaprolactone/barium titanate composites. Mater Lett. 2019;236:686-689. doi: 10.1016/j.matlet.2018.11.036
- Köllner D, Simon S, Niedermeyer S, et al. Relation between structure, mechanical and piezoelectric properties in cellular ceramic auxetic and honeycomb structures. Adv Eng Mater. 2023;25. doi: 10.1002/adem.202201387
- Liu J, He X, Wang F, Zhou X, Li G. Dielectric and mechanical properties of polycaprolactone/nano-barium titanate piezoelectric composites. Plast Rubber Compos. 2021;50:299-306. doi: 10.1080/14658011.2021.1893517
- Sikder P, Nagaraju P, Naganaboyina HPS. 3D-printed piezoelectric porous bioactive scaffolds and clinical ultrasonic stimulation can help in enhanced bone regeneration. Bioengineering (Basel, Switzerland). 2022;9:679. doi: 10.3390/bioengineering9110679
- Ranella A, Barberoglou M, Bakogianni S, Fotakis C, Stratakis E. Tuning cell adhesion by controlling the roughness and wettability of 3D micro/nano silicon structures. Acta Biomater. 2010;6:2711-2720. doi: 10.1016/j.actbio.2010.01.016
- Fu SZ, Wang XH, Guo G, et al. Preparation and properties of nano-hydroxyapatite/PCL-PEG-PCL composite membranes for tissue engineering applications. J Biomed Mater Res B Appl Biomater. 2011;97:74-83. doi: 10.1002/jbm.b.31788
- Ji L, Wang W, Jin D, Zhou S, Song X. In vitro bioactivity and mechanical properties of bioactive glass nanoparticles/ polycaprolactone composites. Mater Sci Eng C Mater Biol Appl. 2015;46:1-9. doi: 10.1016/j.msec.2014.09.041
- Kim Y, Lim JY, Yang GH, Seo JH, Ryu HS, Kim G. 3D-printed PCL/bioglass (BGS-7) composite scaffolds with high toughness and cell-responses for bone tissue regeneration. J Indus Eng Chem. 2019;79:163-171. doi: 10.1016/j.jiec.2019.06.027
- Li X, Shi J, Dong X, Zhang L, Zeng H. A mesoporous bioactive glass/polycaprolactone composite scaffold and its bioactivity behavior. J Biomed Mater Res A. 2008;84:84-91. doi: 10.1002/jbm.a.31371
- Paxton NC, Ho SWK, Tuten BT, Lipton-Duffin J, Woodruff MA. Degradation of melt electrowritten PCL scaffolds following melt processing and plasma surface treatment. Macromol Rapid Commun. 2021;42:e2100433. doi: 10.1002/marc.202100433
- Yeo A, Wong WJ, Teoh SH. Surface modification of PCL-TCP scaffolds in rabbit calvaria defects: evaluation of scaffold degradation profile, biomechanical properties and bone healing patterns. J Biomed Mater Res A. 2010;93:1358-1367. doi: 10.1002/jbm.a.32633
- Poh PSP, Hutmacher DW, Stevens MM, Woodruff MA. Fabrication and in vitro characterization of bioactive glass composite scaffolds for bone regeneration. Biofabrication. 2013;5:45005. doi: 10.1088/1758-5082/5/4/045005
- Zheng T, Yu Y, Pang Y, et al. Improving bone regeneration with composites consisting of piezoelectric poly(l-lactide) and piezoelectric calcium/manganese co-doped barium titanate nanofibers. Comp B Eng. 2022;234:109734. doi: 10.1016/j.compositesb.2022.109734
- Sims NA. Influences of the IL-6 cytokine family on bone structure and function. Cytokine. 2021;146:155655. doi: 10.1016/j.cyto.2021.155655
- Nalesso PRL, Vedovatto M, Gregório JES, et al. Early in vivo osteogenic and inflammatory response of 3D printed polycaprolactone/carbon nanotube/hydroxyapatite/ tricalcium phosphate composite scaffolds. Polymers. 2023;15:2952. doi: 10.3390/polym15132952
- Hayami T, Kapila YL, Kapila S. MMP-1 (collagenase-1) and MMP-13 (collagenase-3) differentially regulate markers of osteoblastic differentiation in osteogenic cells. Matrix Biol. 2008;27:682-692. doi: 10.1016/j.matbio.2008.07.005
- Rozila I, Azari P, Munirah S, Safwani WKZW, Pingguan- Murphy B, Chua KH. Polycaprolactone-based scaffolds facilitates osteogenic differentiation of human adipose-derived stem cells in a co-culture system. Polymers. 2021;13:597. doi: 10.3390/polym13040597
- Antunovic F, Tolosa F, Klein C, Ocaranza R. Polycaprolactone-based scaffolds for guided tissue regeneration in periodontal therapy: a systematic review. J Appl Biomater Funct Mater. 2023;21:22808000231211416. doi: 10.1177/22808000231211416
- Xu X, Xiao L, Xu Y, et al. Vascularized bone regeneration accelerated by 3D-printed nanosilicate-functionalized polycaprolactone scaffold. Regen Biomater. 2021;8:rbab061. doi: 10.1093/rb/rbab061
- Haxaire C, Haÿ E, Geoffroy V. Runx2 controls bone resorption through the down-regulation of the Wnt pathway in osteoblasts. Am J Pathol. 2016;186:1598-1609. doi: 10.1016/j.ajpath.2016.01.016
- Amarasekara DS, Kim S, Rho J. Regulation of osteoblast differentiation by cytokine networks. Int J Mol Sci. 2021;22:2851. doi: 10.3390/ijms22062851